Abstract
Inflammation is the crucial biological process of immune system which acts as body's defense and protective response against the injuries or infection. However, the systemic inflammation devotes the adverse effects such as multiple inflammation associated diseases. One of the best ways to treat this entity is by blocking the tumor necrosis factor alpha (TNF-α) and TNF-related apoptosis-inducing ligand (TRAIL) to avoid the proinflammation cytokines production. Thus, this study aims to evaluate the potency of Sambucus bioactive compounds as anti-inflammation through in silico approach. In order to assess that, molecular docking was performed to evaluate the interaction properties between the TNF-α or TRAIL with the ligands. The 2D structure of ligands were retrieved online via PubChem and the 3D protein modeling was done by using SWISS Model. The prediction results of the study showed that caffeic acid (−6.4 kcal/mol) and homovanillic acid (−6.6 kcal/mol) have the greatest binding affinity against the TNF-α and TRAIL respectively. This evidence suggests that caffeic acid and homovanillic acid may potent as anti-inflammatory agent against the inflammation associated diseases. Finally, this study needs further examination and evaluation to validate the potency of Sambucus bioactive compounds.
Inflammation is the metabolic condition that caused by various factors covering inflammatory inducers, sensors, and mediators.1 One of the most common term classically defined inflammation as the immunological response to the infection or injury.2 Generally, the inflammation signal activated under the intruder attack or tissue damage. The inflammation characterized by redness, swelling, heat, pain, and loss of tissue function.3 This condition commonly followed by certain pathological condition like the production of pro-inflammatory cytokines such as TNF-α and IL-1β.4 However, the excessive amount of inflammatory mediators in chronic inflammation lead to adverse effect called inflammation related diseases such as obesity, cardiovascular, neurodegenerative diseases and cancer.5678910
Based on the severity level, the inflammation are divided into two types, acute and chronic inflammation.11 During the acute inflammation condition, the cellular and molecular of biological activities attempts to suppress the negative effect of inflammation. But, ironically uncontrolled acute inflammation leads to the worst effect called chronic inflammation and commonly caused the inflammation related diseases.121314 The intervention of anti-inflammatory medication toward the inflammation associated diseases could be one of the solution to overcome this entity.15 Equally important, natural products derivate from plants, mostly flavonoids and phenolic constituents, have been used as classic therapy or medication to promote health and quality of life due to its therapeutic properties.11
Sambucus plant or elderberry is a group of shrub that widely found in almost of continents such as Europe, Asia, North Africa, and America.16 The plant extracts of Sambucus contain bioactive-rich compounds especially flavonoids and phenolic.17 Historically, Sambucus plants have been used as medical treatment against broad spectrum of diseasesin order to promoting health status. However, up to date, the study about the effect of Sambucus in inflammation associated diseases is based on very limit data.18 Therefore, this study aimed to predict the possibility of bioactive constituents of Sambucus plant as inflammatory inhibitor against inflammation associated diseases.
Accumulating evidence showed the Sambucus plants contain broad spectrum of bioactive compounds. In the present study, about 31 bioactive constituents (Table 1) from Sambucus plants were occupied against TNF-α and TRAIL protein model.17181920 The therapeutic features of bioactive compounds were assessed by PASS online prediction.21 Also, in this study, several inhibitors were used such as Thalidomide, Lenalidomide, and Pomalidomide as comparison control toward the bioactive compounds interaction with protein model (Table 1). All ligands 2D structure were retrieved from Pubchem and converted into sdf. format for next procedure.22
Accumulating evidence showed that inflammation associated diseases directly caused by the failure of immune system to take control under acute inflammation, in turn the condition worsen and leads to the chronic status.327 As metabolic disorder (Fig. 1), the chronic inflammation promotes multiple diseases such as cancer, cardiovascular diseases, pulmonary diseases, diabetes mellitus, inflammatory breast cancer, inflammatory bowel diseases, and arthritis and autoimmune diseases.327282930 General pathophysiological condition revealed both acute and chronic inflammation induce tissues injury in most of organ system. Obviously, the molecular sign of this entity is following with the high production of pro-inflammatory cytokines such as IL-1, IL-6, IL-8, IL-12, and TNF-α.3
