INTRODUCTION
MATERIALS AND METHODS
Search strategy
Inclusion/exclusion criteria
Assessment of risk of bias
RESULTS
Table 1
Summary of the studies included in this systematic review
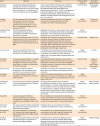
Study | Objective | Conclusion | Primary testing method | Secondary testing method |
---|---|---|---|---|
Osternack et al. [21] | This study evaluated the hardness and shrinkage of a precooled or preheated hybrid composite resin cured by a QTH light and LED curing units. The temperature on the tip of the devices was also investigated. | It was concluded that the hardness was not affected by precooling or preheating. However, polymerization shrinkage was slightly affected by different pre-polymerization temperatures. The QTH-curing generated greater shrinkage than LED-curing only when the composite was preheated. Different temperatures did not affect the composite hardness and shrinkage when cured by a LED curing unit. | Knoop hardness | Shrinkage gap formation |
D'amario et al. [22] | This study assessed the flexural strength, flexural elastic modulus and Vickers microhardness of 3 resin composites prepared at room temperature or cured after 1 or repeated preheating cycles to a temperature of 39°C. | The tested preheating procedure did not negatively influence the mechanical properties of the resin composites even when extensively repeated. | Vickers microhardness | Three-point bending test |
Jafarzadeh Kashi et al. [23] | This study evaluated the effect of 3 preheating temperatures on the microhardness of 3 different nanohybrid resin-based composites. | Regardless of the resin composite material used, surface hardness was considerably improved by increasing the temperature. The microhardness values were influenced significantly by the resin-based composite brand. | Vickers microhardness | |
Muñoz et al. [16] | This study evaluated the depth of cure and surface hardness of 2 resin composites when subjected to 3 preheating temperatures, 3 polymerization times and 2 types of curing lights. | Preheating resin composites increased the monomer conversion rate and increased the depth of cure and hardness of the tested composites. LEDs produced statistically significantly better results that the halogen curing light. Shorter polymerization times with a preheated resin can produce similar hardness values as a room-temperature resin with longer curing times. | Knoop hardness | Depth of cure |
Lucey et al. [8] | This study evaluated the effect of pre-heating resin composite on pre-cured viscosity and post-cured surface hardness. | Pre-heating a resin composite reduces its pre-cured viscosity and enhances its subsequent surface hardness. These effects may translate into easier placement, together with an increased degree of polymerization and depth of cure. | Vickers microhardness | Viscosity |
Theobaldo et al. [24] | This study evaluated the effect of composite preheating and polymerization mode on the degree of conversion, microhardness, plasticization, and depth of polymerization of a bulk fill composite. | Composite preheating increased the polymerization degree of 4-mm-increment bulk fill, but it led to a higher plasticization compared to the conventional flowable composite evaluated. Microhardness was not affected by temperature or curing mode. | Knoop hardness | Degree of conversion |
Depth of polymerization | ||||
Plasticization | ||||
Ayub et al. [25] | This study evaluated the effect of temperature on the microhardness and viscosity of 4 resin composite materials. | The effects of preheating resin composites may allow easier placement of restorations and greater monomer conversion. | Knoop hardness | Viscosity |
