Abstract
Purpose
CD81 is a prognostic biomarker for high-grade bladder cancer (BC). In this study, we aimed to determine the functional mechanisms underlying the role of CD81 in BC progression.
Materials and Methods
In two invasive BC cell lines (T24, J82), CD81 expression was suppressed by the transfection of lentiviral vectors including CD81-specific shRNAs, and then the migration and invasion of BC cells was analyzed. Enzymatic activity of matrix metalloproteinases (MMPs) was also analyzed by collagen-zymography. The expression of MMPs was confirmed by western blotting using culture supernatants from each cell line. Signaling pathways related to MMPs were investigated using various antibodies.
Results
CD81 was successfully knocked down by shRNAs in T24 and J82 cell lines. While the migration of BC cells was not affected after the knockdown of CD81, the invasive activity was significantly increased in both cell lines. Zymography produced distinct bands using supernatants from CD81-knockdown cells, whereas only faint bands were observed with empty vector-transfected cells. We also observed an increased expression of MMPs, specifically MMP2 and 9, in the conditioned media from CD81-knockdown cells by western blotting. Mechanistically, the phosphorylation of extracellular signal-regulated kinase (ERK) was associated with the invasive activity of BC cells, while U0126 (an ERK inhibitor) reduced the invasive activity of CD81-knockdown BC cells.
Bladder cancer (BC) is the second most common cancer of the three major urological cancers (i.e., prostate, bladder, and kidney cancer), with a worldwide incidence of 541,000 in 2015, which led to 188,000 deaths [1]. Non-muscle invasive BC (NMIBC) comprises 70% of pTa, 20% of pT1, and 10% of carcinoma in situ [23], while 30% to 80% of NMIBC patients experience recurrence and 3% to 45% of NMIBC patients undergo progression to muscle invasive BC (MIBC) within five years [45]. The recurrence of NMIBC and progression to MIBC have a significant impact on patient quality of life as well as survival, thus the identification of a prognostic biomarker is an important issue. Our previous study found that CD81 was one such biomarker for high grade BC [6], and we demonstrated that an inverse correlation between membranous CD81 expression and adverse pathological characteristics existed and had prognostic value in BC.
CD81, a member of the tetraspanin family, is a cell surface protein expressed in most tissues and tumor cells [7]. It plays an important role in cell organization, protein trafficking, cellular fusion, and cell-cell interactions, and is associated with cell signaling molecules such as protein kinase C, extracellular signal-regulated kinase (ERK), mitogen-activated protein kinase (MAPK), Ras, and Rac [8]. CD81 is also involved in B and T cell activation in the immune system and affects tumor growth and metastasis through the regulation of T regulatory cell function [9101112]. In several cancers such as breast and lung cancer, hepatocellular carcinoma, and melanoma, CD81 expression was reported to be associated with cancer progression [1314151617]. However, in other cancers (e.g., stomach cancer and multiple myeloma), CD81 expression was found to inhibit tumor progression [1819]. As such, the role of CD81 in cancer progression may be cancer type-dependent. In any case, the CD81 mechanism of action in different cancers remains unclear.
Although CD81 has been identified as a biomarker of BC in a limited number of studies [620], no study to date has evaluated a functional mechanism for CD81 in BC. In this study, we sought to investigate the functional mechanism of CD81 in BC progression through in vitro modulation of CD81 expression in BC cells.
The study protocol was approved by the Institutional Review Board of the Eulji University Hospital (approval number: 2018-07-010).
BC cell lines (T24, J82) were obtained from the Korean Cell Line Bank. T24 and J82 cells were cultured in Dulbecco's Modified Eagle's medium (DMEM; Corning Life Science, Corning, NY, USA) and RPMI 1640 medium (Lonza, Allendale, NJ, USA) supplemented with 10% fetal bovine serum (FBS; Hyclone, Logan, UT, USA), respectively. The cells were maintained in a humidified atmosphere of 5% CO2 at 37℃.
Plasmids containing shRNA for human CD81 (TRCN0000382433 and TRCN0000300293; Sigma, St. Louis, MO, USA), scramble plasmid for shRNA (#1864; Addgene, Cambridge, MA, USA), and CD81 overexpression vector (pCDH; SBI, Palo Alto, CA, USA) were co-transfected with pPACKF1 packaging plasmid mix (SBI) into Lenti-X 293T cells (Takara, Otsu, Japan) using X-tremeGENE HP DNA transfection reagent (Sigma) according to the manufacturer's protocol. BC cells were infected with viral supernatants from 293T cells along with polybrene (5 µg/mL) for 24 hours. After 10 days of selection with puromycin (0.5 µg/mL), an efficiency of CD81 knockdown was evaluated by flow cytometry and western blotting.
BC cells were resuspended in 1% of FBS/phosphatebuffered saline (PBS) (v/v) and stained with anti-CD81 antibody (Santa Cruz Biotechnology, Dallas, TX, USA) at 4℃ for 30 minutes. For labeling, allophycocyanin-conjugated anti-mouse IgG (R&D systems, Minneapolis, MN, USA) was used as a secondary antibody. After washing with PBS, the cells were analyzed with a Guava easyCyte Flow Cytometer (Merck Millipore, Bedford, MA, USA) and InCyte 3.1 software (Merck Millipore).
