INTRODUCTION
Stroke or cerebrovascular accident is a major cause of death along with malignant neoplasm and cardiovascular disease. As serious disability can persist among survivors, post-stroke intensive and comprehensive rehabilitation is important in facilitating functional recovery and minimizing disability [
1].
Evaluating the initial functional status and prognostic factors is useful for establishing effective rehabilitation plans for patients with subacute stroke. One study suggested that age, initial National Institutes of Health Stroke Scale (NIHSS) score, and severity of upper-limb paresis are associated with activities of daily living (ADL) [
2]. Another study suggested that age, sex, urinary incontinence, initial trunk control function, and initial NIHSS score were associated with ambulatory function at 6 months post-stroke [
3]. However, these are not modifiable risk factors even after detection. Thus, controllable factors such as vital signs and laboratory parameters are more important for secondary prevention and functional recovery. The laboratory parameters that can help predict post-stroke functional recovery have not been identified. One study reported that C-reactive protein (CRP) levels may be associated with post-stroke functional outcome, but this finding remains controversial [
4]. Another group suggested that a moderate blood pressure reduction in acute ischemic stroke could regulate brain edema and facilitate functional recovery [
5]. Others showed that the administration of metformin might regulate diabetes mellitus, the risk factor of stroke, thereby attaining a favorable neurological outcome [
6].
However, although hypokalemia is a known risk factor of cardiovascular disease or stroke, few studies have examined serum potassium levels, which commonly fluctuate after stroke, as a prognostic factor of functional outcome after stroke [
7-
10]. Another study reported that a poor functional prognosis was observed when hypokalemia was present in patients with acute ischemic stroke [
11]. Here we further evaluated the functional status before and after the rehabilitation of subacute stroke patients with hypokalemia to prove the hypothesis that hypokalemic patients have worse functional recovery after stroke than normokalemic patients.
MATERIALS AND METHODS
Patients
This retrospective case-control study included 96 consecutive post-stroke hemiplegic patients who visited a single tertiary hospital and were diagnosed with acute stroke between March 2014 and February 2018. All patients were admitted to the department of neurology or neurosurgery and subsequently transferred to the department of rehabilitation medicine after acute treatment. All patients underwent laboratory examinations upon admission. The patients with serum potassium levels < 3.5 mmol/L on admission or hypokalemia within 7 days after onset were classified into the hypokalemic group. The exclusion criteria were as follows: discharge without rehabilitation or functional assessment, quadriplegia, and previous history of stroke. Finally, 46 patients were included in the hypokalemic group and 50 patients whose serum potassium levels were maintained within the normal range (3.5–5.5 mmol/L) for 1 week after admission were included in the normokalemic group. All patients received comprehensive rehabilitation treatment, including physical and occupational therapy. This study conformed to the principles of the Declaration of Helsinki.
To clarify whether a difference existed between long-lasting and transient hypokalemia, hypokalemia duration from onset to the day the serum potassium level was normalized was also determined in the hypokalemic group. The management method for hypokalemia and the timing of replacement were decided by each physician. Serum electrolyte levels were assessed daily or every other day and monitored over time until normalized.
Baseline characteristics
The baseline characteristics of the 2 groups were compared by medical record review and included age, sex, hospitalization duration, and risk factors of stroke and those related to serum electrolyte levels such as hypertension, diabetes mellitus, dyslipidemia, cardiac disease, arrhythmia, renal impairment, previous use of oral diuretics, vomiting, and smoking were reviewed. The classification of stroke and the hemiplegic side were also compared. Serum sodium, potassium, homocysteine, and CRP levels and erythrocyte sedimentation rate (ESR) were recorded. To evaluate and compare the cognitive, motor function and performance level of ADL, the NIHSS and Glasgow Coma Scale (GCS) scores were assessed on the day of admission, while Mini Mental Status Examination (MMSE), Hemispheric Stroke Scale (HSS), Functional Ambulation Category (FAC), and Functional Independence Measure (FIM) scores were assessed on the day of transfer to the department of rehabilitation.