Caneppele et al. [26] | This study evaluated the effects of the preheating or precooling resin composite on surface hardness. | It was concluded that preheating the resin composite significantly increased its microhardness, and a light-cure time of 40 sec improved top microhardness for the use of resins at 24°C or 5°C. | Vickers microhardness | |
Mohammadi et al. [27] | This study evaluated the effect of preheating on the mechanical properties of 2 different classes of composites. | Preheating silorane enhanced the composite's microhardness and elastic modulus, but did not affect its flexural strength. In contrast, preheating Z250 increased its microhardness, but did not change its flexural strength or elastic modulus. In addition, the Z250 composite showed higher microhardness and flexural strength than silorane, but the elastic modulus values with preheating were similar. | Vickers microhardness | Flexural strength |
Elastic modulus | ||||
Awliya [28] | This study evaluated the effect of different temperatures on the efficacy of polymerization during the insertion of composite resin using different light-curing units. | The use of prewarmed composite resins might help to improve polymerization of composite resin, especially at the deeper areas of a restoration, which could result in an increase in the expected life of a composite restoration. | Vickers microhardness | |
Theodoridis et al. [29] | This study evaluated the effect of preheating and shade on the surface microhardness of silorane-based composites. | Preheating, shade, and composition of the tested composite resins affected their surface microhardness. | Vickers microhardness | |
Dionysopoulos et al. [30] | This study evaluated the microhardness of 2 composite resins when subjected to 3 different temperatures and 3 different light-curing times. | The temperature of composites affected their surface microhardness. Also, light-curing time influenced the microhardness values of the composites tested. | Vickers microhardness | |
Tantbirojn et al. [19] | This study evaluated the effect of composite preheating and light-curing duration on hardness and postgel shrinkage. | Preheating of the composites only slightly increased hardness values and did not negatively affect postgel shrinkage. Reducing the light-curing durations, however, significantly reduced the hardness at both the top (0 mm) and bottom (2 mm) surfaces. | Vickers microhardness | Postgel shrinkage |
Table 2
Bibliographic data and materials used in the included studies
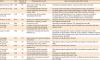
Study | Year | Total No. of specimens | Preheating device used | Type of composite used (brand name) |
---|---|---|---|---|
Osternack et al. [21] | 2013 | 120 | Water bath (TE 054 Mag, Tecnal, Piracicaba, Brazil) | Nanohybrid composite (Charisma, Heraeus-Kulzer, Hanau, Germany) |
D'amario et al. [22] | 2015 | 180 | Commercially-available composite warmer (ENA Heat, Micerium SpA, Avegno, Italy) | Enamel Plus HFO microhybrid composite (Micerium SpA); microhybrid composite (Opallis, FGM Produtos Odontologicos, Joinville, Brazil); nanoceramic composite (Ceram X Duo, Dentsply DeTrey GmbH, Konstanz, Germany) |
Jafarzadeh Kashi et al. [23] | 2015 | 90 | Laboratory oven at the specified temperatures for 30 min | Microhybrid composite (Grandio, Voco, Cuxhaven, Germany); microhybrid composite (Simile, Jeneric Pentron, Wallingford, CT, USA); nanohybrid composite (Tetric N-Ceram, Ivoclar Vivadent, Schaan, Liechtenstein) |
Muñoz et al. [16] | 2008 | 180 | Calset Unit 21 composite warming unit (AdDent, Inc., Danbury, CT, USA) | Microhybrid (Esthet-X, Dentsply Caulk, Milford, DE, USA); microhybrid composite (TPH Spectra, Dentsply Caulk) |
Lucey et al. [8] | 2009 | 30 | Dry oven for 15 min | Microhybrid composite (Spectrum TPH, Dentsply DeTrey GmbH) |
