Abstract
Escitalopram is one of selective serotonin reuptake inhibitor antidepressants. As an S-enantiomer of citalopram, it shows better therapeutic outcome in depression and anxiety disorder treatment because it has higher selectivity for serotonin reuptake transporter than citalopram. The objective of this study was to determine the direct inhibitory effect of escitalopram on 5-hydroxytryptamine type 3 (5-HT3) receptor currents and study its blocking mechanism to explore additional pharmacological effects of escitalopram through 5-HT3 receptors. Using a whole-cell voltage clamp method, we recorded currents of 5-HT3 receptors when 5-HT was applied alone or co-applied with escitalopram in cultured NCB-20 neuroblastoma cells known to express 5-HT3 receptors. 5-HT induced currents were inhibited by escitalopram in a concentration-dependent manner. EC50 of 5-HT on 5-HT3 receptor currents was increased by escitalopram while the maximal peak amplitude was reduced by escitalopram. The inhibitory effect of escitalopram was voltage independent. Escitalopram worked more effectively when it was co-applied with 5-HT than pre-application of escitalopram. Moreover, escitalopram showed fast association and dissociation to the open state of 5-HT3 receptor channel with accelerating receptor desensitization. Although escitalopram accelerated 5-HT3 receptor desensitization, it did not change the time course of desensitization recovery. These results suggest that escitalopram can inhibit 5-HT3 receptor currents in a non-competitive manner with the mechanism of open channel blocking.
Citalopram is a commonly used selective serotonin reuptake inhibitor (SSRI) to treat major depressive disorders [1]. However, citalopram is a racemic mixture of R and S-enantiomer form. Since S-enantiomer is more active than R-enantiomer, escitalopram, an S-enantiomer of the citalopram, has been developed. It shows markedly higher potency than citalopram for treating depression and anxiety disorder [2]. This could be explained by allosteric binding of escitalopram with serotonin reuptake transporter which enhances binding affinity of escitalopram at the primary binding sites, resulting in more effective blocking of 5-hydroxytryptamine (5-HT) reuptake [3].
Recently, clinical uses of escitalopram have been expanded to treat chronic pain and seasonal affective disorder [45], suggesting that the role of escitalopram is not limited to SSRI for the treatment of depressive disorders. In addition, escitalopram has adverse effects on various organs such as cardiac and nervous systems, suggesting that it might have unexpected off-target effects [67]. To explore these unidentified off-targets of escitalopram, several studies have investigated its effects on neurotransmission and ion channels by using animal model and cultured cells. Escitalopram could potentiate glutamate transmission, thereby it has faster antidepressant effect on major depressive disorders than other antidepressants [8]. Voltage-gated sodium channel and human ether-a-go-go-related gene potassium channel are directly inhibited by escitalopram [910]. However, unidentified targets of escitalopram still need to be investigated.
5-HT type 3 (5-HT3) receptor is a cation-selective ligand-gated ion channel that is gated by 5-HT. It could influence membrane depolarization and modulate neurotransmitter release at the presynaptic terminal [111213]. It is a member of the Cys-loop receptor family, which has a pentameric structure together with nicotinic acetylcholine receptors, γ-aminobutyric acid (GABA) type A receptors, and glycine receptors [1415]. 5-HT3 receptor is widely distributed in the central nervous system including postrema and nucleus tractus solitaries, hippocampus, cingulate cortex, amygdala, frontal cortex, nucleus accumbens, and dorsal horn. It is also expressed at dorsal root ganglia, colon, kidney, and immune cells [1316]. Such wide distribution of 5-HT3 receptor suggests its potential therapeutic effects on emesis, irritable bowel syndrome, schizophrenia, anxiety, substance abuse and addiction, analgesic action, and inflammatory actions [131617]. However, only antiemetic efficacy of 5-HT3 receptor antagonists is widely used clinically [1819]. For other disorders, the pathophysiologic role of 5-HT3 receptor is currently not clear enough to demonstrate the therapeutic efficacy of 5-HT3 receptor antagonist, although previous studies have suggested the possibility of its effectiveness [1820212223]. To understand the pathophysiologic role of 5-HT3 receptor in depressive disorder, past studies have tested whether 5-HT3 receptor antagonists could improve symptom of depressive disorder [24]. However, only a few antidepressants have been tested to explore its inhibitory mechanism on 5-HT3 receptor [252627]. Thus, the objective of this study was to determine the direct effect of escitalopram on 5-HT3 receptor currents using voltage-clamping technique to suggest the possible mechanism of action of escitalopram in treating depressive disorders without being limited to the function of SSRI.
