Abstract
PURPOSE
The aim of this study was to investigate simulated localized and generalized wear of indirect composite resins used for implant supported provisional restorations.
MATERIALS AND METHODS
The study investigated ten indirect composite resins. Two kinds of wear were simulated by 400,000 cycles in a Leinfelder-Suzuki (Alabama) machine. Localized wear was simulated with a stainless-steel ball bearing antagonist and generalized with a flat-ended stainless-steel cylinder antagonist. The tests were carried out in water slurry of polymethyl methacrylate beads. Wear was measured using a Proscan 2100 noncontact profilometer in conjunction with Proscan and AnSur 3D software.
RESULTS
Both localized and generalized wear were significantly different (P<.05) among the indirect composite resins. SR Nexco and Gradia Plus showed significantly less wear than the other indirect composite resins. The rank order of wear was same in both types of wear simulation.
CONCLUSION
Indirect composite resins are recommended when a provisional implant-supported restoration is required to function in place over a long period. Although only some indirect composite resins showed similar wear resistance to CAD/CAM composite resins, the wear resistance of all the indirect composite resins was higher than that of bis-acryl base provisional and polymethyl methacrylate resins.
Implant-supported restorations are documented to have predictable results and are currently considered a suitable way to replace missing or failing teeth.12 Success with current implant dentistry depends on management of hard and soft tissue, and on the achievement of optimal aesthetics.34 Thus, the state of the art trend is to place implants and immediately add long-term provisional restorations.56 The importance of such restorations is particularly clear in long-term provisionalization.78 The restorations represent an important step in determining the final shape and design, dependent on the osseointegration between bone and implant, and maturation of the soft tissues in the peri-implant area.9
Although an implant-supported provisional restoration is generally in place for at least 3 months when a single tooth is being replaced, if a fixed partial denture is being placed, this period is longer.10 Provisional implant-supported restorations may be in place for one or two years for long span fixed partial dentures and full-arch reconstruction and the necessary evaluation period may extend this even further.11 To achieve predictability for implant, provisional restorative materials should ideally possess suitable mechanical and physical properties, such as high fracture toughness, improved occlusal wear resistance, and high flexural properties.12
However, these restorations are known to sometimes fail after prolonged use, and often show occlusal wear.12 This may require repair, which would ideally be avoided in a provisional restoration. Various approaches have been proposed for the fabrication of implant-supported provisional restorations.13 The restoration can be made chair-side or through laboratory fabrication. Two major groups of resins are found in chair-side materials: 1) methacrylates (methyl methacrylate and ethyl methacrylate) and 2) bis-acryl resin materials.14 When the restorations are prepared in a laboratory, indirect composite resins or CAD/CAM composite resins are typically used.15
Takamizawa et al.16 investigated the simulated generalized wear of chair-side provisional restorative materials and reported that bis-acryl provisional resins demonstrated significantly better resistance to wear than conventional methacrylate resins. A previous study8 connected to this topic concluded that the use of indirect composite resin is preferable to that of chair side provisional materials when the implant-supported provisional restoration has to be in use for a prolonged period. However, the wear characteristics of the indirect composite resins used for the provisional restorations placed for implant therapy have not been investigated.
The purpose of this study was to investigate simulated localized and generalized wear in indirect composite resins used to make implant supported provisional restorations. The two null hypotheses tested were: 1) there are no differences between the two types (localized and generalized) of simulated wear of indirect composite resins for implant supported provisional restorations; 2) simulated localized and generalized wear is not affected by the type of indirect composite resin.