BC cell proteins were isolated using a 1X sodium dodecyl sulfate (SDS) buffer containing 62.5 mM Tris-HCL at pH 6.8, 2% (w/v) SDS, 10% glycerol, 50 mM dithiothreitol, and 0.01% (w/v) bromophenol blue. The cell suspension was boiled for 10 minutes and centrifuged at 13,000×g for 10 minutes. The proteins were resolved by electrophoresis in a 10% SDS polyacrylamide gel and transferred to a nitrocellulose blotting membrane (GE Healthcare, Little Chalfont, UK). The membrane was blocked with 5% skim milk. Mouse polyclonal anti-CD81 antibody (Santa Cruz Biotechnology), rabbit anti-Akt antibody (Bioss Antibodies Inc., Woburn, MA, USA), rabbit anti-phospho-Akt antibody (Cell Signaling Technology, Beverly, CA, USA), mouse anti-ERK antibody (Bioss Antibodies Inc.), anti-phospho-ERK (Bioss Antibodies Inc.), rabbit anti-p38 antibody (Bioss Antibodies Inc.), rabbit anti-c-Jun N-terminal kinases (JNK) antibody (Bioss Antibodies Inc.), anti-phospho-JNK (Santa Cruz Biotechnology), rabbit anti-glyceraldehyde 3-phosphate dehydrogenase (GAPDH) (Cusabio, Houston, TX, USA), rabbit anti-MMP2 antibody (Bioss Antibodies Inc.), rabbit anti-MMP9 antibody (Bioss Antibodies Inc.) were used as primary antibodies. Horseradish peroxidase-conjugated anti-rabbit or anti-mouse antibodies (Bethyl Laboratories Inc., Montgomery, TX, USA) were used as secondary antibodies. The results were visualized with enhanced chemiluminescence detection reagent (Bio-Rad, Hercules, CA, USA) and a ChemiDoc Touch Imaging System (Bio-Rad).
The membrane within the insert (BD Biosciences, San Jose, CA, USA) was coated with matrigel. BC cells, at 4×104 cells/well, were introduced into the cell culture insert and incubated at 37℃ for 18 hours. After incubation, the membrane was stained with crystal violet.
Cell proliferation was measured by MTT assay. About 1×104 cells/well were seeded into 96-well plates and incubated for 1 hour or 72 hours, the cells were incubated with 0.5 mg/mL of thiazolyl blue tetrazolium bromide (MTT) solution at 37℃ for 4 hours. The solution was replaced with dimethylsulfoxide (DMSO), and the optical densities were determined at 540 nm and 630 nm using a microplate reader (Molecular Devices, San Jose, CA, USA). The data are representative of three independent experiments performed in triplicate.
For the zymographic assay, cells were cultured in DMEM without FBS for 48 hours. Conditioned media (CM) was collected and centrifuged at 2,000×g for 10 minutes. CM was concentrated with an Amicon Ultra-2 Centrifugal Filter Unit (Merck Millipore) and the concentrated CM was normalized for cell number. Proteins in the normalized CM were then separated by electrophoresis in a 10% polyacrylamide gel impregnated with 2 mg/mL gelatin (Sigma). After electrophoresis, the gels were washed in 2.5% Triton X-100 for 30 minutes and then incubated in zymogram development solution buffer (50 mm Tris-HCl, 5 mM CaCl2 dissolved in distilled water) for 24 hours at 37℃. This buffer was changed every 4 hours, and stained with 0.2% Coomassie Blue.
Knockdown of CD81 in BC cells Given that decreased CD81 expression was associated with BC invasion in our prior study [6], we tried to suppress the expression of CD81 in two invasive BC cell lines (T24, J82) using CD81-specific shRNAs. Empty vector was used as a control and two shRNAs against a different region of CD81 was applied to each cell line. After 10 days of culture of the shRNA-transfected cells with selective antibiotics, the expression of CD81 was analyzed (Fig. 1A). Following flow cytometry, an obvious suppression of CD81 expression was observed in both cell lines with shRNAs as compared to the control (Fig. 1A). Western blot analyses were consistent with these results (Fig. 1B).
To assess the effect of CD81 knockdown on the migration and invasion of BC cells, transwell migration and invasion assays were performed. Migration increased slightly in CD81-knockdown cells as compared to control cells, but the difference was not significant (Fig. 2A). However, the invasive activity of CD81-knockdown cells was significantly increased in both T24 and J82 cells as compared with the control (Fig. 2B), indicating that CD81 was associated with the invasive activity of BC cells. To analyze the correlation between cell proliferation and invasive activity, proliferation of CD81-knockdown cells was analyzed by MTT assay after 72 hours of culture (Fig. 2C, D). While proliferation of T24 cells was decreased by CD81 knockdown, CD81-knockdown J82 cells showed slightly increased proliferation rate. These findings indicated that CD81 knockdown did not have consistent effects on BC cell proliferation and there was no correlation between cell proliferation and invasive activity.
To elucidate the relevant cause of the increased invasive activity of CD81-knockdown BC cells, the enzymatic activity and expression of MMPs was analyzed by zymography and western blotting. Obvious bands of MMPs were observed in our zymogram (Fig. 3A) with supernatants from CD81-knockdown cells, whereas only faint bands were observed with empty vector-transfected cells. Specifically, the CD81-knockdown T24 cells had a higher expression of MMP2 than control cells, while CD81-knockdown J82 cells showed a higher expression of MMP9 than control cells (Fig. 3A). We confirmed the increased expression of MMP2 and 9 in the CM from CD81-knockdown T24 and J82 cells, respectively, by western blotting (Fig. 3B).
To identify signaling proteins involved in increased MMP expression in CD81-knockdown BC cells, the expression of various proteins in MMP2/9-associated signaling pathways was analyzed by western blot. Among the examined proteins, only phosphorylated ERK (p-ERK) was increased in both CD81-knockdown T24 (Fig. 4A) and J82 cells (Fig. 4B), compared with control, indicating that increased p-ERK in the MAPK pathway may lead to increased expression of MMP2/9.
In order to examine the effect of inhibition of ERK phosphorylation on the invasive activity of CD81-knockdown BC cells, T24 and J82 cells were treated with U0126 (ERK inhibitor) or DMSO (control). ERK phosphorylation and the invasive activity of BC cells were analyzed by western blot and invasion assay, respectively. ERK phosphorylation was successfully inhibited by a non-toxic level of U0126 (10 µM) in both T24 and J82 cells (Fig. 5A). While invasive activity was increased in CD81-knockdown cells, treatment with U0126 suppressed the invasion of CD81-knockdown cells to a level similar to those of control cells (Fig. 5B). These findings suggest that the invasive activity of CD81-knockdown BC cells is mediated by ERK phosphorylation.
