Abstract
Background and Purpose
Various features of the cerebral cortex and white matter have been extensively investigated in migraine with aura (MwA), but the morphological characteristics of subcortical structures have been largely neglected. The aim of this study was to identify possible differences in subcortical structures between MwA patients and healthy subjects (HS), and also to determine the correlations between the characteristics of migraine aura and the volumes of subcortical structures.
Methods
Thirty-two MwA patients and 32 HS matched by sex and age were analyzed in this study. Regional subcortical brain volumes were automatically calculated using the FSL/FMRIB Image Registration and Segmentation Tool software (https://fsl.fmrib.ox.ac.uk/fsl/fslwiki/Glossary). A general linear model analysis was used to investigate differences in the volume of subcortical structures between the MwA patients and HS. A partial correlation test was used to assess correlations between the volume of subcortical structures and characteristics of MwA.
Results
The volumes of the right globus pallidus, left globus pallidus, and left putamen were significantly smaller in MwA patients than in HS (mean±SD): 1,427±135 mm3 vs. 1,557±136 mm3 (p<0.001), 1,436±126 mm3 vs. 1,550±139 mm3 (p=0.001), and 4,235±437 mm3 vs. 4,522±412 mm3 (p=0.006), respectively. There were no significant relationships between subcortical structures and clinical parameters.
Migraine is a common neurological disorder that is preceded by an aura in approximately 20–30% of migraine patients.1 Migraine aura (MA) is a phenomenon whose manifestations coincide with a wave of cortical spreading depolarization/depression (CSD) through the cerebral cortex23 and/or even through associative, commissural, and projective fibers of white matter.4 Numerous imaging studies of migraine patients have described multiple changes in the migraineur brain.5678 A few studies have investigated the role of subcortical structures in the pathophysiology of migraine,91011121314 and they revealed decreased volumes of the left caudate, putamen, right nucleus accumbens, and striatum.101214 Moreover, it was found that the basal ganglia play a significant role in the pathophysiology of pain in episodic migraine.9 However, previous studies have not explored the role of subcortical structures in the pathophysiology of migraine with aura (MwA). Also, they did not pay attention to volume and shape abnormalities in distinct subcortical structures in MwA patients. Thus, alterations of the subcortical structures in MwA patients remain insufficiently explored.
The aim of this study was to compare the volumes of subcortical structures between MwA patients and healthy subjects (HS) using advanced structural neuroimaging techniques. We also aimed to determine the correlations between the characteristics of MwA and the volumes of subcortical structures.
Participants were recruited from a registry of the Headache Center at the Neurology Clinic, Clinical Center of Serbia and diagnosed as MwA using third edition of the International Classification of Headache Disorders (ICHD-3) criteria.1 The inclusion criteria were as follows: 1) 21 to 60 years of age, 2) MwA attacks for 2 years or longer, 3) no clear structural pathology or hyperintense white-matter lesions on previous MRI scans, 4) no comorbid basal ganglia disorder or history of other neurological disorders, and 5) not receiving any prophylactic therapy for 1 year prior to the present MRI examination.
Thirty-two HS who had no neurological or metabolic diseases were voluntarily recruited from clinical staff and matched with the included migraineurs in terms of sex and age. The HS had never experienced any type of a headache other than infrequent tension-type headaches, and had no family members who suffered from migraine. All participants were screened and examined by a neurologist (J.Z.T.) to ensure that they met the inclusion/exclusion criteria, and they were all righthanded. All of the MwA patients were asked about the disease onset, the average aura duration, the annual frequency of MwA attacks, and the occurrence of visual, somatosensory, and language disturbances during MA. A questionnaire specifically designed for MA features was used in the study.15