In-hospital medical complications
To measure the effect of post-stroke medical complications on hospitalization length, we compared the incidence of stroke complications between the 2 groups. The frequent complications or comorbidities that have been assumed to have potential for poor post-stroke outcome included in-hospital respiratory, urinary tract, or central nervous system infection, delirium, number of operations, and initial abnormal electrocardiography findings.
Outcome measurements
Here we aimed to compare the degree of recovery with emphasis on motor and cognitive dysfunction, major problems that occur after stroke. Therefore, all patients underwent neurologic examinations and were assessed with several functional scales before and after rehabilitation. The FAC score and FIM locomotion (FIMlocomotion) subscore were assessed to determine gait function. The motor function items of the HSS was assessed to evaluate muscle strength improvements based on the Medical Research Council (MRC) scores for the proximal and distal parts of the upper and lower extremities (MRC 5, 0 points; MRC 4+, 1 point; MRC 4, 2 points; MRC 4−, 3 points; MRC 3, 4 points; MRC 2, 5 points; MRC 1, 6 points; and MRC 0, 7 points). The MMSE was used to assess cognitive function. The delta score between the pre and the post-rehabilitation score was expressed such as ΔMMSE, ΔFAC, ΔFIM and ΔHSS.
Statistical analysis
Student's t-test and the χ
2 test (Fisher's exact test) were used to determine intergroup differences. To analyze the correlation between the serum potassium levels and the delta parameters of the functional outcomes, a Pearson correlation analysis was performed. Pearson's correlation coefficients were obtained between serum sodium-to-potassium ratio and the delta parameter values of the functional outcomes before versus after treatment. The Pearson's correlation coefficient between the disease duration of hypokalemia and functional recovery was also assessed [
12]. Pearson's correlation coefficients were obtained between serum sodium-to-potassium ratio and the delta parameter values of the functional outcomes before versus after treatment. The Pearson's correlation coefficient between the disease duration of hypokalemia and functional recovery was also assessed. To determine the independent risk factor of hypokalemia, univariate and multivariate logistic regression analysis was performed. The p values < 0.05 were considered statistically significant. SPSS version 23.0 (SPSS Inc., Chicago, IL, USA) was used for the statistical analysis.
RESULTS
Baseline characteristics were compared between the hypokalemic (n = 46) and normokalemic (n = 50) groups. The mean serum potassium levels of the 2 groups were 3.30 and 4.02 mmol/L, while the mean serum sodium/potassium ratios were 41.91 and 34.35, respectively. Serum sodium and homocysteine and CRP levels and ESR did not differ between groups.
Table 1 summarizes the patients' baseline characteristics. Age, sex, stroke classification, hemiplegic side and medical history did not differ between groups, except the prevalence of the history of previous use of oral diuretics was significantly higher in the hypokalemic group (p = 0.03).
Table 1
Baseline characteristics of the patients
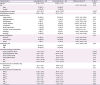
Characteristics |
Hypokalemia (n = 46) |
Normokalemia (n = 50) |
Difference (95% CI) |
p value |
Age (yr) |
61.56 ± 15.01 |