To assess the effect of CD81 overexpression on the invasive property of BC cells, we overexpressed CD81 using a lentivirus system. BC cells were infected with lentivirus containing control vectors or CD81 overexpression vectors, and then CD81 expression was analyzed by western blot (Fig. 6). While both T24 and J82 cells showed overexpression of CD81 by lentivirus infection, the expression of p-ERK was unaffected. These results suggest that a basal level of CD81 in BC cells might be sufficient to suppress the phosphorylation of ERK.
Previous studies have shown conflicting results regarding the function of CD81 in cancer progression [16171819], and the functional mechanism of CD81 in BC has not been investigated. Our study was the first to identify the functional mechanism of CD81 in BC progression.
We found that the suppression of CD81 in BC cells promoted BC cell invasion, but did not significantly affect cell migration. As alluded to above, previous studies in several cancers have reported conflicting results with regard to the effect of CD81 expression on cancer cell migration and invasion. For instance, in a study of multiple myeloma, overexpression of CD81/82 resulted in decreased cell migration and invasion potential [18]. In contrast, a study of melanoma showed that CD81 overexpression resulted in increased cell migration and invasion [16]. Similar to melanoma, overexpression of CD81 in breast cancer increased cell migration [17]. Given these previous studies in conjunction with our current study, it is apparent that the effect of CD81 on cancer cell migration and invasion is dependent upon the cancer type.
Our data also show that the suppression of CD81 promotes the expression of MMPs, specifically MMP2 and MMP9. MMPs are zinc-containing endopeptidases expressed in connective tissue and inflammatory cells, which are regulated by hormones, growth factors and cytokines. MMPs play an important role in tissue remodeling in a variety of physiological or pathological processes (e.g., bone morphogenesis, the menstrual cycle, development, arthritis, atherosclerosis, and cancer invasion) [2122]. Upregulation of MMPs has been identified to promote cancer progression by promoting cancer cell growth, migration, invasion, and metastasis in almost every type of human cancer [23]. In particular, upregulation of MMP2 and MMP9 in BC is known to be associated with cancer progression and poor survival, which is consistent with our findings [242526]. Of note, though MMPs promote the progression of most cancers, differences exist in the association between CD81 expression and MMPs depending on the cancer type. In line with our BC results, CD81/82 overexpression in multiple myeloma reduced MMP9 secretion, and attenuated cell adherence, motility, and invasive potential [18]. However, in a study of breast cancer, knocking down two or three of the tetraspanin proteins (CD9, CD81, TSPAN2) reduced cancer cell invasion and growth by decreasing the function of membrane type 1(MT1)-MMP [15]. Moreover, a melanoma study has also shown that CD81 induces MT1-MMP expression, leading to the migration, invasion, and metastasis of cancer cells [16].
The conflicting results on the role of CD81 in cancer progression [15161718] indicate that its mechanism of action is mediated by MMP through other factors that are downstream of CD81. As a potential downstream mediator of CD81, we found that p-ERK in the MAPK pathway is involved in BC tumor invasion. Specifically, increased p-ERK following CD81 suppression, induced the increased expression of MMP2/9, and consequently resulted in increased BC cell invasion.
ERK is a signaling substance that plays an important role in cell proliferation, survival, migration, differentiation, and apoptosis via a related pathway (e.g., the MAPK pathway) in various cancers [27]. Prior studies have suggested possible mediators of CD81 signaling in several cancers. In liver tumor cells, CD81 leads to cell proliferation and activation of ERK/MAPK, which is involved in Shc [14]. A study of melanoma showed that CD81 induces MT1-MMP expression through Akt-dependent Sp1 activation [16]. Moreover, in gastric cancer, the suppression of CD81 may provide benefits to tumor cell growth and survival through p38 phosphorylation [19]. While these other studies have suggested other mediators of CD81 such as Shc, Akt-dependent Sp1 and p38, we found that ERK phosphorylation plays a role in BC progression through increased MMP expression after CD81 suppression. Our finding that treatment with the ERK phosphorylation inhibitor, U0126, suppressed the invasion of CD81-knockdown cells (Fig. 5) supports the role of p-ERK in BC. The different mediators of CD81 might be responsible for the conflicting results regarding the role of CD81 in cancer progression in different cancers. Further studies are needed to elucidate the exact mechanisms behind the varied roles of CD81 among different cancers, focusing on the factors that work downstream of membranous CD81.
We demonstrated that CD81 suppression promotes the invasion of BC cells by increased MMP expression via ERK phosphorylation. Further studies are needed to elucidate the signaling mediators between membranous CD81 and p-ERK expression. Our results suggest that there may be some therapeutic benefit in regulating CD81 by modulating ERK phosphorylation in BC.
ACKNOWLEDGMENTS
This research was supported by EMBRI Grants 2018-DJ-0001 from the Eulji University and a National Research Foundation of Korea (NRF) Grant funded by the Korean Government (MSIP) (No. 2017031765).
References
1. Global Burden of Disease Cancer Collaboration. Fitzmaurice C, Allen C, Barber RM, Barregard L, Bhutta ZA, et al. Global, regional, and national cancer incidence, mortality, years of life lost, years lived with disability, and disability-adjusted life-years for 32 cancer groups, 1990 to 2015: a systematic analysis for the Global Burden of Disease Study. JAMA Oncol. 2017; 3:524–548. PMID: 27918777.
2. van Rhijn BW, Burger M, Lotan Y, Solsona E, Stief CG, Sylvester RJ, et al. Recurrence and progression of disease in non-muscle-invasive bladder cancer: from epidemiology to treatment strategy. Eur Urol. 2009; 56:430–442. PMID: 19576682.