Participants were referred to the Special Hospital for Prevention and Treatment of Cerebrovascular Diseases (SHPTCD) to undergo three-dimensional (3D) T1-weighted (T1W) and T2-weighted (T2W) MRI scans. All participants gave written informed consent and were scanned on the same MRI scanner. The research protocol of this study was approved by the Review Boards of the SHPTCD and the Neurology Clinic (IRB No. 2773/4).
MRI examinations of patients were performed using a 1.5-Tesla MRI scanner with an eight-channel head coil (Signa, General Electric Healthcare, Milwaukee, WI, USA). The imaging protocol consisted of a T2W spin-echo sequence in an axial plane [echo time (TE)=105.8 ms, repetition time (TR)=5700 ms, flip angle (FA)=90 degrees, 24 slices with 0.47×1×5 mm3 voxels, slice thickness=5 mm, acquisition matrix=512×512] and a 3D T1W fast spoiled gradient-echo (FSPGR) series (TE=3.60 ms, TR=8.12 ms, FA=15 degrees, 248 continuous slices with 0.47×0.47×1.4 mm3 voxels, slice thickness=1.4 mm, acquisition matrix=512×512, and field of view=256×256 mm2). Each MwA patient was migraine free for 72 hours prior to the MRI scan and 48 hours thereafter. T2W images were only used to exclude the presence of hyperintense white-matter lesions.
Images were transferred to a PC workstation and converted to Neuroimaging Informatics Technology Initiative (NifTI-1) format using the dcm2nii software (https://people.cas.sc.edu/rorden/mricron/dcm2nii.html). Only T1W FSPGR volumetric images were included in the analysis. In the first step, images were checked for orientation using the fslreorient2std (https://fsl.fmrib.ox.ac.uk/fsl/fslwiki/Orientation_Explained) routine in the FSL 5.0 package. The subcortical gray matter was segmented using the model-based FMRIB Image Registration and Segmentation Tool (FIRST) in the FSL 5.0 package. This tool utilizes the principles of active shape and appearance models placed within a Bayesian framework. The models were trained for 15 different subcortical structures using 336 manually segmented and labeled T1W MRI images.16 This method has been successfully implemented in several recent investigations.1718 Voxels were interpolated to 1×1×1 mm3 using the FMRIB Linear Image Registration Tool (FLIRT). The segmentations of subcortical structures were assessed visually. The brainstem was excluded because the shape model used in the FIRST extended beyond the inferior boundary of the images. We also did not investigate the volume of the nucleus accumbens due to its small volume making the measurements unreliable.19 The volume of each of the 12 extracted structures (left and right thalamus, caudate, putamen, GP, hippocampus, and amygdala) was measured in cubic millimeters. The results of subcortical structure segmentation for each subject were carefully reviewed for errors (Fig. 1) by a radiologist (I.P.) and a trained analyst (M.D.). Moreover, the total intracranial volume was computed from the highresolution T1W images of each subject using the fully automated Structural Image Evaluation, using Normalization, of Atrophy (SIENAX) tool in the FSL 5.0 package. Also, shape analysis was performed for subcortical structures that were significantly different while controlling for the effects of age, sex, and total intracranial volume.
Subject demographics and MwA characteristics were reported using descriptive statistics and compared amongst the subject groups using the independent-samples t test or chi-squared test as appropriate. The criterion for statistical significance was set at p<0.05.
The data were exported to the R statistics program (https://www.r-project.org/). A general linear model (GLM) analysis was used to investigate differences in the volumes of subcortical structures between the MwA patients and HS while controlling for the effects of age, sex and total brain volume in order to avoid spurious results. Also, the observed power based on the effect size estimated from our data was automatically derived from the GLM analysis. A partial correlation test while controlling for the effects of age, sex and total brain volume was used to assess the correlations between the volumes of subcortical structures and the following characteristics of the MwA patients: aura duration, age at disease onset, disease duration, frequency of MwA attacks, and whether or not language disturbances were present during MA. The results for the GLM and partial correlations were corrected for multiple comparisons using the false-discovery rate with the Bonferroni-Holm correction (p<0.05).