NCB-20 neuroblastoma cells provided by Dr. Lovinger (National Institute on Alcohol Abuse and Alcoholism, USA) have already been revealed to show 5-HT-induced current mediated by 5-HT3 receptor, making it possible to test drug effect on 5-HT3 receptor [28293031]. They were incubated in culture flasks filled with culture medium consisting of 89% Dulbecco Modified Eagle's Medium, 10% fetal bovine serum, and 1% hypoxanthine aminopterin thymidine supplement. Cells were grown in an incubator at 37℃ with 5% CO2. Cells were harvested after treatment with 0.25% trypsin-EDTA. They were then seeded to a treated culture dish at 24–48 h prior to recording. Culture medium in dish was exchanged to extracellular solution, after which cells were moved to a recording chamber 2 h before recording.
Extracellular solution contained 150 mM NaCl, 2.5 mM KCl, 2.5 mM CaCl2, 0.1 mM MgCl2, 10 mM D-glucose and 10 mM N-(2-hydroxyethyl)piperazine-N′-2-ethansulfonic acid (HEPES). Its pH was adjusted to 7.4 with NaOH and its osmolality was adjusted to 340 mOsm/kg with sucrose. Intracellular solution contained 140 mM CsCl, 2 mM MgCl2, 5 mM ethylene glycol bis(2-aminoethylether)-N,N,N′,N′-tetraacetic acid (EGTA), 10 mM HEPES. Its pH was adjusted to 7.2 with CsOH and its osmolality was adjusted to 310 mOsm/kg with sucrose. DM IRM inverted microscope (Leica, Wetzler, Germany) was used to visualize cells. Borosilicate glass capillaries (1B150-4; World Precision Instruments, Sarasota, FL, USA) were used to make recording pipettes with a horizontal micropipette puller (P-97; Sutter Instrument, Novato, CA, USA). Pipette resistance was around 2.0 MΩ when it was filled with an intracellular solution. 5-HT3 receptor currents were recorded with an Axopatch 200B amplifier (Molecular Devices, Sunnyvale, CA, USA). They were filtered at 2 kHz and digitized at 10 kHz. Digidata 1322 and pClmap9 software (Molecular Devices) were used to save the recording data on a PC. Pipette capacitance was compensated after achieving giga-ohm seals with holding potential of −50 mV. Whole cell capacitance and series resistance were compensated after making whole-cell. Leak subtraction was not used in this study. All experiments were performed at room temperature (25℃). Holding potential was −50 mV except when testing current-voltage relationship.
An extracellular solution was continuously perfused at each side of the theta glass micropipette which were pulled from theta glass capillary tubes (Clark Borosilicate Theta; Warner Instruments, Hamden, CT, USA; 2 mm outer diameter, 1.4 mm inner diameter, 0.2 mm septum thickness) to an outer diameter of ~300 µm. After making a whole-cell configuration, cell was gently lifted with a 4-axis motorized micromanipulator (QUAD; Sutter Instrument) from the bottom of the recording chamber. Cell was moved to the side of theta glass micropipette which was flowing extracellular solution. Perfusion of extracellular or drug solutions through theta glass pipette was turn-on and -off by a perfusion valve control system (VC-8; Warner Instruments). While cell was located on the side of the extracellular solution flow at the theta glass micropipette, the agonist or escitalopram mixed agonist solution was perfused on the other side of theta glass tubing. Theta glass micropipette was mounted onto a piezo actuator (P-601 PiezoMove Linear Actuator; Physik Instrumente, Karlsruhe, Germany) which moved theta micropipette laterally in msec time resolution. Therefore, cell could be switched rapidly to the drug solution flow of theta micropipette from the extracellular solution flow. After cell was exposed to the drugs for a programmed time, it rapidly returned to the original side of the theta micropipette perfused with the extracellular solution.
Peak amplitudes, time constants, and decay slopes of currents were analyzed from the recorded data by using statistical tools in Clampfit 10 software (Molecular Devices). Decay slopes of currents were measured between 10% to 90% of the maximal amplitude. Each current amplitude was normalized to the peak current amplitude induced by 10 µM 5-HT. Normalized 5-HT or escitalopram concentration-peak amplitude data were fitted to the four-parameter logistic equation using Prism 8.0 (GraphPad Software, San Diego, CA, USA).
Equation (1) was used to calculate EC50.
The bottom of equation (1) was minimal response while the top was maximal response. X was the logarithm of 5-HT concentration and EC50 was the concentration of 5-HT producing a half response between bottom and top.
Equation (2) was used to calculate IC50.
The bottom of equation (2) was minimal response while the top was maximal response. X was the logarithm of escitalopram concentration and IC50 was the concentration of escitalopram resulting in a half response between bottom and top.
Interaction kinetics of escitalopram to opened 5-HT3 receptor are described on the basis of a first-order blocking scheme [32]. Apparent rate constants for association (k+1) and dissociation (k−1), and dissociation constant (Kd) were calculated from the following equations:
where τ was the time constant of binding of escitalopram on open 5-HT3 receptors analyzed by fitting decay of currents by escitalopram (for 5 sec) 3 sec after 5-HT application to the single exponential function.
Mean and SEM value were calculated using Prism 8.0. Statistical significance was set at p < 0.05. Student's t-test, ANOVA, and Tukey's multiple comparisons test were used for data analysis.
First, we evaluated concentration-dependent inhibitory effect of escitalopram on 5-HT3 receptor (i.e., 1, 3, 10, 30, and 100 µM of escitalopram was co-applied with 3 µM of 5-HT, near the EC50 value from our previous experiment [33]). Current peak amplitudes were then normalized to 5-HT3 receptor current induced by 3 µM 5-HT alone. Drugs were applied for 5 sec with 1 min interval. Fig. 1A shows superimposed representative current traces induced by application of 3 µM 5-HT alone or with various concentrations of escitalopram. As seen in Fig. 1B, 5-HT3 receptor currents were inhibited by escitalopram in a concentration-dependent manner. Using equation (2) in Materials and Methods, the IC50 value of escitalopram for 3 µM 5-HT induced currents was 5.35 ± 0.95 µM and Hill slope was −1.28 ± 0.13 (n = 10).
To test whether the inhibitory effect of escitalopram was competitive or non-competitive, we applied 5-HT at various concentrations (0.3, 1, 3, 10, 30 µM) along with escitalopram at 10 µM which was above IC50 value calculated in Fig. 1B. Fig. 2A shows representative 5-HT3 receptor current traces induced by various concentrations of 5-HT with or without escitalopram. 5-HT3 receptor currents were inhibited by escitalopram co-applied with 5-HT at all tested concentrations. Fig. 2B presents averaged 5-HT concentration-response curves with or without 10 µM escitalopram. Peak amplitudes of 5-HT3 receptor currents were decreased by escitalopram. It failed to reach the maximal even when 30 µM 5-HT was co-applied with 10 µM escitalopram. From these experiments, we calculated EC50 using equation (1) shown in the Materials and Methods. EC50 value of 5-HT alone (n = 9) and coapplied with escitalopram (n = 9) were 3.13 ± 0.11 µM and 6.36 ± 0.18 µM, respectively. Hill slope of 5-HT alone and co-applied with escitalopram were 2.58 ± 0.14 and 2.74 ± 0.14, respectively. Thus, escitalopram shifted the 5-HT concentration-response curve for 5-HT3 receptor currents to the right (p < 0.001, unpaired t-test) without significantly changing Hill coefficient (p = 0.4570, unpaired t-test). Escitalopram also significantly decreased the maximal response of 5-HT3 receptor currents evoked by 30 µM 5-HT; 103.0 ± 1.7% of 10 µM 5-HT induced current for 30 µM 5-HT alone and 87.8 ± 2.1% for 30 µM 5-HT co-applied with escitalopram (p < 0.001, unpaired t-test). These results suggest that escitalopram can inhibit 5-HT3 receptor function in a non-competitive manner because escitalopram increases EC50 of 5-HT on the 5-HT3 receptor currents concomitant with a decrease of maximal effect.
We next tested voltage dependency of the inhibitory effect of escitalopram on 5-HT3 receptor currents. We applied 3 µM 5-HT with or without 10 µM escitalopram to cells at holding potentials of −50, −30, −10, 0, 10, and 30 mV. Left side of Fig. 3A shows superimposed current traces of 3 µM 5-HT at various holding potentials. Right side of Fig. 3A shows 3 µM 5-HT induced currents co-applied with 10 µM escitalopram. Peak amplitude of each current was normalized to the peak amplitude of 3 µM 5-HT. Averaged results are presented in Fig. 3B. Peak currents were decreased at all tested holding potentials ranging from −50 mV to 30 mV. Reversal potential of 5-HT induced currents was 7.53 ± 0.53 mV (n = 9) by 5-HT alone and 8.43 ± 0.64 mV (n = 9) by 5-HT co-applied with escitalopram, showing no significant difference in the reversal potentials between the two (p = 0.2893, unpaired t-test). The ratio of peak amplitude between currents with or without escitalopram (I5-HT+ES/I5-HT) was not changed in any holding potential (Fig. 3C, one-way ANOVA, F = 0.7624, p = 0.5560). These results (i.e., reversal potential was not changed and inhibitory ratio was not significantly different among various holding potentials) suggested that the inhibitory effect of escitalopram was voltage independent.
In order to study whether escitalopram could bind to open form or closed form of 5-HT3 receptor ion channel, we tested effects of different application modes of escitalopram (10 µM) on 3 µM 5-HT induced currents and then compared its inhibitory effect between application modes. Fig. 4A–D show representative current traces of 3 µM 5-HT induced currents, absence of escitalopram (Fig. 4A), co-application of escitalopram (Fig. 4B), continuous application of escitalopram from 1 min prior to 5-HT application (Fig. 4C), and pre- and post-application without coapplication of escitalopram (Fig. 4D). Peak amplitudes of each application method of escitalopram was normalized to 3 µM 5-HT response. Averaged data are shown in Fig. 4E. Escitalopram inhibited peak amplitudes of 5-HT currents at each application mode. However, escitalopram exerted larger inhibition in continuous application mode (n = 10, 21.6 ± 2.7% of 3 µM 5-HT