3. Miyake M, Fujimoto K, Hirao Y. Active surveillance for non-muscle invasive bladder cancer. Investig Clin Urol. 2016; 57 Suppl 1:S4–S13.


4. Hall MC, Chang SS, Dalbagni G, Pruthi RS, Seigne JD, Skinner EC, et al. Guideline for the management of nonmuscle invasive bladder cancer (stages Ta, T1, and Tis): 2007 update. J Urol. 2007; 178:2314–2330. PMID: 17993339.


5. Babjuk M, Oosterlinck W, Sylvester R, Kaasinen E, Böhle A, Palou-Redorta J. European Association of Urology (EAU). EAU guidelines on non-muscle-invasive urothelial carcinoma of the bladder. Eur Urol. 2008; 54:303–314. PMID: 18468779.


6. Lee MS, Kim JH, Lee JS, Yun SJ, Kim WJ, Ahn H, et al. Prognostic significance of CREB-Binding protein and CD81 expression in primary high grade non-muscle invasive bladder cancer: identification of novel biomarkers for bladder cancer using antibody microarray. PLoS One. 2015; 10:e0125405. PMID: 25915404.


7. Levy S, Todd SC, Maecker HT. CD81 (TAPA-1): a molecule involved in signal transduction and cell adhesion in the immune system. Annu Rev Immunol. 1998; 16:89–109. PMID: 9597125.