The study included 25 female and 7 male MwA patients who were matched with the same number of HS. The main demographic and clinical characteristics of the included participants are presented in Table 1.
The multivariate GLM had an observed power of 0.965 (p=0.005), indicating that the obtained results were accurate for the sample and generalizable to the population level. After correcting for multiple comparisons, the volumes of the right GP, left GP, and left putamen were significantly smaller in MwA patients than in HS: 1,427±135 mm3 (mean±SD) vs. 1,557±136 mm3 (p<0.001), 1,436±126 mm3 vs. 1,550±139 mm3 (p=0.001), and 4,235±437 mm3 vs. 4,522±412 mm3 (p=0.006), respectively (Table 2). Shape analysis showed statistically significant inward deformations in the right GP, left GP, and left putamen, with maximum differences of −0.53 mm, −0.50 mm, and −0.44 mm, respectively (Fig. 2). We observed no correlations between the volumes of subcortical structures and the occurrence of somatosensory and dysphasic symptoms, MA duration, age at disease onset, disease duration, and frequency of MwA attacks.
This study investigated differences in the volumes of subcortical gray matter structures between MwA patients and HS. Our main finding was that MwA patients exhibit significantly smaller volumes of the globus pallidi and left putamen relative to HS. However, no relationships with clinical parameters were found.
The function and structure of the GP in migraine have been investigated previously,910 but the exact connection with MwA patients was not explored. The present findings suggest that MwA patients have a smaller GP compared to HS that is not demonstrated in the population of migraineurs without aura.10
It is known that GABAergic neurons from the GP externa (GPe) project throughout the subthalamic nucleus, GP internus (GPi), substantia nigra, and striatum. Thus, GPe neurons are positioned to respond to the main basal ganglia pathways and coordinate activity throughout the brain using synaptic inhibition.20 Considering that the GPi forms the final output pathway from the basal ganglia to the cerebral cortex21 and has the ability to modulate cortical excitability and plasticity,22 the role of the GP in the pathophysiology of MA cannot be excluded. Our shape analysis of the globus pallidi revealed that both the GPe and GPi are significantly smaller in MwA patients than in HS. Since the morphological correlate of the reduced volumes of the GPe and GPi is currently unknown, it is even more difficult to speculate on the possible functional effects of these volume reductions. Moreover, the causal directions of the associations between MwA and GP volumes are unclear because of the cross-sectional design of this study. However, a reduced volume of the GP was not detected in patients with migraine without aura10 or in patients with nonmigraine or tension-type headache.14 The role of the basal ganglia in headaches and other pain conditions has not been explored sufficiently. However, the results of our study indicate that alterations in subcortical volumes could not be related to aura symptoms per se. Thus, future studies with longitudinal experimental designs should focus on the role of subcortical volumes in delineating MwA patients and migraineurs without aura.