induced currents) than co-application without pretreatment (n = 10, 34.5 ± 2.8% of 3 µM 5-HT induced currents) (p < 0.001, AVOVA and Tukey's multiple comparison test). The inhibitory effect of escitalopram in pretreatment only was much smaller than continuous application and co-application modes (n = 10, 65.4 ± 3.7% of 3 µM 5-HT induced currents, p < 0.001, AVOVA and Tukey's multiple comparison test). These results suggest that escitalopram can inhibit 5-HT3 receptor by binding to both open and closed state of 5-HT3 receptor ion channel, although it preferentially acts on the open state of 5-HT3 receptor ion channel. This was because 5-HT3 receptor currents still remained even in continuous application of escitalopram (Fig. 4C). In addition, inhibitory effect by pretreatment only was not profound (Fig. 4D).
After 3 sec application of 3 µM 5-HT, escitalopram at various concentrations (1, 3, 10, 30, and 100 µM) was co-applied with 5-HT for 5 sec to investigate association and dissociation kinetics of escitalopram binding on open state of 5-HT3 receptor. Superimposed representative traces are shown in Fig. 5A. Escitalopram accelerated current decays during co-application. Currents reappeared upon discontinuation of escitalopram co-application at concentration higher than 10 µM. Association time constants for escitalopram on open 5-HT3 receptors were obtained by single exponential function fitted to current decays during escitalopram co-application. Dissociation time constants for escitalopram were obtained by single exponential function fitted to current risings upon termination of escitalopram co-application. Escitalopram decreased association time constants in a concentrationdependent manner from 1 to 100 µM. Although dissociation time constants were measurable in concentration range of 10–100 µM escitalopram, there were no significant changes in dissociation time constants upon treatment with different escitalopram concentrations (Fig. 5B). Fig. 5C shows a linear relationship of reverse values of association time constants depending on the concentration of escitalopram obtained from equation (3) as shown in the Methods. The slope of this linear function (meaning association rate constant (k+1) of escitalopram on open 5-HT3 receptors) was 0.056 µM−1sec−1. Y intercept value meaning dissociation rate constant (k−1) was 0.279 sec−1. From these association and dissociation rate constants, the calculated Kd value on open 5-HT3 receptors using equation (4) shown in the Methods was 4.99 µM. This value was very close to the IC50 of escitalopram on 5-HT3 receptor currents as shown in Fig. 1.
To study change of desensitization process of 5-HT3 receptor caused by escitalopram, we applied 10 µM 5-HT for 10 sec to induce strong desensitization and analyzed decay slopes of currents during application of 5-HT alone or with 10 µM escitalopram. As shown in Fig. 6A, current decay during 5-HT application was accelerated by escitalopram. Averaged decay slopes were increased from 0.1114 ± 0.0170 pA/msec to 0.3027 ± 0.0458 pA/msec by escitalopram (Fig. 6B, n = 10, p < 0.05, paired t-test). This indicated that escitalopram forced the open 5-HT3 receptor channel to close or desensitization, supporting the mechanism of open channel blocking. Effects of escitalopram on the time course of recovery from desensitization were studied using two pulses of 5-HT application in variable inter-pulse intervals (1, 5, 10, 30, 60 sec). Peak amplitude of the second pulse was then normalized to the first peak amplitude (paired-pulse ratio) and plotted on inter-pulse intervals. Fig. 6C and D show representative superimposed current traces evoked by two pulses of 10 µM 5-HT for 5 sec in the interpulse intervals of 1, 5, 10, 30, 60 sec with (Fig. 6D) or without (Fig. 6C) 10 µM escitalopram. A single exponential function was fitted to the 5-HT3 receptor desensitization recovery time course with a time constant of 8.80 ± 0.63 sec (n = 9) for 5-HT alone and 8.94 ± 0.70 sec (n = 9) for 5-HT co-applied with escitalopram (Fig. 6E, p = 0.8826, unpaired t-test). These results suggested that escitaolpram did not change 5-HT3 receptor desensitization recovery time course.
In this study, escitalopram reduced peak amplitude of 5-HT3 receptor current in a concentration-dependent manner. Current induced by 5-HT was almost completely blocked by escitaolpram at concentration higher than 30 µM. Escitalopram functioned as a non-competitive antagonist. Thus, escitalopram not likely bound to agonist binding sites. The inhibitory effect of escitalopram showed voltage-independency. It was more effective in the co-application mode than pre-application alone. It also accelerated the 5-HT3 receptor desensitization. The effectiveness of preapplication represents that antagonist can act as a closed channel blocker and that of co-application represents that antagonist can act as an open channel blocker [34]. Open channel antagonism is associated with channel pore blocking when it shows voltage-dependency [3435]. In our study, escitalopram showed an inhibitory effect in both pre-application mode and co-application