62.80 ± 15.10 |
|
0.69 |
Sex |
|
|
0.07 (−0.12–0.26) |
0.53 |
|
Male |
27 (58.7) |
33 (66.0) |
|
Female |
19 (41.3) |
17 (34.0) |
Hospitalization duration |
47.15 ± 13.71*
|
38.12 ± 10.33*
|
|
< 0.001*
|
Rehabilitation duration |
24.78 ± 7.50 |
23.74 ± 7.50 |
|
0.50 |
Medical history |
|
|
|
|
|
Hypertension |
30 (65.2) |
29 (58.0) |
0.07 (−0.12–0.26) |
0.47 |
|
Diabetes mellitus |
5 (10.9) |
7 (14.0) |
0.03 (−0.11–0.17) |
0.76 |
|
Dyslipidemia |
4 (8.7) |
3 (6.0) |
0.03 (−0.09–0.15) |
0.71 |
|
Cardiac disease |
10 (21.7) |
11 (22.0) |
0.003 (−0.16–0.17) |
0.98 |
|
Arrhythmia |
14 (30.4) |
13 (26.0) |
0.04 (−0.13–0.22) |
0.63 |
|
Renal impairment |
5 (10.9) |
7 (14.0) |
0.03 (−0.11–0.17) |
0.76 |
|
Previous use of oral diuretics |
11 (23.9) |
4 (8.0) |
0.16 (0.01–0.31) |
0.03*
|
|
Vomiting |
4 (8.7) |
0 (0.0) |
0.09 (−0.002–0.20) |
0.06 |
|
Smoking |
16 (34.8) |
12 (24.0) |
0.11 (−0.07–0.28) |
0.27 |
Stroke classification |
|
|
|
0.54 |
|
Ischemic |
18 (39.1) |
20 (40.0) |
0.01 (−0.18–0.20) |
|
Hemorrhagic |
26 (56.5) |
25 (50.0) |
0.07 (−0.13–0.25) |
|
Mixed |
2 (4.3) |
5 (10.0) |
0.06 (−0.06–0.17) |
Hemiplegic side |
|
|
0.11 (−0.09–0.29) |
0.32 |
|
Right |
20 (43.5) |
27 (54.0) |
|
Left |
26 (56.5) |
23 (46.0) |
Laboratory findings at admission |
|
|
|
|
|
Serum potassium level |
3.30 ± 0.22 |
4.02 ± 0.29 |
|
< 0.001*
|
|
Sodium/potassium ratio |
41.91 ± 2.75 |
34.35 ± 2.56 |
|
< 0.001*
|
|
Serum sodium level |
137.7 ± 2.71 |
137.3 ± 2.83 |
|
0.47 |
|
Homocysteine level |
10.76 ± 4.95 |
10.15 ± 4.65 |
|
0.59 |
|
ESR |
18.41 ± 20.94 |
24.63 ± 24.88 |
|
0.19 |
|
CRP |
16.24 ± 52.42 |
11.77 ± 25.55 |
|
0.60 |
Functional scores on admission day |
|
|
|
|
|
NIHSS score |
10.11 ± 6.16 |
9.64 ± 6.39 |
|
0.81 |
|
GCS score |
11.80 ± 3.58 |
13.16 ± 2.81 |
|
0.18 |
Functional scores on transfer day |
|
|
|
|
|
MMSE |
17.39 ± 10.61 |
18.74 ± 10.31 |
|
0.53 |
|
HSSmotor
|
17.65 ± 6.56 |
15.44 ± 6.01 |
|
0.09 |
|
HSSupper
|
9.13 ± 3.26 |
7.88 ± 3.15 |
|
0.06 |
|
HSSlower
|
8.52 ± 3.51 |
7.56 ± 3.13 |
|
0.16 |
|
FAC |
0.91 ± 1.31 |
0.76 ± 1.08 |
|
0.53 |
|
FIMtotal
|
51.22 ± 27.67 |
59.16 ± 22.54 |
|
0.13 |
|
FIMlocomotion
|
1.78 ± 1.47 |
1.90 ± 1.39 |
|
0.69 |
A significant intergroup difference in hospitalization period was found (hypokalemic group, 47.2 days; normokalemic group, 38.1 days; p < 0.001). However, no significant difference was found in the hospitalization period for comprehensive rehabilitation treatment (hypokalemic group, 24.8 days; normokalemic group, 23.7 days; p = 0.50). No significant differences were found in the baseline characteristics with respect to early neurologic findings reflected by NIHSS, GCS, MMSE, HSS, FAC, and FIM scores.
Intergroup comparison of functional recovery
The primary outcomes were compared between the hypokalemic and normokalemic groups using the delta parameters of the dependent variables (
Table 2). In hypokalemia group, significant lower intergroup differences were found in the mean ΔFAC score, ΔHSS lower extremity (ΔHSS
lower) and total motor (ΔHSS
motor) scores. No significant differences were observed in the ΔMMSE and ΔFIM
locomotion scores; however, in ΔFIM
locomotion score, the mean value of the delta parameter tended to be lower in the hypokalemic group than in the normokalemic group (ΔFAC, p = 0.001; ΔHSS
upper, p = 0.05; ΔHSS
lower, p =0.01; ΔHSS
motor, p = 0.02; ΔMMSE, p = 0.45; and ΔFIM
locomotion, p = 0.08).