8. Vences-Catalán F, Duault C, Kuo CC, Rajapaksa R, Levy R, Levy S. CD81 as a tumor target. Biochem Soc Trans. 2017; 45:531–535. PMID: 28408492.


9. Vences-Catalán F, Rajapaksa R, Srivastava MK, Marabelle A, Kuo CC, Levy R, et al. Tetraspanin CD81 promotes tumor growth and metastasis by modulating the functions of T regulatory and myeloid-derived suppressor cells. Cancer Res. 2015; 75:4517–4526. PMID: 26329536.


10. Vences-Catalán F, Rajapaksa R, Srivastava MK, Marabelle A, Kuo CC, Levy R, et al. Tetraspanin CD81, a modulator of immune suppression in cancer and metastasis. Oncoimmunology. 2015; 5:e1120399. PMID: 27467918.


11. Levy S, Shoham T. The tetraspanin web modulates immune-signalling complexes. Nat Rev Immunol. 2005; 5:136–148. PMID: 15688041.


12. Mittelbrunn M, Yáñez-Mó M, Sancho D, Ursa A, Sánchez-Madrid F. Cutting edge: dynamic redistribution of tetraspanin CD81 at the central zone of the immune synapse in both T lymphocytes and APC. J Immunol. 2002; 169:6691–6695. PMID: 12471100.


13. Mazzocca A, Liotta F, Carloni V. Tetraspanin CD81-regulated cell motility plays a critical role in intrahepatic metastasis of hepatocellular carcinoma. Gastroenterology. 2008; 135:244–256.e1. PMID: 18466772.


14. Carloni V, Mazzocca A, Ravichandran KS. Tetraspanin CD81 is linked to ERK/MAPKinase signaling by Shc in liver tumor cells. Oncogene. 2004; 23:1566–1574. PMID: 14676841.


15. Lafleur MA, Xu D, Hemler ME. Tetraspanin proteins regulate membrane type-1 matrix metalloproteinase-dependent pericellular proteolysis. Mol Biol Cell. 2009; 20:2030–2040. PMID: 19211836.


16. Hong IK, Byun HJ, Lee J, Jin YJ, Wang SJ, Jeoung DI, et al. The tetraspanin CD81 protein increases melanoma cell motility by up-regulating metalloproteinase MT1-MMP expression through the pro-oncogenic Akt-dependent Sp1 activation signaling pathways. J Biol Chem. 2014; 289:15691–15704. PMID: 24733393.


17. Zhang N, Zuo L, Zheng H, Li G, Hu X. Increased expression of CD81 in breast cancer tissue is associated with reduced patient prognosis and increased cell migration and proliferation in MDA-MB-231 and MDA-MB-435S human breast cancer cell lines in vitro. Med Sci Monit. 2018; 24:5739–5747. PMID: 30117494.


18. Tohami T, Drucker L, Shapiro H, Radnay J, Lishner M. Overexpression of tetraspanins affects multiple myeloma cell survival and invasive potential. FASEB J. 2007; 21:691–699. PMID: 17210782.


19. Yoo TH, Ryu BK, Lee MG, Chi SG. CD81 is a candidate tumor suppressor gene in human gastric cancer. Cell Oncol (Dordr). 2013; 36:141–153. PMID: 23264205.


20. White A, Lamb PW, Barrett JC. Frequent downregulation of the KAI1(CD82) metastasis suppressor protein in human cancer cell lines. Oncogene. 1998; 16:3143–3149. PMID: 9671393.


21. Van Lint P, Libert C. Chemokine and cytokine processing by matrix metalloproteinases and its effect on leukocyte migration and inflammation. J Leukoc Biol. 2007; 82:1375–1381. PMID: 17709402.


22. Verma RP, Hansch C. Matrix metalloproteinases (MMPs): chemical-biological functions and (Q)SARs. Bioorg Med Chem. 2007; 15:2223–2268. PMID: 17275314.


23. Egeblad M, Werb Z. New functions for the matrix metalloproteinases in cancer progression. Nat Rev Cancer. 2002; 2:161–174. PMID: 11990853.


24. Davies B, Waxman J, Wasan H, Abel P, Williams G, Krausz T, et al. Levels of matrix metalloproteases in bladder cancer correlate with tumor grade and invasion. Cancer Res. 1993; 53:5365–5369. PMID: 8221672.
25. Kanayama H, Yokota K, Kurokawa Y, Murakami Y, Nishitani M, Kagawa S. Prognostic values of matrix metalloproteinase-2 and tissue inhibitor of metalloproteinase-2 expression in bladder cancer. Cancer. 1998; 82:1359–1366. PMID: 9529029.