mode, although the co-application mode was more effective than pre-application alone. This means that escitalopram prefers open state inhibition than close state inhibition. The voltage-independency from our results suggests that escitalopram is not a pore blocker. Taken together, these results indicate that escitalopram does not share the binding site with 5-HT and that it does not bind at the channel pore. Thus, allosteric site could be the most potential candidate binding site for escitalopram. Furthermore, open channel inhibition of escitalopram during co-application could be explained by allosteric modulation because escitalopram could bind to receptor independently from binding to 5-HT. Allosteric binding might also happen at close state of 5-HT3 receptor. Thus, escitalopram showed inhibitory effect in both pre-treatment mode and co-application mode. These characteristics were consistent with previous studies revealing that non-competitive inhibition with open channel block was mediated by allosteric modulation [3637]. After binding of escitalopram at allosteric site, binding affinity of 5-HT on 5-HT3 receptor could be changed, thus inhibiting the channel to open or accelerating the receptor to be desensitized. The calculated Kd value in Fig. 5C was close to the IC50 obtained from peak amplitude analysis shown in Fig. 1B. This finding could confirm these interpretations.
In addition, escitalopram showed fast association and dissociation to open 5-HT3 receptor because currents inhibited rapidly by escitalopram and rapidly re-occurred upon termination of escitalopram co-application concentration-dependently. This finding support the faster-acting characteristics of escitalopram for treating patients with major depressive disorder than other SSRIs [38]. Because 5-HT3 receptors are located in presynaptic terminals to modulate release of neurotransmitters including 5-HT [3940], an inhibitory effect of escitalopram on presynaptic 5-HT3 receptor could modulate the release of 5-HT from synaptic terminals. This effect could be additive to secondary changes of synaptic transmission, such as desensitization of presynaptic autoreceptors and inhibition of neurotransmitter synthesis [41] following a primary mechanism of escitalopram (i.e., serotonin reuptake blocking). This additive effect could contribute to pharmacologic characteristics of escitalopram, such as faster-acting than other SSRIs and withdrawal symptoms.
In conclusion, we demonstrated an inhibitory effect of escitalopram on 5-HT3 receptor by allosteric modulation. Escitalopram acted through the mechanism of open channel blocking. It underwent fast association and dissociation, reflecting its fast effectiveness and possibility of its withdrawal symptom. These results suggest that the therapeutic effect of escitalopram could be due to direct inhibition of 5-HT3 receptor function in addition to primary serotonin reuptake blocking.
Figures and Tables
Fig. 1
Inhibitory effect of escitalopram on 5-hydroxytryptamine (5-HT)3 receptor currents.
(A) Representative 5-HT3 receptor currents induced by 3 µM of 5-HT with (black traces) or without (gray trace) escitalopram at different concentrations (1, 3, 10, 30, 100 µM). Open horizontal bar indicates drug application period. 5-HT induced currents were inhibited depending on 5-HT concentration. (B) Concentration-inhibition curve of 5-HT3 receptor current peak amplitudes induced by 3 µM 5-HT co-applied with escitalopram. IC50 of escitalopram on 3 µM 5-HT induced peak amplitudes was 5.35 ± 0.95. Hill slope was −1.28 ± 0.13. Data are expressed as means ± SEM.
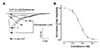
Fig. 2
Concentration-response of 5-hydroxytryptamine (5-HT) induced currents with escitalopram.
(A) Representative traces of 5-HT3 receptor currents induced by 0.3, 1, 3, 10, 30 µM of 5-HT in the presence (black traces) or absence (gray traces) of 10 µM escitalopram (ES). Open horizontal bars indicate drug application period. (B) Averaged concentration-response curve of 5-HT3 receptor current peak amplitudes in the presence (closed circle) or absence (open circle) of escitalopram. Escitalopram reduced maximal response of 5-HT induced current *p < 0.001, unpaired t-test) and increased EC50 value of 5-HT without Hill slope change. Data are expressed as means ± SEM.
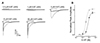
Fig. 3
Voltage independency of escitalopram effect.
(A) Representative current traces of 3 µM 5-HT at holding potentials of −50, −30, −10, 0, 10, 30 mV without (left) or with (right) escitalopram (ES). Open horizontal bars indicate drug application period. (B) Averaged normalized peak current amplitudes and holding potential (VHolding) relationship with (closed circles) or without (open circles) escitalopram. Peak current amplitudes were decreased in all tested holding potentials. However, reversal potential was not changed by escitalopram. (C) Inhibitory ratio (I5-HT+ES/I5-HT) of 5-HT induced currents by escitalopram at various holding potentials. I5-HT+ES/I5-HT at test holding potential was not significantly different. Data are expressed as means ± SEM.