Table 2
Comparison of functional improvement between the hypokalemic and normokalemic groups of patients with subacute stroke
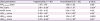
Delta parameters |
Hypokalemia (n = 46) |
Normokalemia (n = 50) |
p value |
FIMlocomotion score |
1.37 ± 1.76 |
1.98 ± 1.65 |
0.08 |
FAC score |
0.91 ± 0.99*
|
1.66 ± 1.10*
|
0.001*
|
MMSE score |
2.85 ± 4.30 |
2.26 ± 3.33 |
0.45 |
HSSmotor score |
0.83 ± 1.45*
|
2.18 ± 3.63*
|
0.02*
|
HSSupper score |
0.46 ± 0.98*
|
1.04 ± 1.81*
|
0.050 |
HSSlower score |
0.37 ± 0.68*
|
1.10 ± 1.80*
|
0.01*
|
Linear correlation between serum potassium level and functional recovery
In the linear correlation analysis between the delta parameter values and the serum potassium levels, the ΔFAC and ΔHSS
lower and ΔHSS
motor scores showed significant positive correlations with the serum potassium level (ΔHSS
lower score [
r = 0.25, p = 0.01], ΔHSS
motor score [
r = 0.21, p = 0.04], and ΔFAC score [
r = 0.34, p = 0.001]) (
Table 3,
Fig. 1).
Table 3
Pearson correlation coefficient (r) of functional improvement in the patients with subacute stroke with initial serum potassium levels and initial serum sodium-to-potassium ratio
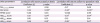
Delta parameters |
Correlation with serum potassium levels |
Correlation with serum sodium-to-potassium ratio |
Coefficient (r) |
p value |
Coefficient (r) |
p value |
FIMlocomotion score |
0.17 |
0.09 |
−0.17 |
0.09 |
FAC score |
0.34 |
0.001*
|
−0.34*
|
0.001*
|
MMSE score |
−0.02 |
0.84 |
−0.002 |
0.99 |
HSSmotor score |
0.21 |
0.04*
|
−0.21*
|
0.04*
|
HSSupper score |
0.16 |
0.13 |
−0.15 |
0.14 |
HSSlower score |
0.25 |
0.01*
|
−0.25*
|
0.01*
|
Fig. 1
Linear relationship of functional improvement with initial serum potassium levels and with serum sodium-to-potassium ratio; ΔFAC (A), ΔHSSlower (B) and ΔHSSmotor (C) with serum potassium levels and ΔFAC (D), ΔHSSlower (E) and ΔHSSmotor (F) with serum sodium-to-potassium ratio.
FAC, Functional Ambulation Category; HSS, Hemispheric Stroke Scale.
Linear correlation between serum sodium-to-potassium ratio and functional recovery
Serum sodium-to-potassium ratio showed a more significant association with functional recovery than did serum potassium level (
Table 3,
Fig. 1). Significant negative correlations were seen with ΔHSS
lower (
r = −0.25, p = 0.01), ΔHSS
motor (
r = −0.21, p = 0.04), and ΔFAC (
r = −0.34, p = 0.001) scores.
Linear correlation between hypokalemia duration and functional recovery
Fig. 2 showed that hypokalemia duration was not significantly correlated with functional recovery in hypokalemia group (ΔMMSE, p = 0.92; ΔHSS
motor, p = 0.55; ΔHSS
upper, p = 0.42; ΔHSS
lower, p = 0.93; ΔFAC, p = 0.74). Potassium replacement was performed through the oral or intravenous route in hypokalemic patients. In the hypokalemic group, 11 patients (23.9%) underwent potassium replacement via the oral route, while 23 patients (50.0%) underwent it via the intravenous route. Serum potassium level normalized spontaneously in 12 patients (26.1%) without potassium replacement.
Fig. 2
Linear relationship between hypokalemia duration and the delta parameter values of functional outcome: ΔMMSE (A), ΔHSSupper (B), ΔHSSlower (C), ΔHSSmotor (D), ΔFAC (E), and ΔFIMlocomotion (F).
MMSE, Mini Mental Status Examination; HSS, Hemispheric Stroke Scale; FAC, Functional Ambulation Category; FIMlocomotion, Functional Independence Measure locomotion subscore.