Recently, the most common treatment for these entities is inhibiting the production of pro-inflammatory cytokines by blocking the TNF-α or TRAIL with the inhibitory drugs. Even though this approach has been established for long time ago, but there is the classic treatment that might be more potential as anti-inflammation, called natural medicine.3132 As shown in Fig. 2, the proper way to suppress inflammation is by blocking the TNF-α or TRAIL as inflammation inducer with natural products, in this case the potency of Sambucus bioactive compounds. It has been reported that flavonoids and phenolic compounds exert their biological properties as anti-inflammation by blocking the activation of NF-κB and mitogen-activated protein kinase (MAPK).11 Importantly, the TNF-α and TRAIL promote the pro-inflammatory cytokines and cell survival via the activation of NF-κB and MAPK. Therefore, by blocking the TNF-α and TRAIL can prevent the worst condition from the inflammation.3132
Numerous studies demonstrated the elderberry and elderflower contain several bioactive compounds such as flavonols, proanthocyanidins, anthocyanins, and other phenolic acids and metabolites as shown in Table 1. It has reported that the bioactive compounds have wide range of biological activities like anti-inflammatory, antioxidant, and antidiabetes.171819 Interestingly, based on the molecular docking prediction there are three selected bioactive compounds regarding their greatest potency to interact with TNF-α such as caffeic acid (−6.4 kcal/mol), protocatechuic acid (−6.3 kcal/mol), and 3-hydroxy-benzoic acid (−6.1 kcal/mol). These compounds have better binding free energy compared to the control as the thalidomide just count about 1.1 kcal/mol for binding free energy to the TNF-α (Table 2). Caffeic acid (3,4-dihydroxy-cinnamic acid) is natural compound that abundantly provided in numerous plants such as potato, carrot, apple, and berries.3334 It has been widely demonstrated that caffeic acid exerts its biological activities as anti-diabetic, antioxidant, and anti-inflammation.3536373839 Moreover, based on several studies, the caffeic acid isolated from Rhodiaola sacra and propolis has been reported to act as inflammation suppressor in lipopolysaccharide-treated inflammatory mouse model.4041 The molecular mechanism of action how the caffeic acid plays as anti-inflammatory agent through inhibiting some of enzyme activities related to inflammation such as xanthine oxidase and cyclooxygenase. Interestingly, the caffeic acid also inhibit the activation of NF-κB to reduce the production of pro-inflammatory cytokines.3641 Additionally, the molecular docking prediction also showed the top three greatest binding free energy among the TRAIL with the ligands such as homovanillic acid (−6.6 kcal/mol), 3,4-dihydroxy-phenylacetic acid (−6.5 kcal/mol), and protocatechuic acid (−6.3 kcal/mol). Importantly, these respective compounds have better potency to interact with the TRAIL compared to lenalidomide (−4.0 kcal/mol) as the control (Table 2). Homovanillic acid (3'-methoxy-4'-hydroxyphenylacetic acid) is largely known as the final product of dopamine degradation through dopamine oxidative metabolism.434445 Homovanillic acid has been used as health-promoting therapy agent because it possesses the antioxidant and antiradical activity.46
On the other hand, the docking prediction also showed numerous of amino acids residues that interact with the ligands (Fig. 3). Surprisingly, on the TNF-α – ligands interaction showed the similar amino acid residues such as GLN137, LYS174, PRO193, ILE194, TYR195, and LEU196 that found in protocatechuic acid, 3-hydroxybenzoic acid, and thalidomide interaction but not in caffeic acid. In the same way, on the TRAIL – ligands interaction also showed the similar amino acid residues such as ARG227, SER241, ILE242, and TYR243 that found in homovanillic acid, 3,4-dihydroxy-phenylacetic acid, protocatechuic acid, and lenalidomide interaction (Table 2). These results suggest that the accuracy of ligands interaction on the both protein models seemly are in the similar interaction coordinate. To greater extent, to understand more about the protein-ligands interaction features, this study explored the additional characters such as hydrophobicity, hydrogen-bonds, and interpolated charge (Fig. 4). On the protein-ligand binding, the hydrogen bonding and hydrophobicity are playing pivotal role in maintenance the protein structure and stabilizing the interaction. Moreover, the optimizing of hydrophobicity and hydrogen bonding promotes the binding affinity of the protein-ligand interaction which in turn this phenomenon can be considered as sign of good efficacy of drug.46 Additionally, the protein surface isoelectric also considered as one of the crucial part that determine the interaction stability.47
In conclusion, the results of the study showed that caffeic acid and homovanillic acid have the greatest binding against the TNF-α and TRAIL respectively. This evidence suggests that caffeic acid and homovanillic acid may potent as anti-inflammatory agents against the inflammation associated diseases. Finally, this study need further examination and evaluation to validate the potency of Sambucus bioactive compounds.