Fig. 4
Inhibition of 5-hydroxytryptamine (5-HT)3 receptor currents by escitalopram in different application methods.
(A) Representative trace of 5-HT3 receptor current induced by 3 µM 5-HT alone. (B) Representative trace of 5-HT3 receptor current induced by co-application of 5-HT and 10 µM escitalopram (ES). (C) 5-HT3 receptor current trace induced by 5-HT during continuous application of escitalopram from 1 min prior to 5-HT application to the end recording. (D) 5-HT3 receptor current trace induced by 5-HT with pre-application and post-application of escitalopram. At this application method, 5-HT was applied without escitalopram co-application. Open horizontal bars indicate 5-HT application period and closed bars indicate escitalopram application. (E) Comparison of normalized peak amplitudes (IPeak) of 5-HT3 receptor currents among each application mode. Right hatched bar indicates co-application. Filled bar indicates continuous application. Dotted bar indicates pretreatment without co-application of escitalopram. Inhibitory effect by escitalopram was the smallest in the pre-application mode without co-application. *p < 0.001 between indicated pair. Data are expressed as means ± SEM.
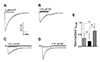
Fig. 5
Association and dissociation kinetics of escitalopram on open 5-hydroxytryptamine (5-HT)3 receptor.
(A) Representative superimposed 5-HT3 receptor currents induced by 3 µM 5-HT with 5 sec co-application of escitalopram (ES) at 0 (gray trace), 1, 3, 10, 30, or 100 µM (black traces) 3 sec after the start of 5-HT application. Open horizontal bar indicates 5-HT application period and closed bar indicates escitalopram application. (B) Average of association (open circles) and dissociation (closed circles) time constants (τ) plotted against concentration of escitalopram. (C) Plotting of reverse values of the association time constants (1/τ) against escitalopram concentration. Linear regression fitted to these values and the slope of this function (i.e., an association rate constant) and the y-axis intercept (i.e., a dissociation rate constant) were used to calculate the apparent Kd of escitalopram from equation (4) shown in the Methods. Data are expressed as means ± SEM.
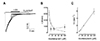
Fig. 6
Effect of escitalopram on 5-hydroxytryptamine (5-HT)3 receptor desensitization.
(A) Representative current traces of 10 sec application of 5-HT with (black trace) or without (gray trace) 10 µM escitalopram. Open horizontal bar indicates drug application period. Current decay during application of 5-HT was accelerated by escitalopram. (B) Comparison of slopes of current decay by 5-HT3 receptor desensitization. Open bar indicates 5-HT alone. Right hatched bar indicates escitalopram co-application. The decay slope was increased by escitalopram. (C, D) Superimposed sample traces evoked by two pulses of 10 µM 5-HT for 5 sec in inter-pulse intervals of 1, 5, 10, 30, 60 sec with (D) or without (C) 10 µM escitalopram. Open arrow-head indicates the first application and filled arrow-head indicates the second application of 5-HT. (E) Averaged data of paired-pulse ratio (the second peak amplitudes/the first peak amplitude) plotted against inter-pulse intervals. A single exponential function was fitted to the data and compared time constants of 5-HT alone (open circle) and co-applied with escitalopram (closed circle). Escitalopram did not change the time course of recovery from desensitization. *p < 0.001. Data are expressed as means ± SEM.
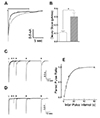
ACKNOWLEDGEMENTS
This research was supported by a grant (NRF-2017R1D-1A1B03034551) of the Basic Science Research Program through the National Research Foundation of Korea (NRF) funded by the Ministry of Science. We thank Dr. Lovinger (NIAAA, USA) for providing us with NCB-20 cells.
Notes
Author contributions Conception and design of study: Y.S.P., K.W.S. Conducting experiments, acquisition of data, analysis and interpretation of data: Y.S.P. Drafting the article: Y.S.P. Revising the article critically for important intellectual content: Y.S.P., K.W.S. Final approval of the version to be submitted: K.W.S.
References
1. Hyttel J. Citalopram--pharmacological profile of a specific serotonin uptake inhibitor with antidepressant activity. Prog Neuropsychopharmacol Biol Psychiatry. 1982; 6:277–295.
2. Waugh J, Goa KL. Escitalopram : a review of its use in the management of major depressive and anxiety disorders. CNS Drugs. 2003; 17:343–362.
3. Bræstrup C, Sanchez C. Escitalopram: a unique mechanism of action. Int J Psychiatry Clin Pract. 2004; 8 Suppl 1:11–13.