Comparison of in-hospital complications and comorbidities between the hypokalemic and normokalemic groups
The intergroup comparison of in-hospital complications and comorbidities is summarized in
Table 4. The total number of surgical interventions and the in-hospital infection rate, especially that of urinary tract infection, were significantly higher in the hypokalemic group (urinary tract infection, p = 0.01; total in-hospital infection, p = 0.01; number of surgical interventions, p < 0.001).
Table 4
Stroke complications or comorbidities
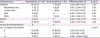
Characteristics |
Hypokalemia (n = 46) |
Normokalemia (n = 50) |
Difference (95% CI) |
p value |
Infection |
18 (39.1)*
|
7 (14.0)*
|
0.25 (0.08–0.41) |
0.01*
|
|
Respiratory tract |
7 (15.2) |
4 (8.0) |
0.07 (−0.06–0.21) |
0.27 |
|
Urinary tract |
8 (17.4) |
1 (2.0) |
0.15 (0.04–0.29) |
0.01*
|
|
CNS |
2 (4.4) |
0 |
0.04 (−0.03–0.15) |
0.07 |
|
Other |
1 (2.2) |
2 (4.0) |
0.02 (−0.08–0.11) |
0.30 |
Seizure |
2 (4.4) |
0 |
0.04 (−0.03–0.15) |
0.14 |
ECG |
31 (67.4) |
31 (62.0) |
0.05 (−0.13–0.24) |
0.59 |
Venous thromboembolism |
0 |
0 |
|
|
No. of surgical intervention |
|
|
|
< 0.001*
|
|
0 |
16 (34.8) |
34 (68.0) |
0.33 (0.13–0.50) |
|
1 |
26 (56.5) |
16 (32.0) |
0.25 (0.05–0.42) |
|
>2 |
4 (8.7) |
0 (0.0) |
0.09 (−0.002–0.20) |
Univariate and multivariate analysis of independent risk factor of hypokalemia which impedes rehabilitation
All risk factors (p < 0.05) that were significantly associated in the univariate model were considered as subjects of multivariate analysis and are shown in
Table 5. On univariate logistic regression analysis, hospitalization duration (odds ratio [OR], 1.06; 95% confidence interval [CI], 1.02–1.10, p = 0.003) and previous use of oral diuretics history (OR, 3.61; 95% CI, 1.06–12.32, p = 0.04) were independent risk factors for hypokalemia. Multivariate logistic regression analysis also shows hospitalization duration and previous use of oral diuretics history as significant risk factors for hypokalemia (hospitalization duration (OR, 1.08; 95% CI, 1.03–1.14, p = 0.002), previous use of oral diuretics (OR, 5.35; 95% CI, 1.08–26.58, p = 0.04)). Other risk factors did not show significant association both in univariate and multivariate analysis.
Table 5
Univariate and multivariate logistic regression analysis of independent risk factors for hypokalemia
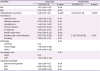
Variables |
Univariate |
Multivariate |
OR (95% CI) |
p value |
OR (95% CI) |
p value |
Sex |
1.37 (0.60–3.13) |
0.46 |
|
|
Age |
1.00 (0.97–1.02) |
0.69 |
|
|
Hospitalization duration |
1.06 (1.02–1.10) |
0.003*
|
1.08 (1.03–1.14) |
0.002*
|
Past history |
|
|
|
|
|
Hypertension |
1.36 (0.59–3.10) |
0.47 |
|
|
|
Diabetes mellitus |
0.75 (0.22–2.55) |
0.64 |
|
|
|
Dyslipidemia |
1.49 (0.32–7.06) |
0.61 |
|
|
|
Cardiac disease |
0.99 (0.37–2.60) |
0.98 |
|
|
|
Arrhythmia |
1.25 (0.51–3.04) |
0.63 |
|
|
|
Renal impairment |
0.75 (0.22–2.55) |
0.64 |
|
|
|
Previous use of oral diuretics |
3.61 (1.06–12.32) |
0.04*
|
5.35 (1.08–26.58) |
0.04*
|
|
Smoking |
1.69 (0.70–4.11) |
0.25 |
|
|
Pathology |
|
0.55 |
|
|
|
Ischemic |
1.00 |
|
|
|
Hemorrhagic |
1.16 (0.50–2.68) |
|
|
|
Mixed |
0.44 (0.08–2.58) |
|
|
Hemiplegic side |
|
0.30 |
|
|
|
Right |
1.00 |
|
|
|
Left |
1.53 (0.68–3.42) |
|
|
Laboratory findings |
|
|
|
|
|
Serum homocysteine |
1.02 (0.92–1.13) |
0.68 |
|
|
|
ESR |
0.99 (0.96–1.02) |
0.35 |
|
|
|
CRP |
1.01 (0.99–1.02) |
0.41 |
|
|
DISCUSSION
This study hypothesized that a relationship exists between serum hypokalemia and post-stroke functional prognosis in patients with subacute stroke. In this study, motor function was less improved in the hypokalemic group than in the normokalemic group, especially in terms of HSSlower, HSSmotor, and FAC scores. Therefore, we suggest that hypokalemia would be associated with a decreased recovery of post-stroke ambulatory function.