Ten indirect composite resins were used in this laboratory study: 1) Ceramage (CM, Shofu), 2) Experia (EX, GC), 3) Gradia (GD, GC), 4) Gradia Forte (GF, GC), 5) Gradia Plus (GP, GC), 6) Signum ceramis (SC, Heraus Kulzer), 7) Solidex (SO, Shofu), 8) Solidex Hardura (SH, Shofu), 9) SR Nexco paste (SN, Ivoclar Vivadent) and 10) Symphony (SY, 3M Oral Care). Further information about each indirect resin composite is shown in Table 1.
Ten specimens of each of the ten composite resins were fabricated for each of the two types of simulated wear: localized and generalized. Brass holders for specimens with a cylindrical cavity (6.5 mm diameter, 4.0 mm depth) were specially prepared for localized wear testing. Stainless steel holders with a cylindrical cavity (4.5 mm diameter, 4.0 mm depth) were prepared for generalized wear testing. Two increments (each approximately 2.0 mm thick) of the composite resins were inserted in the cavities and photopolymerized following the manufacturers' instructions. All of the surfaces of the composite resin were standardized 24 hours after photopolymerization by wet-polishing using a succession of silicon carbide papers (Struers) until 4,000-grit surface was obtained.
All wear simulations were conducted in a Leinfelder-Suzuki (Alabama) machine (Fig. 1). This machine has four stations in a plastic water bath where the wear holders described above were fixed. A brass ring was then placed to enclose each holder to create a reservoir in which the abrasive media (a mix of polymethyl methacrylate [PMMA] beads with a typical size of around 40 µm and water) would completely cover the surface of the composite resin in the holders. The layer of PMMA beads inside the reservoirs was about 6 mm deep.
A stainless steel ball bearing (approximately 2.4 mm radius) mounted inside a spring-loaded piston assembly simulated localized wear. Generalized wear was simulated with a cylinder (approximately 3.2 mm radius) of stainless steel with a flat tip, also on a spring-loaded piston assembly. Antagonists turned about 30° during the application of the force (peak load of (78.5 N) and then counter-turned to the starting position as the piston was retracted to complete each cycle (2 Hz). All tests were conducted with 400,000 cycles.
Each wet-polished specimen was profiled using a Proscan 2100 non-contact optical profilometer (Scantron Industrial Products) before wear simulation as a baseline. The 3D contours generated were compared to those generated after wear simulation.
After completion of 400,000 wear cycles, the specimens were ultrasonically cleaned (L&R T-14B solid state ultrasonic cleaner, L&R Manufacturing Company) for three minutes in distilled water to remove any debris. Then, the specimens were profiled for a second time with the Proscan 2100 unit using the exact instrument settings used before. The three-dimensional coordinates of both scans were transferred from the Proscan software to AnSur 3D software (Minnesota Dental Research Center for Biomaterials and Biomechanics [MDRCBB]) for analysis.
The differences between the data sets were compared to calculate facet depth and volume loss measurements. AnSur 3D was used to fit the pairs of scans to calculate volume loss (VL, mm3) for both types of wear simulation for each of the ten composite resins. Localized wear specimens were further analyzed by calculating maximum depth of wear facets (MXD, µm) and generalized wear specimens were further analyzed by calculating mean depth of wear facets (MD, µm).
Table top scanning electron microscopy (SEM; TM3000, Hitachi-High Technology) was used to observe the structure of randomly selected localized wear facets. A thin coating of gold-palladium alloy was applied in a sputter coater (Emitech SC7620 Mini Sputter Coater, Quorum Technologies) to prevent charging. The observations were done at an operating voltage of 15 kV at 500× and 5000×.
VL and MXD (for localized wear) and VL and MD (for generalized wear) were analyzed using a one-way analysis of variance (ANOVA) and Tukey's post-hoc tests (SPSS Statistics Base, IBM) with significance level of α=.05.
The results for the simulated localized wear of indirect composite resins are shown in Table 2. The VL, ranging from 0.018 to 0.194 mm3, and MXD, ranging from 72.2 to 230.8 µm, differed (P<.05) for different materials. The rank order of VL and MXD was SN-GP-GF-EX-SY-GD-SHSL-CS-CM.
Table 3 shows the results for simulated generalized wear. The VL, ranging from 0.129 to 0.608 mm3, and MD, ranging from 8.8 to 47.5 µm, of wear facets differed (P<.05) for different materials. The rank order of VL and MD was SN-GP-GF-EX-SY-GD-SH-SL-CS-CM.