The left putamen was also found to be smaller in our study. The putamen together with the GP make up the lenticular nucleus, which is highly interconnected with the cerebral cortex, thalamus, and brainstem. A smaller volume of putamen was demonstrated in patients with nonmigraine and tensiontype headaches,14 as well as in other pain conditions such as knee osteoarthritis and lumbar disc herniation.2324 In contrast, a larger volume putamen has been demonstrated in some chronic neuropathic pain conditions,252627 indicating that the effects of pain on the putamen and vice versa are not straightforward. Previously reported alterations in the cerebral cortex of MwA patients2829 together with the observation of lenticular volume differences in our study suggest the involvement of corticolenticular loops in the pathogenesis of MwA. It can be speculated that a smaller putamen could exert excitatory or inhibitory effects on the hyperexcitability of MwA brains. Moreover, we did not find any correlations between the volumes of subcortical structures and the disease duration or frequency of attacks, and so we are inclined to think that the observed changes are a biomarker for the migraineur brain—rather than a consequence of repetitive stimulation—triggered by CSD, which is not exclusively reserved for the cerebral cortex.4 However, significant regional atrophy of the thalamus, putamen, and GP was previously demonstrated in patients with idiopathic generalized epilepsy.3031 It is therefore possible that cumulative stress on the cerebral parenchyma (e.g., from CSD) could lead to the atrophy of the most vulnerable subcortical structures. A longitudinal study is needed to clarify this issue.
The current study had both strengths and limitations. Clear strengths of this study are 1) the inclusion of a relatively large sample of MwA patients, 2) the methodology used for investigating subcortical structures, and 3) the detailed information about MA in our sample that allowed us to investigate the connections between dysphasic symptoms and subcortical structures that have previously been neglected. Limitations of this study include its cross-sectional design, which meant that we could not determine whether the observed differences were a consequence or precondition of migraine. Also, we did not use any scales to measure depression, anxiety, or the pain intensity during a migraine attack, and hence the influences of these aspects on subcortical structures were not assessed. In addition, we did not include patients who had migraine without aura, and so we cannot suggest that the observed changes are specific to MwA. However, a recent study stressed that MwA and migraine without aura should be investigated separately, since they could be distinct disorders.32 Another limitation of this study was the use of a T1W FSPGR sequence—rather than a magnetization-prepared rapid acquisition gradient-echo sequence—with nonisotropic voxels. However, the unreliability of findings stemming from the use of nonisotropic voxels in morphometric studies had not been established. Moreover, the reliability of subcortical measures is extremely high regardless of whether nonisotropic or isotropic imaging parameters are used.33
In summary, we found that both the globus pallidi and left putamen were significantly smaller in MwA patients than in a well-matched group of HS. The importance of this result remains to be determined in future neuroimaging studies addressing the role of the basal ganglia in MwA patients.
Acknowledgements
This research received no specific grant from any funding agency in the public, commercial, or not-for-profit sectors. The authors disclosed receipt of the following financial support for the research, authorship, and/or publication of this article: I.P. and M.D. received research grant support from the Ministry of Education and Science, Republic of Serbia (project no. III 41005), and JZT received research grant support from the Ministry of Education and Science, Republic of Serbia (project no. 175022).
Notes
Author Contributions:
Conceptualization: Igor Petrusic.
Data curation: Igor Petrusic.
Formal analysis: Igor Petrusic.
Investigation: Igor Petrusic.
Methodology: Igor Petrusic, Jasna Zidverc-Trajkovic.
Supervision: Jasna Zidverc-Trajkovic.
Validation: Marko Dakovic.
Visualization: Igor Petrusic, Marko Dakovic.
Writing—original draft: Igor Petrusic.
Writing—review & editing: Marko Dakovic, Jasna Zidverc-Trajkovic.
References
1. Headache Classification Committee of the International Headache Society (IHS). The international classification of headache disorders, 3rd edition. Cephalalgia. 2018; 38:1–211.
2. Hadjikhani N, Sanchez Del Rio M, Wu O, Schwartz D, Bakker D, Fischl B, et al. Mechanisms of migraine aura revealed by functional MRI in human visual cortex. Proc Natl Acad Sci U S A. 2001; 98:4687–4692. PMID: 11287655.