4. Thaler K, Delivuk M, Chapman A, Gaynes BN, Kaminski A, Gartlehner G. Second-generation antidepressants for seasonal affective disorder. Cochrane Database Syst Rev. 2011; (12):CD008591.


5. Patetsos E, Horjales-Araujo E. Treating chronic pain with SSRIs: what do we know? Pain Res Manag. 2016; 2016:2020915.


6. Howland RH. A question about the potential cardiac toxicity of escitalopram. J Psychosoc Nurs Ment Health Serv. 2012; 50:17–20.


7. Garfield LD, Dixon D, Nowotny P, Lotrich FE, Pollock BG, Kristjansson SD, Doré PM, Lenze EJ. Common selective serotonin reuptake inhibitor side effects in older adults associated with genetic polymorphisms in the serotonin transporter and receptors: data from a randomized controlled trial. Am J Geriatr Psychiatry. 2014; 22:971–979.


8. Björkholm C, Marcus MM, Konradsson-Geuken Å, Jardemark K, Svensson TH. The novel antipsychotic drug brexpiprazole, alone and in combination with escitalopram, facilitates prefrontal glutamatergic transmission via a dopamine D1 receptor-dependent mechanism. Eur Neuropsychopharmacol. 2017; 27:411–417.


9. Thériault O, Poulin H, Beaulieu JM, Chahine M. Differential modulation of Nav1.7 and Nav1.8 channels by antidepressant drugs. Eur J Pharmacol. 2015; 764:395–403.


10. Chae YJ, Jeon JH, Lee HJ, Kim IB, Choi JS, Sung KW, Hahn SJ. Escitalopram block of hERG potassium channels. Naunyn Schmiedebergs Arch Pharmacol. 2014; 387:23–32.


11. Sugita S, Shen KZ, North RA. 5-hydroxytryptamine is a fast excitatory transmitter at 5-HT3 receptors in rat amygdala. Neuron. 1992; 8:199–203.
12. Katsurabayashi S, Kubota H, Tokutomi N, Akaike N. A distinct distribution of functional presynaptic 5-HT receptor subtypes on GABAergic nerve terminals projecting to single hippocampal CA1 pyramidal neurons. Neuropharmacology. 2003; 44:1022–1030.


