Abstract
Purpose
Materials and Methods
Results
Figures and Tables
Fig. 1
Flow chart illustrating patient selection. Among 42 patients with solid lung nodules or masses that were prospectively enrolled, 6 were excluded, resulting in a total of 36 patients with non-small cell lung cancer that were included in the study.
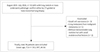
Fig. 2
Contrast-enhanced axial chest CT (A), PETRA (B), and radial VIBE (C) images from a 44-year-old woman with adenocarcinoma in right upper lobe. Arrows indicate intratumoral bubble-like lucency.

Fig. 3
Contrast-enhanced axial chest CT (A), PETRA (B), and radial VIBE (C) images from a 78-year-old man with squamous cell carcinoma in left lower lobe. In (A), the mass's interface (arrow) shows obliterated relationship with adjacent chest wall on CT. However, on MRI, the tumor interface with the pleura (arrows, B, C) is clear. This is well-recognized in (C) rather than (B). This mass underwent operation and was proven to have no parietal pleural invasion.
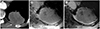
Fig. 4
Coronal reformatted images of contrast-enhanced chest CT (A), PETRA (B), and radial VIBE (C) images from a 65-year-old man with adenocarcinoma in right lower lobe base. An irregular nodule (arrow) with contact with the adjacent diaphragm is apparent in (A). However the nodule (arrows) is not apparent in (B, C).
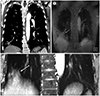
Table 1
MRI Protocol and Acquisition Time in Order of Acquisition
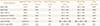
*Indicates electrocardiography-gated sequences, so acquisition times are subject to patients' RR interval.
fs = fat suppression, Gd-En = gadolinium-enhanced, PETRA = point-wise encoding time reduction with radial acquisition, T1WI = T1-weighted imaging, T2WI = T2-weighted imaging, VIBE = volumetric interpolated breath-hold examination
Table 3
Agreement between Free-Breathing PETRA and Radial VIBE Compared to CT, as κ-Values*

*Agreement was considered slight if the κ-values were below 0.20, fair if they were between 0.21–0.40, moderate if they were between 0.41–0.60, substantial if they were between 0.61–0.80, and almost perfect if they were greater than 0.81.
κ = Kappa, PETRA = pointwise encoding time reduction with radial acquisition, VIBE = volumetric interpolated breath-hold examination
Table 4
Internal Characteristics of Tumors Observed on CT, PETRA, and Radial VIBE

Internal necrosis is defined as inhomogeneous low attenuation or signal intensity without cavitation on enhanced CT or MRIs. Calcification is defined as high attenuated foci on CT and corresponding signal void on MRIs. Bubble-like lucency is defined as intratumoral air attenuation or signal intensity.
PETRA = pointwise encoding time reduction with radial acquisition, VIBE = volumetric interpolated breath-hold examination
References