3. Rogawski MA. Common pathophysiologic mechanisms in migraine and epilepsy. Arch Neurol. 2008; 65:709–714. PMID: 18541791.


4. Martens-Mantai T, Speckmann EJ, Gorji A. Propagation of cortical spreading depression into the hippocampus: the role of the entorhinal cortex. Synapse. 2014; 68:574–584. PMID: 25049108.


5. Kim JH, Suh SI, Seol HY, Oh K, Seo WK, Yu SW, et al. Regional grey matter changes in patients with migraine: a voxel-based morphometry study. Cephalalgia. 2008; 28:598–604. PMID: 18422725.


6. DaSilva AF, Granziera C, Snyder J, Hadjikhani N. Thickening in the somatosensory cortex of patients with migraine. Neurology. 2007; 69:1990–1995. PMID: 18025393.


7. Bashir A, Lipton RB, Ashina S, Ashina M. Migraine and structural changes in the brain: a systematic review and meta-analysis. Neurology. 2013; 81:1260–1268. PMID: 23986301.


8. Chong CD, Schwedt TJ, Dodick DW. Migraine: what imaging reveals. Curr Neurol Neurosci Rep. 2016; 16:64. PMID: 27181270.


9. Maleki N, Becerra L, Nutile L, Pendse G, Brawn J, Bigal M, et al. Migraine attacks the basal ganglia. Mol Pain. 2011; 7:71. PMID: 21936901.


10. Yuan K, Zhao L, Cheng P, Yu D, Zhao L, Dong T, et al. Altered structure and resting-state functional connectivity of the basal ganglia in migraine patients without aura. J Pain. 2013; 14:836–844. PMID: 23669074.


11. Liu J, Lan L, Li G, Yan X, Nan J, Xiong S, et al. Migraine-related gray matter and white matter changes at a 1-year follow-up evaluation. J Pain. 2013; 14:1703–1708. PMID: 24290450.
12. Magon S, May A, Stankewitz A, Goadsby PJ, Tso AR, Ashina M, et al. Morphological abnormalities of thalamic subnuclei in migraine: a multicenter MRI study at 3 tesla. J Neurosci. 2015; 35:13800–13806. PMID: 26446230.


13. Chong CD, Dumkrieger GM, Schwedt TJ. Structural co-variance patterns in migraine: a cross-sectional study exploring the role of the hippocampus. Headache. 2017; 57:1522–1531. PMID: 28976002.


14. Husøy AK, Pintzka C, Eikenes L, Håberg AK, Hagen K, Linde M, et al. Volume and shape of subcortical grey matter structures related to headache: a cross-sectional population-based imaging study in the Nord-Trøndelag Health Study. Cephalalgia. 2019; 39:173–184. PMID: 29848110.


15. Petrusic I, Zidverc-Trajkovic J, Podgorac A, Sternic N. Underestimated phenomena: higher cortical dysfunctions during migraine aura. Cephalalgia. 2013; 33:861–867. PMID: 23430982.


16. Patenaude B, Smith SM, Kennedy DN, Jenkinson M. A Bayesian model of shape and appearance for subcortical brain segmentation. Neuroimage. 2011; 56:907–922. PMID: 21352927.


17. Sameti M, Smith S, Patenaude B, Fein G. Subcortical volumes in longterm abstinent alcoholics: associations with psychiatric comorbidity. Alcohol Clin Exp Res. 2011; 35:1067–1080. PMID: 21332530.


18. Amann M, Andělová M, Pfister A, Mueller-Lenke N, Traud S, Reinhardt J, et al. Subcortical brain segmentation of two dimensional T1-weighted data sets with FMRIB's Integrated Registration and Segmentation Tool (FIRST). Neuroimage Clin. 2014; 7:43–52. PMID: 25610766.


19. Nugent AC, Luckenbaugh DA, Wood SE, Bogers W, Zarate CA Jr, Drevets WC. Automated subcortical segmentation using FIRST: test-retest reliability, interscanner reliability, and comparison to manual segmentation. Hum Brain Mapp. 2013; 34:2313–2329. PMID: 22815187.


21. Hoover JE, Strick PL. Multiple output channels in the basal ganglia. Science. 1993; 259:819–821. PMID: 7679223.


22. Ni Z, Kim SJ, Phielipp N, Ghosh S, Udupa K, Gunraj CA, et al. Pallidal deep brain stimulation modulates cortical excitability and plasticity. Ann Neurol. 2018; 83:352–362. PMID: 29369401.


23. Mao CP, Bai ZL, Zhang XN, Zhang QJ, Zhang L. Abnormal subcortical brain morphology in patients with knee osteoarthritis: a crosssectional study. Front Aging Neurosci. 2016; 8:3. PMID: 26834629.


24. Luchtmann M, Steinecke Y, Baecke S, Lützkendorf R, Bernarding J, Kohl J, et al. Structural brain alterations in patients with lumbar disc herniation: a preliminary study. PLoS One. 2014; 9:e90816. PMID: 24595036.


25. Mao C, Wei L, Zhang Q, Liao X, Yang X, Zhang M. Differences in brain structure in patients with distinct sites of chronic pain: a voxel-based morphometric analysis. Neural Regen Res. 2013; 8:2981–2990. PMID: 25206618.
26. Wu Q, Inman RD, Davis KD. Neuropathic pain in ankylosing spondylitis: a psychophysics and brain imaging study. Arthritis Rheum. 2013; 65:1494–1503. PMID: 23460087.