Theobaldo et al. [24] | 2017 | 40 | Incubator (model 502, Fanem Ltda, Guarulhos, Brazil) with preheating temperature (23°C and 54°C) for 1 hr | Light-cured low-stress posterior bulk fill flowable base composite (Surefil SDR, Dentsply Caulk) |
Ayub et al. [25] | 2014 | 80 | Calset Unit 3 composite warming unit (AdDent, Inc.) | Microhybrid composite (Vit-l-escence, Ultradent, Salt Lake City, UT, USA); nanohybrid composite (Tetric Ceram HB, Ivoclar Vivadent); nanofilled composite (Filtek Supreme Ultra, 3M ESPE, St Paul, MN, USA); Filtek LS Low Shrink Posterior Restorative System (3M ESPE) |
Caneppele et al. [26] | 2011 | 60 | Water bath set to a specific preheating temperature | Microhybrid composite (Z250, 3M ESPE) |
Mohammadi et al. [27] | 2016 | 102 | Water bath set to a specific preheating temperature (25°C, 37°C, or 68°C) | Filtek silorane-based composite (3M ESPE); microhybrid composite (Z250, 3M ESPE) |
Awliya [28] | 2007 | 45 | Calset Unit 21 composite warming unit (AdDent, Inc.) | Microhybrid composite (Z250, 3M ESPE) |
Theodoridis et al. [29] | 2017 | 60 | Commercially-available composite warmer (ENA Heat, Micerium SpA) | Filtek silorane-based composite (3M ESPE); microhybrid composite (Z250, 3M ESPE) |
Dionysopoulos et al. [30] | 2015 | 90 | Commercially-available composite warmer (ENA Heat, Micerium SpA) | Microhybrid composite (Z250, 3M ESPE), |
Nanohybrid composite (GrandioSO, Voco) | ||||
Tantbirojn et al. [19] | 2011 | 40 | Calset Unit 21 composite warming unit (AdDent, Inc.) | Microhybrid composite (Z250, 3M ESPE); nanofilled composite (Filtek Supreme Plus, 3M ESPE) |
Table 3
Criteria used for quality assessment and determination of the risk of bias
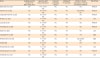
Study | Verifying spectral irradiance of light-curing units | Specimen with similar dimension | Universal preheating device | Blinding of the examiner | Vickers or Knoop indentation on the top and bottom surfaces | Risk of bias |
---|---|---|---|---|---|---|
Osternack et al. [21] | Yes | 7-12 × 2 mm | No | No | Yes | Medium |
Yes | ||||||
D'amario et al. [22] | Yes | 10 × 2 mm | Yes | No | Top surface | Medium |
Yes | No | |||||
Jafarzadeh Kashi et al. [23] | Yes | 10 × 2 mm | No | No | Yes | Medium |
Yes | ||||||
Muñoz et al. [16] | Yes | 4 × 6 mm | Yes | No | Yes | Low |
Yes | ||||||
Lucey et al. [8] | Yes | 8 × 1.5 mm | No | No | Yes | Medium |
Yes | ||||||
Theobaldo et al. [24] | Yes | 5 × 4 mm | No | No | Yes | Medium |
Yes | ||||||
Ayub et al. [25] | Yes | 5 × 2 mm | Yes | No | Yes | Low |
Yes | ||||||
Caneppele et al. [26] | No | 5 × 3 mm | No | No | Yes | High |
Yes | ||||||
Mohammadi et al. [27] | No | 4 × 2 mm | No | No | Top surface | High |
Yes | No | |||||
Awliya [28] | Yes | 8 × 2 mm | Yes | No | Yes | Low |
Yes | ||||||
Theodoridis et al. [29] | Yes | 4 × 2 mm | Yes | No | Yes | Low |
Yes | ||||||
Dionysopoulos et al. [30] | No | 6 × 2 mm | Yes | No | Yes | Medium |
Yes | ||||||
Tantbirojn et al. [19] | Yes | 4.7 × 2 mm | Yes | No | Yes | Low |
Yes |
Table 4
Microhardness of different resin composites for the top and bottom surfaces of specimens used in the included studies
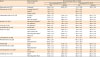
Study | Resin composite brand name | Microhardness (KHN or VHN) | |||
---|---|---|---|---|---|
Non-preheated (21°C–25°C) | Preheated (55°C–60°C) | ||||
Top | Bottom | Top | Bottom | ||
Osternack et al. [21] | Charisma* | 29.6 ± 2.0 | 29.9 ± 2.7 | 31.1 ± 1.85 | 29.1 ± 3.6 |
D'amario et al. [22] | Enamel Plus HFO* | 78.2 ± 5.8 | - | 72.5 ± 8.6 | - |
Opallis* | 64.1 ± 2.2 | - | 66.4 ± 5.2 | - | |
Ceram X Duo* | 70.1 ± 4.8 | - | 72.5 ± 5.5 | - | |
Jafarzadeh-Kashi et al. [23] | Grandio† | 118.8 ± 3.2 | 111.8 ± 4.4 | 125.3 ± 3.8 | 121.8 ± 3.5 |
Simile† | 67.9 ± 5.4 | 64.1 ± 5.1 | 71.0 ± 3.7 | 66.1 ± 3.8 | |
Tetric N-Ceram† | 54.4 ± 3.1 | 49.9 ± 4.5 | 56.8 ± 3.3 | 51.6 ± 6.1 | |