13. Thompson AJ, Lummis SC. 5-HT3 receptors. Curr Pharm Des. 2006; 12:3615–3630.
14. Barnes NM, Hales TG, Lummis SC, Peters JA. The 5-HT3 receptor--the relationship between structure and function. Neuropharmacology. 2009; 56:273–284.
15. Maricq AV, Peterson AS, Brake AJ, Myers RM, Julius D. Primary structure and functional expression of the 5HT3 receptor, a serotonin-gated ion channel. Science. 1991; 254:432–437.
16. Thompson AJ, Lummis SC. The 5-HT3 receptor as a therapeutic target. Expert Opin Ther Targets. 2007; 11:527–540.
17. Walstab J, Rappold G, Niesler B. 5-HT3 receptors: role in disease and target of drugs. Pharmacol Ther. 2010; 128:146–169.
18. Navari RM. 5-HT3 receptors as important mediators of nausea and vomiting due to chemotherapy. Biochim Biophys Acta. 2015; 1848(10 Pt B):2738–2746.
19. Smith HS, Cox LR, Smith EJ. 5-HT3 receptor antagonists for the treatment of nausea/vomiting. Ann Palliat Med. 2012; 1:115–120.
20. Gupta D, Radhakrishnan M, Kurhe Y. Ondansetron, a 5HT3 receptor antagonist reverses depression and anxiety-like behavior in streptozotocin-induced diabetic mice: possible implication of serotonergic system. Eur J Pharmacol. 2014; 744:59–66.
21. Nasirinezhad F, Hosseini M, Karami Z, Yousefifard M, Janzadeh A. Spinal 5-HT3 receptor mediates nociceptive effect on central neuropathic pain; possible therapeutic role for tropisetron. J Spinal Cord Med. 2016; 39:212–219.
22. Engleman EA, Rodd ZA, Bell RL, Murphy JM. The role of 5-HT3 receptors in drug abuse and as a target for pharmacotherapy. CNS Neurol Disord Drug Targets. 2008; 7:454–467.
23. Lovinger DM, White G. Ethanol potentiation of 5-hydroxytryptamine3 receptor-mediated ion current in neuroblastoma cells and isolated adult mammalian neurons. Mol Pharmacol. 1991; 40:263–270.
24. Rajkumar R, Mahesh R. The auspicious role of the 5-HT3 receptor in depression: a probable neuronal target? J Psychopharmacol. 2010; 24:455–469.
25. Fan P. Inhibition of a 5-HT3 receptor-mediated current by the selective serotonin uptake inhibitor, fluoxetine. Neurosci Lett. 1994; 173:210–212.
26. Eisensamer B, Rammes G, Gimpl G, Shapa M, Ferrari U, Hapfelmeier G, Bondy B, Parsons C, Gilling K, Zieglgänsberger W, Holsboer F, Rupprecht R. Antidepressants are functional antagonists at the serotonin type 3 (5-HT3) receptor. Mol Psychiatry. 2003; 8:994–1007.
27. Choi JS, Choi BH, Ahn HS, Kim MJ, Rhie DJ, Yoon SH, Min DS, Jo YH, Kim MS, Sung KW, Hahn SJ. Mechanism of block by fluoxetine of 5-hydroxytryptamine3 (5-HT3)-mediated currents in NCB-20 neuroblastoma cells. Biochem Pharmacol. 2003; 66:2125–2132.
28. Lambert JJ, Peters JA, Hales TG, Dempster J. The properties of 5-HT3 receptors in clonal cell lines studied by patch-clamp techniques. Br J Pharmacol. 1989; 97:27–40.
29. Lovinger DM, Sung KW, Zhou Q. Ethanol and trichloroethanol alter gating of 5-HT3 receptor-channels in NCB-20 neuroblastoma cells. Neuropharmacology. 2000; 39:561–570.
30. Kim KJ, Jeun SH, Sung KW. Lamotrigine, an antiepileptic drug, inhibits 5-HT3 receptor currents in NCB-20 neuroblastoma cells. Korean J Physiol Pharmacol. 2017; 21:169–177.
31. Park YS, Sung KW. Gastrokinetic agent, mosapride inhibits 5-HT3 receptor currents in NCB-20 cells. Korean J Physiol Pharmacol. 2019; 23:419–426.
32. Snyders DJ, Hondeghem LM, Bennett PB. Mechanisms of drug-channel interaction. In : Fozzard HA, Haber E, Jennings RB, Katz AM, Morgan HE, editors. The heart and cardiovascular system: scientific foundations. New York: Raven Press;1991. p. 2165–2193.
33. Park YS, Myeong SH, Kim IB, Sung KW. Tricyclic antidepressant amitriptyline inhibits 5-hydroxytryptamine 3 receptor currents in NCB-20 cells. Korean J Physiol Pharmacol. 2018; 22:585–595.


34. Föhr KJ, Zeller K, Georgieff M, Köster S, Adolph O. Open channel block of NMDA receptors by diphenhydramine. Neuropharmacology. 2015; 99:459–470.


35. Johnson JW, Kotermanski SE. Mechanism of action of memantine. Curr Opin Pharmacol. 2006; 6:61–67.


36. Wang DS, Mangin JM, Moonen G, Rigo JM, Legendre P. Mechanisms for picrotoxin block of alpha2 homomeric glycine receptors. J Biol Chem. 2006; 281:3841–3855.
37. Gunthorpe MJ, Lummis SC. Diltiazem causes open channel block of recombinant 5-HT3 receptors. J Physiol. 1999; 519 Pt 3:713–722.
38. Kasper S, Spadone C, Verpillat P, Angst J. Onset of action of escitalopram compared with other antidepressants: results of a pooled analysis. Int Clin Psychopharmacol. 2006; 21:105–110.


39. Thompson AJ, Sullivan NL, Lummis SC. Characterization of 5-HT3 receptor mutations identified in schizophrenic patients. J Mol Neurosci. 2006; 30:273–281.


40. Nayak SV, Rondé P, Spier AD, Lummis SC, Nichols RA. Calcium changes induced by presynaptic 5-hydroxytryptamine-3 serotonin receptors on isolated terminals from various regions of the rat brain. Neuroscience. 1999; 91:107–117.


41. Haggarty SJ, Perlis RH. Pharmacology of serotonergic and central adrenergic neurotransmission. In : Golan DE, editor. Principles of pharmacology: the pathophysiologic basis of drug therapy. 4th ed. Philadelphia: Wolters Kluwer;2017. p. 227–248.