Figure 2 shows representative SEM images of wear facets after simulated localized wear. High magnification SEM images exhibited filler particle plucking and cracking. Lowmagnification SEM images clearly showed that there were differences in size and shape of fillers in the indirect composite resins. SEM images of CM showed small (< 1 to 5 µm) spherical particles. SEM of EX showed irregular particles with a broad size range (< 1 – 60 µm). SEM images of GD and GF were quite similar and showed relatively large (30 – 45 µm) and small (< 1 – 4 µm) irregular particles. SEM images of GP showed relatively large (40 – 50 µm) and small (1 – 3 µm) irregular particles. SEM images of SC showed small (< 1 to 8 µm) irregular particles. SEM images of SO and SH were quite similar to SC and showed small (< 1 – 2 µm) spherical particles and wide size range of relatively large (20 – 80 µm) irregular particles. SEM images of SN showed small (< 1 – 2 µm) particles with wide size range (20 – 70 µm) of irregular shapes. SEM images of SY showed small (< 1 – 4 µm) particles of irregular shapes. The rank order of average filler size was SY-CM-SC-GD-GF-GP-EX-SN-SH-SO.
It is well-known that the wear of restorative materials in the mouth is influenced by many factors, such as contacts with antagonist teeth (attrition), food mastication and the use of toothpaste (abrasion), attack by acids in certain beverages and fruits, vomiting, inhaling industrial acids, and chemical effects (corrosion).17 There is no perfect method of simulated wear testing for restorative materials due to the complexity of the wear phenomenon, but the International Organization for Standardization (ISO) published “Dental materials - Guidance on testing of wear -” (2001) outlining guidance for 8 different wear simulations to measure the wear resistance of restorative materials in two- and three-body wear tests.18 The Alabama method was among the methods identified by the ISO publication. This approach was developed by Leinfelder and Suzuki at the University of Alabama using a polyacetal antagonist19 and then further developed by Barkmeier et al.20 at Creighton University School of Dentistry (CU) to allow the simulation of both localized and generalized wear using stainless steel antagonists. Localized wear is generated by attrition, while generalized wear is generated by abrasion. These methods were used to measure simulated localized and generalized wear of indirect composite resins for implant supported provisional restorations in this study.
In this study, the wear of the indirect composite resins ranged from 0.018 to 0.194 mm3 for VL and 72.2 to 230.8 µm for MXD with localized wear, and 0.129 to 0.608 mm3 for VL and 8.8 to 47.5 µm for MD with generalized wear. The VL, MXD, and MD measured in the two wear simulations were all material dependent. Thus, both null hypotheses, that there are no differences between the localized and generalized wear of indirect composite resins for implant supported provisional restorations and that neither type of wear is influenced by the type of indirect composite resins, were rejected.
The filler particle size has previously been found to affect the wear resistance of composite resins, through an influence on the friction coefficient and the surface roughness, which are determining factors for the wear rate of resin composites.21 However, in this study, there was a clear relationship between wear resistance and average filler size of indirect composite resins. The complexity of wear resistance is further pointed out by another study22 in which newly developed conventional composite resins showed variations with same wear simulation that could not be explained purely in terms of filler particle size.
Tsujimoto et al.23 used the same wear apparatus and methods to study simulated localized wear of CAD/CAM composite resins over 400,000 cycles and reported that this wear ranged from 0.019 to 0.035 mm3 for VL and 69.2 to 133.7 µm for MXD. Comparing these results to those of the present study, the simulated localized wear of GF, GP, and SN (VL: 0.018 to 0.034 mm3, MXD: 72.2 to 101.4 µm) was lower than or similar to that of CAD/CAM composite resins and the simulated wear of CM CR, CS, EX, GD, SO, SH and SY (VL: 0.042 to 0.194 mm3; MXD: 142.3 to 230.8 µm) was higher. This may indicate that there are indirect composite resins with wear resistance similar to that of CAD/CAM composite resins. On the other hand, Takamizawa et al.,16 which again used the same apparatus and methods to study simulated