Muñoz et al. [16] | Microhybrid Esthet X* | 41.3 ± 0.6 | 35.1 ± 0.4 | 59.4 ± 0.7 | 52.8 ± 0.2 |
TPH Spectra* | 36.6 ± 0.5 | 30.1 ± 0.6 | 54.5 ± 0.2 | 40.7 ± 0.2 | |
Lucey et al. [8] | TPH Spectra† | 60.6 ± 1.4 | 59.0 ± 3.5 | 68.6 ± 2.3 | 68.7 ± 1.8 |
Theobaldo et al. [24] | Surefil SDR* | 49.1 ± 6.1 | 47.4 ± 5.2 | 48.8 ± 3.3 | 48.3 ± 5.0 |
Ayub et al. [25] | Vit-l-escence* | 52.0 ± 1.7 | 42.6 ± 2.4 | 57.2 ± 2.3 | 48.1 ± 1.7 |
Tetric Ceram HB | 52.3 ± 2.0 | 43.1 ± 3.3 | 53.0 ± 1.9 | 43.6 ± 3.9 | |
Filtek Supreme Ultra* | 70.8 ± 0.8 | 68.6 ± 1.7 | 76.0 ± 2.2 | 72.2 ± 2.2 | |
Filtek LS* | 49.8 ± 1.2 | 44.0 ± 0.7 | 60.1 ± 0.9 | 53.0 ± 1.9 | |
Caneppele et al. [26] | Z250* | 68.8 ± 1.7 | 45.0 ± 6.1 | 81.1 ± 2.7 | 61.0 ± 6.0 |
Mohammadi et al. [27] | Z250* | 114.7 ± 27.1 | - | 124.3 ± 22.3 | - |
Filtek Silorane* | 102.8 ± 25.7 | - | 91.5 ± 18.3 | - | |
Awliya [28] | Z250* | 90.6 ± 1.5 | 81.0 ± 1.4 | 98.2 ± 6.4 | 92.9 ± 8.3 |
Theodoridis et al. [29] | Z250* | 67.0 ± 0.2 | 57.0 ± 0.2 | 78.1 ± 0.2 | 72.0 ± 0.3 |
Filtek Silorane* | 57.3 ± 0.3 | 51.2 ± 0.3 | 66.4 ± 0.3 | 61.3 ± 0.1 | |
Dionysopoulos et al. [30] | Z250† | 65.4 ± 3.3 | 55.1 ± 2.8 | 78.4 ± 4.6 | 72.3 ± 3.8 |
GrandioSO† | 68.1 ± 3.4 | 58.1 ± 2.5 | 75.3 ± 3.6 | 68.7 ± 3.3 | |
Tantbirojn et al. [19] | Z250† | 63.0 ± 2.0 | 58.0 ± 2.0 | 63.0 ± 4.5 | 58.0 ± 3.0 |
Filtek Supreme Plus† | 53.0 ± 2.5 | 28.0 ± 1.5 | 56.0 ± 2.0 | 26.0 ± 2.5 |
Table 5
Results of the meta-analysis of the microhardness of the Z250 resin composite obtained from the top surface in the non-preheated and preheated modes

Study | N1 | N2 | Total | SMD | SE | 95% CI | t | p |
---|---|---|---|---|---|---|---|---|
Caneppele et al. [26] | 10 | 10 | 20 | −5.221 | 0.930 | −7.175 to −3.267 | ||
Mohammadi et al. [27] | 17 | 17 | 34 | −0.378 | 0.338 | −1.066 to 0.311 | ||
Awliya [28] | 5 | 5 | 10 | −1.476 | 0.659 | −2.997 to 0.0447 | ||
Theodoridis et al. [29] | 5 | 5 | 10 | −50.100 | 11.217 | −75.967 to −24.233 | ||
Dionysopoulos et al. [30] | 5 | 5 | 10 | −2.931 | 0.869 | −4.936 to −0.927 | ||
Tantbirojn et al. [19] | 5 | 5 | 10 | 0.000 | 0.571 | −1.317 to 1.317 | ||
Total (fixed effects) | 47 | 47 | 94 | −1.024 | 0.245 | −1.512 to −0.537 | −4.175 | < 0.001 |
Total (random effects) | 47 | 47 | 94 | −2.251 | 0.972 | −4.181 to −0.321 | −2.317 | 0.023 |
Table 6
Results of the meta-analysis of the microhardness of the Z250 resin composite obtained from the bottom surface in the non-preheated and preheated modes

Study | N1 | N2 | Total | SMD | SE | 95% CI | t | p |
---|---|---|---|---|---|---|---|---|
Caneppele et al. [26] | 10 | 10 | 20 | −2.533 | 0.586 | −3.764 to −1.301 | ||
Awliya [28] | 5 | 5 | 10 | −1.805 | 0.699 | −3.417 to −0.193 | ||
Theodoridis et al. [29] | 5 | 5 | 10 | −53.110 | 11.890 | −80.528 to −25.693 | ||
Dionysopoulos et al. [30] | 5 | 5 | 10 | −4.652 | 1.187 | −7.388 to −1.916 | ||
Tantbirojn et al. [19] | 5 | 5 | 10 | 0.000 | 0.571 | −1.317 to 1.317 | ||
Total (fixed effects) | 30 | 30 | 60 | −1.686 | 0.338 | −2.363 to −1.009 | −4.986 | < 0.001 |
Total (random effects) | 30 | 30 | 60 | −2.643 | 1.195 | −5.036 to −0.251 | −2.211 | 0.031 |
![]() | Figure 2Results of the meta-analysis of microhardness of the Z250 resin composite obtained from the top surface in the non-preheated and preheated modes. |
![]() | Figure 3Results of the meta-analysis of microhardness of the Z250 resin composite obtained from the bottom surface in the non-preheated and preheated modes. |
Table 7
Comparison of microhardness (Knoop hardness number; KHN) values of the Z250 resin composite obtained from the top and bottom surfaces using the non-preheated and preheated modes

Heating mode | Microhardness (KHN) | |
---|---|---|
Top | Bottom | |
Non-preheated | 67.4 ± 4.0 | 57.5 ± 5.7 |
Preheated | 78.1 ± 2.9 | 71.8 ± 3.8 |