26. Durkan GC, Nutt JE, Marsh C, Rajjayabun PH, Robinson MC, Neal DE, et al. Alteration in urinary matrix metalloproteinase-9 to tissue inhibitor of metalloproteinase-1 ratio predicts recurrence in nonmuscle-invasive bladder cancer. Clin Cancer Res. 2003; 9:2576–2582. PMID: 12855633.
27. Sun Y, Liu WZ, Liu T, Feng X, Yang N, Zhou HF. Signaling pathway of MAPK/ERK in cell proliferation, differentiation, migration, senescence and apoptosis. J Recept Signal Transduct Res. 2015; 35:600–604. PMID: 26096166.


Fig. 1
Knockdown of CD81 in bladder cancer cell lines by shRNA. CD81 shRNAs were transduced into human bladder cancer cell lines, T24 and J82, by lentivirus infection. Virus-infected cells were selected with puromycin for two weeks and the expression of CD81 was analyzed by flow cytometry (A) and western blots (B).
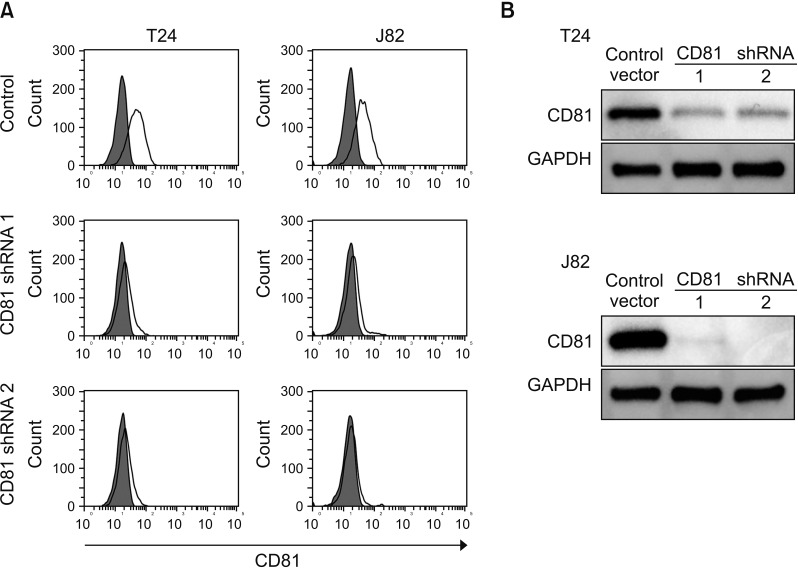
Fig. 2
Migration, invasive activity, and proliferation of CD81-knockdown bladder cancer cells. CD81-knockdown T24 and J82 cells were applied to transwell assays with and without matrigel coating to analyze migration (A), invasive activity (B), and cell