Figures and Tables
Fig. 1
Despite of their favorable function in innate immunity, the chronic inflammation also caused several types of diseases.
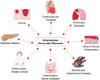
Fig. 2
Schematic illustration shows the hypothesis about the inhibition of Sambucus bioactive compounds to TNF-α or TRAIL may suppress the inflammation rate.
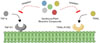
Fig. 3
Molecular docking of protein – ligands. The amino acid residues show the specific interaction to ligand. Left panel – TNF-α interacts with caffeic acid, protocatechuic acid, 3-hydroxybenzoic acid, or thalidomide. Right panel – TRAIL interacts with homovanillic acid, 3,4-dihydroxyphenylacetic acid, protocatechuic acid, or lenalidomide.
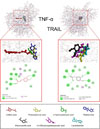
Fig. 4
Molecular interaction characters such as hydrophobicity, hydrogen-bonds, and interpolated charge on TNF-α or TRAIL interaction with the ligand. Upper panel – TNF-α interacts with caffeic acid, protocatechuic acid, 3-hydroxybenzoic acid, or thalidomide. Bottom panel – TRAIL interacts with homovanillic acid, 3,4-dihydroxyphenylacetic acid, protocatechuic acid, or lenalidomide.
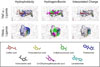
Acknowledgements
The authors thank the Laboratory of Biocomputation, Department of Biology, Brawijaya University for support this study.
References
1. Medzhitov R. Cell. 2010; 140:771–776.
2. Okin D, Medzhitov R. Curr Biol. 2012; 22:R733–R740.
3. Chen L, Deng H, Cui H, Fang J, Zuo Z, Deng J, Li Y, Wang X, Zhao L. Oncotarget. 2017; 9:7204–7218.
4. Nathan C. Nature. 2002; 420:846–852.
5. Liu Z, Wang Y, Wang Y, Ning Q, Zhang Y, Gong C, Zhao W, Jing G, Wang Q. Int Immunopharmacol. 2016; 35:210–216.
6. Czaja AJ. World J Gastroenterol. 2014; 20:2515–2532.
8. Hotamisligil GS, Erbay E. Nat Rev Immunol. 2008; 8:923–934.
9. Libby P. Am J Clin Nutr. 2006; 83:456S–460S.
10. Wyss-Coray T, Mucke L. Neuron. 2002; 35:419–432.
11. Arulselvan P, Fard MT, Tan WS, Gothai S, Fakurazi S, Norhaizan ME, Kumar SS. Oxid Med Cell Longev. 2016; 2016:5276130.
12. Chen C, Huang H, Wu CH. Methods Mol Biol. 2017; 1558:3–39.
13. Zhou Y, Hong Y, Huang H. Kidney Blood Press Res. 2016; 41:901–910.
14. Lintermans LL, Stegeman CA, Heeringa P, Abdulahad WH. Front Immunol. 2014; 5:504.
15. Schmid-Schönbein GW. Annu Rev Biomed Eng. 2006; 8:93–151.
16. Christensen LP, Kaack K, Fretté XC. Eur Food Res Technol. 2008; 227:293–305.
17. Veberic R, Jakopic J, Stampar F, Schmitzer V. Food Chemistry. 2009; 114:511–515.
18. Özgen M, Scheerens JC, Reese RN, Miller RA. Pharmacogn Mag. 2010; 6:198–203.