27. Schmidt-Wilcke T, Luerding R, Weigand T, Jürgens T, Schuierer G, Leinisch E, et al. Striatal grey matter increase in patients suffering from fibromyalgia--a voxel-based morphometry study. Pain. 2007; 132(Suppl 1):S109–S116. PMID: 17587497.
28. Messina R, Rocca MA, Colombo B, Valsasina P, Horsfield MA, Copetti M, et al. Cortical abnormalities in patients with migraine: a surface-based analysis. Radiology. 2013; 268:170–180. PMID: 23533286.


29. Petrusic I, Dakovic M, Kacar K, Zidverc-Trajkovic J. Migraine with aura: surface-based analysis of cerebral cortex with magnetic resonance imaging. Korean J Radiol. 2018; 19:767–776. PMID: 29962883.
30. Du H, Zhang Y, Xie B, Wu N, Wu G, Wang J, et al. Regional atrophy of the basal ganglia and thalamus in idiopathic generalized epilepsy. J Magn Reson Imaging. 2011; 33:817–821. PMID: 21448945.


31. Peng SJ, Harnod T, Tsai JZ, Ker MD, Chiou JC, Chiueh H, et al. Evaluation of subcortical grey matter abnormalities in patients with MRInegative cortical epilepsy determined through structural and tensor magnetic resonance imaging. BMC Neurol. 2014; 14:104. PMID: 24885823.


32. Szabó N, Faragó P, Király A, Veréb D, Csete G, Tóth E, et al. Evidence for plastic processes in migraine with aura: a diffusion weighted MRI study. Front Neuroanat. 2018; 11:138. PMID: 29387002.


33. Wonderlick JS, Ziegler DA, Hosseini-Varnamkhasti P, Locascio JJ, Bakkour A, Van der Kouwe A, et al. Reliability of MRI-derived cortical and subcortical morphometric measures: effects of pulse sequence, voxel geometry, and parallel imaging. Neuroimage. 2009; 44:1324–1333. PMID: 19038349.


Fig. 2
Three-dimensional visualization of the results of shape analysis of the subcortical structures that were significantly smaller in MwA patients than in healthy subjects. MwA patients showed significant inward deformations of the right globus pallidus, left globus pallidus, and left putamen (A, upper view; B, lower view). Significant inward deformations are quantified on a -log(p) scale, with blue indicating the greatest inward deformations. The results are uncorrected for multiple comparisons, and hence are for illustrative purposes only. MwA: migraine with aura.
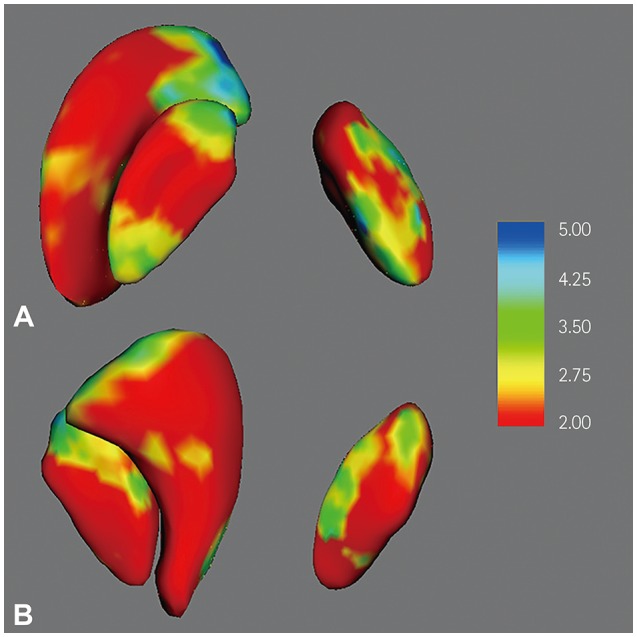