To date, few studies have examined the effect of hypokalemia at admission on post-stroke functional outcomes. The neurological recovery that occurs in the first few days to several weeks after stroke onset is determined by the recovery of the ischemic penumbra and the resolution of edema and associated mass effects [
13]. Hypokalemia may cause vasoconstriction, neural injury, and brain edema, which may be an inhibitory factor of neurologic recovery in patients with stroke [
714]. One study proposed 2 hypotheses regarding the mechanism underlying serum hypokalemia in patients with stroke [
7]. First, stroke-induced catecholamine mediates the transport of serum potassium into the intracellular fluid by stimulating the beta-2 adrenoceptor. Second, the potassium level might decrease from the time of stroke onset owing to the lack of previous dietary potassium supplement. A previous study also suggested that serum hypokalemia lowers the extracellular potassium level, which may induce cellular membrane depolarization and calcium ion influx, leading to ischemia and neural injury [
7]. Furthermore, one study reported that owing to electrolyte disturbance, the accumulation of metabolites such as arachidonic and lactic acids could aggravate brain edema and consequently damage the brain parenchyma [
14]. Another study suggested that hypokalemia is associated with a high mortality rate due to stroke regardless of age and hypertension history [
7]. However, those authors only evaluated mortality and did not describe in detail the functional outcomes. A recent study suggested that the Modified Rankin Scale score tended to be lower in patients with hypokalemia, indicating that it may affect the functional prognosis in stroke [
11]. However, the functional parameters representing muscle strength and ambulatory and cognitive functions were not discussed comprehensively. Furthermore, although the serum potassium level may show day-to-day fluctuations in the clinical environment, only hypokalemia on admission was evaluated in previous studies, and the subsequent changes were not presented.
Here we investigated the effect of hypokalemia in patients with ischemic or hemorrhagic stroke using various functional scales. There is no established critical period in which the serum potassium level should be strictly controlled since the serum potassium level may fluctuate daily during the acute stage of stroke. In this study, in the hypokalemic group, we included 21 patients with hypokalemia at admission and 25 patients who developed hypokalemia within 1 week after admission. We also examined functional differences according to the elapsed time for the recovery of serum potassium level to normal but found no significant association between hypokalemia duration and functional recovery (
Fig. 2). The mean serum potassium level in the hypokalemic group was 3.30 mmol/L, indicating that the hypokalemia was not severe. This is a clinically important point because even a subtle decrement in serum potassium level could be related to a poor functional outcome in post-stroke hemiplegic patients. Thus, serum potassium level can be considered as an indicator of the prognosis of rehabilitation, and must be controlled in the acute phase.
In this study, a significant intergroup functional difference was observed in terms of ambulatory capacity and muscle strength but not cognitive function. The association between cognitive function and hypokalemia in stroke has yet to be revealed. One study compared sodium and potassium levels between mild cognitive impairment (MCI) patients and controls and reported that the higher the potassium level, the higher the MCI risk [
15]. However, this study investigated an MCI group, not a stroke group. Another study reported a negative correlation between sodium-to-potassium ratio and cognitive function; however, no significant correlation was observed between potassium or sodium level itself and cognitive function, and the study population was not a stroke group, but a general elderly population [
12]. In the present study, we observed no significant association between hypokalemia and cognitive improvement.