generalized wear over 200,000 cycles, reported that simulated wear was 0.311 to 0.919 mm3 of VL and 22.4 to 63.7 µm of MD for bis-acryl base provisional resins, and 1.046 mm3 of VL and 70.5 µm of MD for polymethyl methacrylate resin. The present study used 400,000 cycles to simulate the generalized wear of indirect composite resins. Even using 400,000 cycles, the simulated generalized wear of EX, GF, GP and SN (VL: 0.129 to 0.294 mm3; MD: 8.8 to 17.5 µm) was lower than that of bis-acryl base provisional resins after 200,000 cycles, and the simulated generalized wear of CM, CR, SH, and SL (VL: 0.345 to 0.608 mm3; MD: 22.9 to 47.5 µm) was similar or lower. In addition, all indirect composite resins showed lower simulated generalized wear than the polymethyl methacrylate resin after 200,000 cycles. Taken together, some indirect resin composites and CAD/CAM composite resins may be suitable as long-term provisional restorative materials, while bis-acryl base provisional resin and polymethyl methacrylate resin are more suitable for short-term use.
The decision to investigate wear characteristics of indirect composite resins using two distinct types of wear simulation is in line with the recommendations of the ISO publication18 that suggests at least two different wear tests should be used when making clinical predictions. The rank order of the localized wear characteristics of the indirect composite resins (VL and MXD: SN-GP-GF-EX-SY-GD-SH-SL-CR-CM) was the same as that of generalized wear (VL and MD: SN-GP-GF-EX-SY-GD-SH-SL-CR-CM) and regression analysis showed a strong correlation (R=0.945) between localized and generalized wear. This strongly suggests that the results for laboratory wear characteristics of indirect composite resins obtained in this study are reliable.
Barkmeier et al.24 reported that the rates of these types of simulated wear showed a strong relationship with clinical wear rates for composite resins, and that these simulation models appear to be effective ways to evaluate the clinical performance of composite resins. They reported that the simulated wear of Filtek P50 after 400,000 cycles was 0.014 mm3 for VL and 73.7 µm for MXD using localized wear simulation, and 0.191 mm3 for VL and 9.5 µm for MD using generalized wear simulation. In the present study, the simulated localized wear of SN (VL: 0.018 mm3; MXD: 72.2 µm) showed similar values to that of Filtek P50, and generalized wear of GP and SN (VL: 0.129 – 0.147 mm3; MD: 8.8 – 10.0 µm) showed lower or similar values to those of Filtek P50. Thus, the wear characteristics of NX and GP appear to be close to those of Filtek P50. Tsujimoto et al.22 also reported that the clinical wear rate of Filtek P50 was measured as 8.3 µm/year in 2004 and 2008 at the CU and 7.8 µm/year in 2004 at the Catholic University of Leuven School of Dentistry (CL). Therefore, combining the results of this study and previous studies, clinicians can predict the likely clinical wear rates of GP and SN (approximately < 10 µm/year) and understand that other indirect composite resins may have higher wear rates (> 10 µm/year).
In addition, the MD (9.5 µm) of P50 for generalized simulation (400,000 cycles) showed a similar value to clinical wear values at CU and CL (7.8 – 8.3 µm). In the present study, the MD of indirect composite resins for simulated generalized wear was 8.8 – 47.5 µm. Clinicians may be able to take those values as an indicator of the annual wear rate of indirect composite resins. However, further research is needed to clarify the relationship between the mean depths measured in simulations of generalized wear and the annual clinical wear rate.
The simulated localized and generalized wear of indirect composite resins for implant supported provisional restorations was material dependent, and Gradia Plus and SR Nexco paste exhibited significantly less simulated wear than other indirect composite resins. There was a strong positive relationship between the localized and generalized wear of these materials.
ACKNOWLEDGEMENTS
The authors thank Mr. Jason M. Moody for technical contributions. The authors of this manuscript certify that they have no proprietary, financial, or other personal interest of any nature or kind in any product, service, and/or company that is presented in this article.
References
1. Pjetursson BE, Asgeirsson AG, Zwahlen M, Sailer I. Improvements in implant dentistry over the last decade: comparison of survival and complication rates in older and newer publications. Int J Oral Maxillofac Implants. 2014; 29:308–324. PMID: 24660206.