proliferation (C, D). (A, B) After an overnight incubation, migrated or invasive cells were stained and analyzed (stained with crystal violet, ×400). (C, D) The proliferation of CD81-knockdown T24 (C) and J82 (D) cells was analyzed by MTT assay after 72 hours of culture. Empty vector-transfected cells were used as control. Data are shown as the mean±standard deviation. Cells were counted from three random fields. ns, not significant. *p<0.05, **p<0.01.
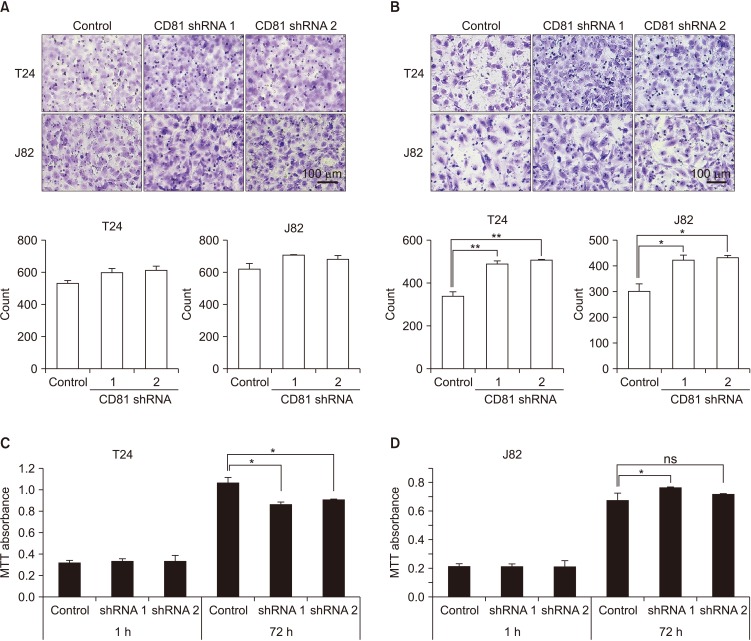
Fig. 3
Increased expression and activity of MMP2/9 in CD81-knockdown bladder cancer cells. (A) Zymography for MMP activity. (B) Western blot analysis of MMP2 and MMP9. The culture supernatant was concentrated by an Amicon Ultra-2 Centrifugal Filter and MMP2/9 expression was analyzed using each specific antibody.
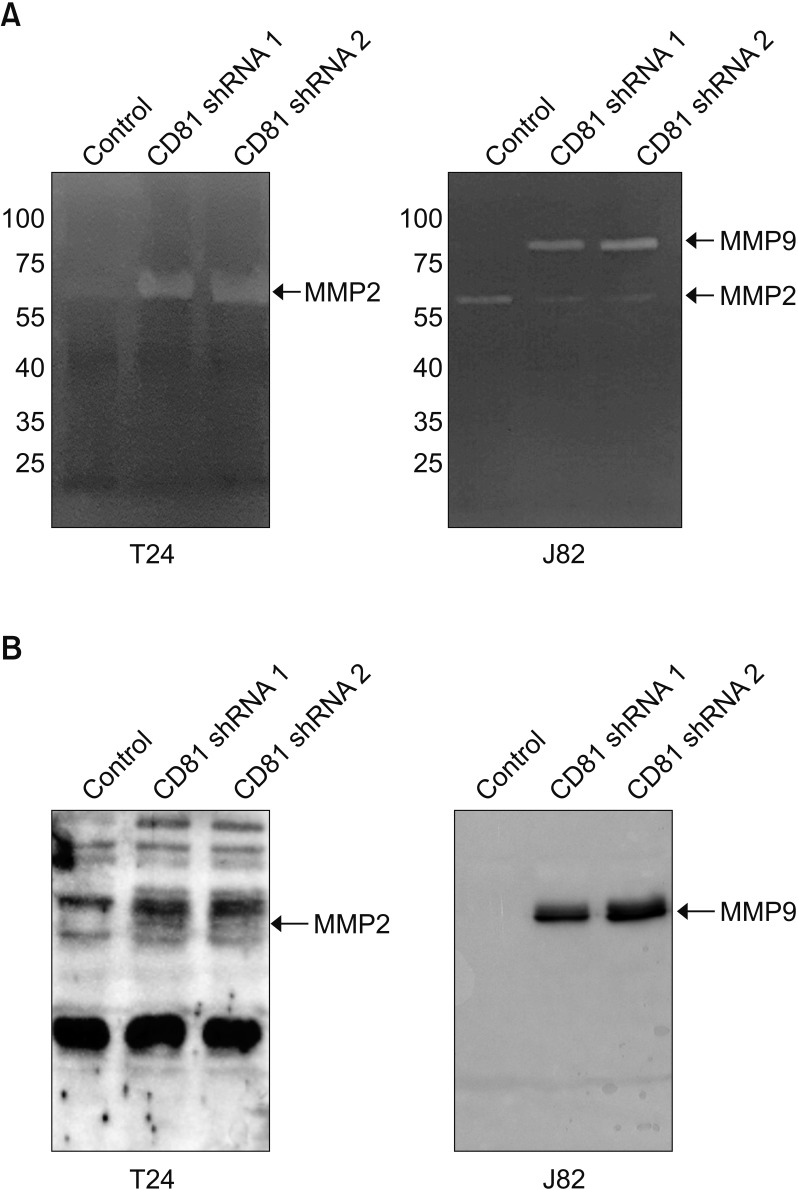
Fig. 4
Screening for MMP2/9-associated signaling pathways in CD81-knockdown bladder cancer cells. MMP2/9-associated signaling pathways were analyzed in CD81-knockdown T24 (A) and J82 (B) bladder cancer cell lines. Glyceraldehyde 3-phosphate dehydrogenase (GAPDH) was used as a housekeeping control. p-Akt, phospho-Akt; ERK, extracellular signal-regulated kinase; p-ERK, phospho-ERK; JNK, c-Jun N-terminal kinases; p-JNK, phospho-JNK.