19. Ho GT, Wangensteen H, Barsett H. Int J Mol Sci. 2017; 18:E584.
20. Putra WE, Rifa'i M. Nat Prod Sci. 2019; 25:59–63.
21. Stepanchikova AV, Lagunin AA, Filimonov DA, Poroikov VV. Curr Med Chem. 2003; 10:225–233.
22. Kim S, Thiessen PA, Bolton EE, Chen J, Fu G, Gindulyte A, Han L, He J, He S, Shoemaker BA, Wang J, Yu B, Zhang J, Bryant SH. Nucleic Acids Res. 2016; 44:D1202–D1213.
23. Waterhouse A, Bertoni M, Bienert S, Studer G, Tauriello G, Gumienny R, Heer FT, de Beer TAP, Rempfer C, Bordoli L, Lepore R, Schwede T. Nucleic Acids Res. 2018; 46:W296–W303.
24. Putra WE, Wafaretta E, Ardiana O, Januarisasi ID, Soewondo A, Rifa'i M. Bio Research. 2017; 14:201–213.
25. Putra WE. FUW Trends Sci Technol J. 2018; 3:682–685.
27. Liu CH, Abrams ND, Carrick DM, Chander P, Dwyer J, Hamlet MRJ, Macchiarini F, PrabhuDas M, Shen GL, Tandon P, Vedamony MM. Nat Immunol. 2017; 18:1175–1180.
28. DeFronzo RA, Ferrannini E, Groop L, Henry RR, Herman WH, Holst JJ, Hu FB, Kahn CR, Raz I, Shulman GI, Simonson DC, Testa MA, Weiss R. Nat Rev Dis Primers. 2015; 1:15019.
29. Fakhoury M, Negrulj R, Mooranian A, Al-Salami H. J Inflamm Res. 2014; 7:113–120.
30. Yamauchi H, Woodward WA, Valero V, Alvarez RH, Lucci A, Buchholz TA, Iwamoto T, Krishnamurthy S, Yang W, Reuben JM, Hortobágyi GN, Ueno NT. Oncologist. 2012; 17:891–899.
31. Dai X, ZhangJ , Arfuso F, Chinnathambi A, Zayed ME, Alharbi SA, Kumar AP, Ahn KS, Sethi G. Exp Biol Med (Maywood). 2015; 240:760–773.
32. Srinivasan S, Kumar R, Koduru S, Chandramouli A, Damodaran C. Apoptosis. 2010; 15:153–161.
33. Sellappan S, Akoh CC, Krewer G. J Agric Food Chem. 2002; 50:2432–2438.
34. Mattila P, Kumpulainen J. J Agric Food Chem. 2002; 50:3660–3667.
35. Chao CY, Mong MC, Chan KC, Yin MC. Mol Nutr Food Res. 2010; 54:388–395.
36. Chao PC, Hsu CC, Yin MC. Nutr Metab (Lond). 2009; 6:33.
37. Makena PS, Chung KT. Food Chem Toxicol. 2007; 45:1899–1909.
38. Prakash D, Suri S, Upadhyay G, Singh BN. Int J Food Sci Nutr. 2007; 58:18–28.
39. Jung UJ, Lee MK, Park YB, Jeon SM, Choi MS. J Pharmacol Exp Ther. 2006; 318:476–483.
40. Armutcu F, Akyol S, Ustunsoy S, Turan FF. Exp Ther Med. 2015; 9:1582–1588.
41. Jung WK, Lee DY, Kim JH, Choi I, Park SG, Seo SK, Lee SW, Lee CM, Park YM, Jeon YJ, Lee CH, Jeon BT, Qian ZJ, Kim SK, Choi IW. Process Biochem. 2008; 43:783–787.
42. Serreli G, Deiana M. Antioxidants (Basel). 2018; 7:E170.
43. Valko-Rokytovská M, Očenáš P, Salayová A, Kostecká Z. J Chromatogr Sep Tech. 2018; 9:1–8.
44. Bonifačić D, Aralica M, Sotošek Tokmadžić V, Rački V, Tuškan-Mohar L, Kučić N. Biomarkers. 2017; 22:790–797.
45. Tuck KL, Hayball PJ, Stupans I. J Agric Food Chem. 2002; 50:2404–2409.
46. Patil R, Das S, Stanley A, Yadav L, Sudhakar A, Varma AK. PLoS One. 2010; 5:e12029.
47. Hladílková J, Callisen TH, Lund M. J Phys Chem B. 2016; 120:3303–3310.