2. Slagter KW, den Hartog L, Bakker NA, Vissink A, Meijer HJ, Raghoebar GM. Immediate placement of dental implants in the esthetic zone: a systematic review and pooled analysis. J Periodontol. 2014; 85:e241–e250. PMID: 24502614.


3. Hartlev J, Kohberg P, Ahlmann S, Andersen NT, Schou S, Isidor F. Patient satisfaction and esthetic outcome after immediate placement and provisionalization of single-tooth implants involving a definitive individual abutment. Clin Oral Implants Res. 2014; 25:1245–1250. PMID: 24024479.


4. Yan Q, Xiao LQ, Su MY, Mei Y, Shi B. Soft and hard tissue changes following immediate placement or immediate restoration of single-tooth implants in the esthetic zone: A systematic review and meta-analysis. Int J Oral Maxillofac Implants. 2016; 31:1327–1340. PMID: 27861657.


5. Bishti S, Strub JR, Att W. Effect of the implant-abutment interface on peri-implant tissues: a systematic review. Acta Odontol Scand. 2014; 72:13–25. PMID: 23834528.


6. Chen ST, Buser D. Esthetic outcomes following immediate and early implant placement in the anterior maxillaa systematic review. Int J Oral Maxillofac Implants. 2014; 29:186–215. PMID: 24660198.


7. Weigl P, Strangio A. The impact of immediately placed and restored single-tooth implants on hard and soft tissues in the anterior maxilla. Eur J Oral Implantol. 2016; 9:S89–S106. PMID: 27314114.
8. Santing HJ, Kleverlaan CJ, Werner A, Feilzer AJ, Raghoebar GM, Meijer HJ. Occlusal wear of provisional implant-supported restorations. Clin Implant Dent Relat Res. 2015; 17:179–185. PMID: 23594356.


9. Tarnow DP, Eskow RN. Preservation of implant esthetics: soft tissue and restorative considerations. J Esthet Dent. 1996; 8:12–19. PMID: 9468826.


10. Chee WW. Provisional restorations in soft tissue management around dental implants. Periodontol 2000. 2001; 27:139–147. PMID: 11551305.


11. Belser UC, Mericske-Stern R, Bernard JP, Taylor TD. Prosthetic management of the partially dentate patient with fixed implant restorations. Clin Oral Implants Res. 2000; 11:126–145. PMID: 11168262.


12. Burns DR, Beck DA, Nelson SK. Committee on Research in Fixed Prosthodontics of the Academy of Fixed Prosthodontics. A review of selected dental literature on contemporary provisional fixed prosthodontic treatment: report of the Committee on Research in Fixed Prosthodontics of the Academy of Fixed Prosthodontics. J Prosthet Dent. 2003; 90:474–497. PMID: 14586312.


13. Castellon P, Casadaban M, Block MS. Techniques to facilitate provisionalization of implant restorations. J Oral Maxillofac Surg. 2005; 63:72–79. PMID: 16125017.


14. Shibasaki S, Takamizawa T, Suzuki T, Nojiri K, Tsujimoto A, Barkmeier WW, Latta MA, Miyazaki M. Influence of different curing modes on polymerization behavior and mechanical properties of dual-cured provisional resins. Oper Dent. 2017; 42:526–536. PMID: 28605612.


15. Abdullah AO, Pollington S, Liu Y. Comparison between direct chairside and digitally fabricated temporary crowns. Dent Mater J. 2018; 37:957–963. PMID: 30135337.


16. Takamizawa T, Barkmeier WW, Tsujimoto A, Scheidel D, Erickson RL, Latta MA, Miyazaki M. Mechanical properties and simulated wear of provisional resin materials. Oper Dent. 2015; 40:603–613. PMID: 25405905.


17. Tsujimoto A, Barkmeier WW, Fischer NG, Nojiri K, Nagura Y, Takamizawa T, Latta MA, Miazaki M. Wear of resin composites: Current insights into underlying mechanisms, evaluation methods and influential factors. Jpn Dent Sci Rev. 2018; 54:76–87. PMID: 29755618.


18. ISO Standards. ISO14569 Dental materials - Guidance on testing of wear - Part 2: Wear by two- and/or three-body contact. ISO. 2001; 1:1–31.
19. Leinfelder KF, Suzuki S. In vitro wear device for determining posterior composite wear. J Am Dent Assoc. 1999; 130:1347–1353. PMID: 10492543.