One study reported that motor weakness is the most common predictors of upper limb performance in subacute stroke but we found no significant intergroup difference in upper-extremity motor score [
16]. In addition to muscle strength, an investigation of dexterity, which may affect performance speed and accuracy, may be needed in an upper-limb functional assessment but was not included in this study. In addition, fine movements of the upper limb can be affected by cognitive function. In this study, no significant intergroup difference in cognition was observed, which might be another reason for the lack of a significant difference between upper-extremity function.
The meaningful risk factors in multivariate analysis for hypokalemia in post-stroke hemiplegic patients were hospitalization duration and previous use of diuretics. In patients with hypokalemia (23.9%), nine patients received hydrochlorothiazide and two received loop diuretics, and in normokalemic group (8.0%), two patients took loop diuretics and two had spironolactone. We could not reveal which diuretics were significantly associated with hypokalemia in stroke in this study, but it could be possible in future research with large population. Thus, the clinician should pay attention to the history of taking diuretics as a predisposing factor of hypokalemia in post-stroke patients who are planning to undergo a rehabilitation program.
Regarding the causes of hypokalemia, a significant correlation is already known to exist between serum potassium level and previous dietary potassium intake [
17]. Gariballa et al. [
7] suggested that hypokalemia may be related to insufficient dietary potassium intake. Subsequently, Young et al. [
18] observed that serum potassium level is highly sensitive to dietary potassium intake rate, especially if the initial potassium intake rate is low. Therefore, serum potassium level could easily change in patients with a low-potassium diet. Several studies reported that dietary potassium supplements could normalize serum potassium levels and protect against atherosclerotic lesion formation by preventing free radical formation, endothelial dysfunction, and vascular smooth muscle cell proliferation [
818-
20]. Dietary potassium intake is more important in modern society, as a refined food–based diet may have lower potassium content and potentially lead to chronic potassium deficiency. However, we did not evaluate the relationship between functional prognosis and dietary potassium intake in patients with stroke, which could be a subject of a further study.
There were several limitations in this study. We observed an intergroup difference in hospitalization duration and previous use of diuretics, which may be a limitation in the comparison of treatment effects. In this study, patients in the hypokalemic group significantly more frequently showed an in-hospital infection and surgical history, which could be the main cause of the longer hospitalization duration. However, no significant intergroup difference was seen in the baseline characteristics or intensive rehabilitation duration. Furthermore, we used delta parameters before versus after treatment; thus, the results of this study are thought to have clinical usefulness. The prescription of diuretics also could be a confounding factor in this study, due to the possible direct correlation between the diuretics and post-stroke functional recovery. A further subgroup study with larger population regarding on the diuretic use would be helpful to specifically reveal the collinearity. Furthermore, in order to exclude the possible confounding effect of hospitalization duration or diuretics, a following prospective randomized trial would be helpful in clarifying the correlation between serum hypokalemia and post-stroke functional recovery.
The retrospective design of this study may have limited the number of usable variables based on the fact that some scales were not evaluated before the initial evaluation and at discharge. The FIM total score at discharge and upper-limb dexterity could have enriched the functional assessment results in this study. The small sample size of this study can also be a limitation. Some dietary potassium intake indices, including dietary questionnaire findings and urinary potassium excretion level should be considered as important parameters to overcome the limitations of this study in future studies. Considering the dynamic alterations of the serum potassium levels in acute post-stroke phase, we included patients with hypokalemia within 1 week after admission in the hypokalemic group. However, since there is no previous consensus on the critical management period for hypokalemia in post-stroke patients, further studies are required to determine the critical management period for serum potassium level.
In conclusion, hypokalemia could be considered as one of deteriorating factors for post-stroke functional prognosis, particularly ambulatory and lower-extremity motor function. Further prospective studies with larger study populations are needed to observe the relationship with other functional parameters and determine the effect of dietary potassium supplements in the prognosis of stroke rehabilitation. A study to identify the critical management period for serum potassium level would be helpful for maximizing post-stroke function in hemiplegic post-stroke patients.