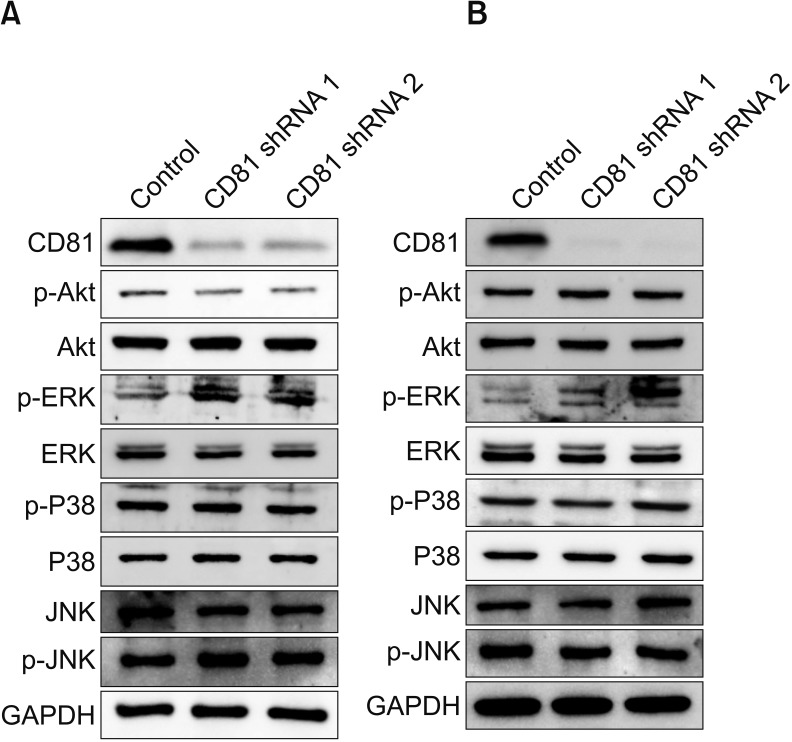
Fig. 5
Inhibition of extracellular signal-regulated kinase (ERK) phosphorylation suppressed the invasive activity of CD81-knockdown bladder cancer cells. The ERK inhibitor, U0126, was administered to T24 and J82 cell lines. (A) Inhibition of ERK phosphorylation by U0126 in T24 and J82 bladder cancer cells. (B) Invasive activity of U0126-treated T24 and J82 bladder cancer cells (stained with crystal violet, ×400). DMSO, dimethylsulfoxide; GAPDH, glyceraldehyde 3-phosphate dehydrogenase.
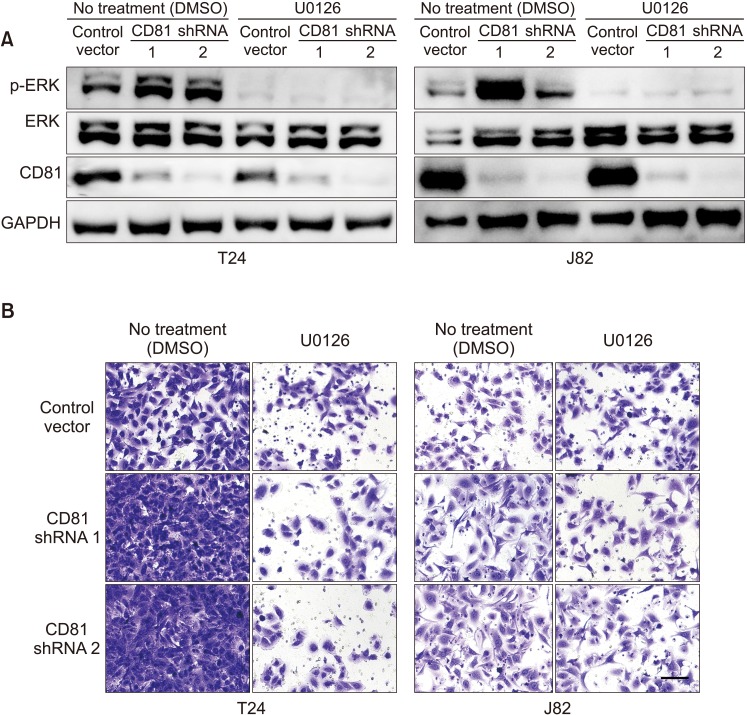
Fig. 6
Overexpression of CD81 did not induce extracellular signal-regulated kinase (ERK) phosphorylation. CD81 was overexpressed in T24 and J82 bladder cancer cells using lentiviral vectors. CD81 expression and ERK phosphorylation were analyzed by western blot analysis. Ctl, control; p-ERK, phospho-ERK; GAPDH, glyceraldehyde 3-phosphate dehydrogenase.
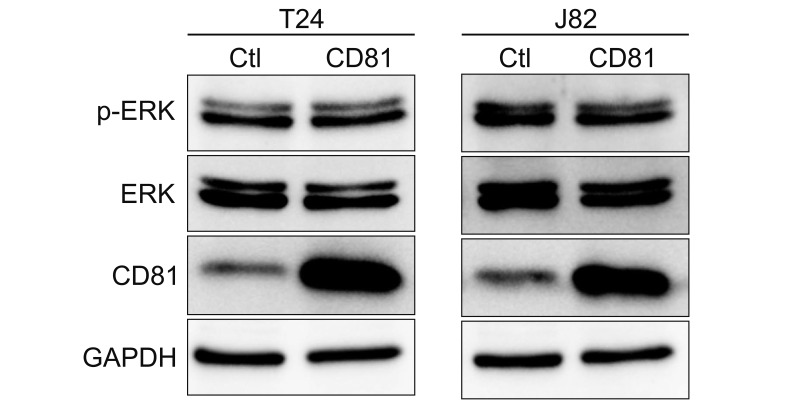