20. Barkmeier WW, Takamizawa T, Erickson RL, Tsujimoto A, Latta M, Miyazaki M. Localized and generalized simulated wear of resin composites. Oper Dent. 2015; 40:322–335. PMID: 25706614.


21. Heintze SD, Reichl FX, Hickel R. Wear of dental materials: Clinical significance and laboratory wear simulation methods A review. Dent Mater J. 2019; 38:343–353. PMID: 30918233.


22. Tsujimoto A, Barkmeier WW, Takamizawa T, Latta MA, Miyazaki M. Influence of thermal stress on simulated localized and generalized wear of nanofilled resin composites. Oper Dent. 2018; 43:380–390. PMID: 29949478.


23. Tsujimoto A, Barkmeier WW, Takamizawa T, Latta MA, Miyazaki M. Influence of thermal cycling on flexural properties and simulated wear of computer-aided design/computer-aided manufacturing resin composites. Oper Dent. 2017; 42:101–110. PMID: 27802120.


24. Barkmeier WW, Latta MA, Erickson RL, Lambrechts P. Comparison of laboratory and clinical wear rates of resin composites. Quintessence Int. 2004; 35:269–274. PMID: 15119711.
Fig. 1
Illustration of the set-up for generalized and localized wear simulation with the Alabama wear testing machine.
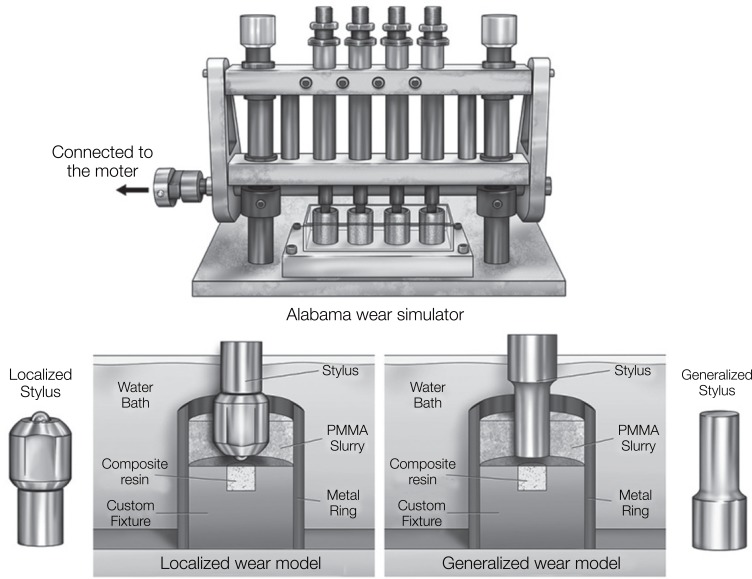
Fig. 2
SEM images of the localized wear facets. (A) Ceramage as viewed at ×500 magnification, (B) Ceramage as viewed at ×5,000 magnification, (C) Experia as viewed at ×500 m agnification, (D) Experia as viewed at ×5,000 magnification, (E) Gradia as viewed at ×500 magnification, (F) Gradia as viewed at ×5,000 magnification, (G) Gradia Forte as viewed at ×500 magnification, (H) Gradia Forte as viewed at ×5,000 magnification, (I) Gradia Plus as viewed at ×500 magnification, (J) Gradia Plus as viewed at ×5,000 magnification, (K) Signum ceramis as viewed at ×500 magnification, (L) Signum ceramis as viewed at ×5,000 magnification, (M) Solidex as viewed a t ×500 magnification, (N) Solidex as viewed at ×5,000 magnification, (O) Solidex Hardura as viewed at ×500 magnification, (P) Solidex Hardura as viewed at ×5,000 magnification, (Q) SR Nexco paste as viewed at ×500 m agnification, (R) SR Nexco paste as viewed at ×5,000 magnification, (S) Symphony as viewed at ×500 magnification, (T) Symphony as viewed at ×5,000 magnification.
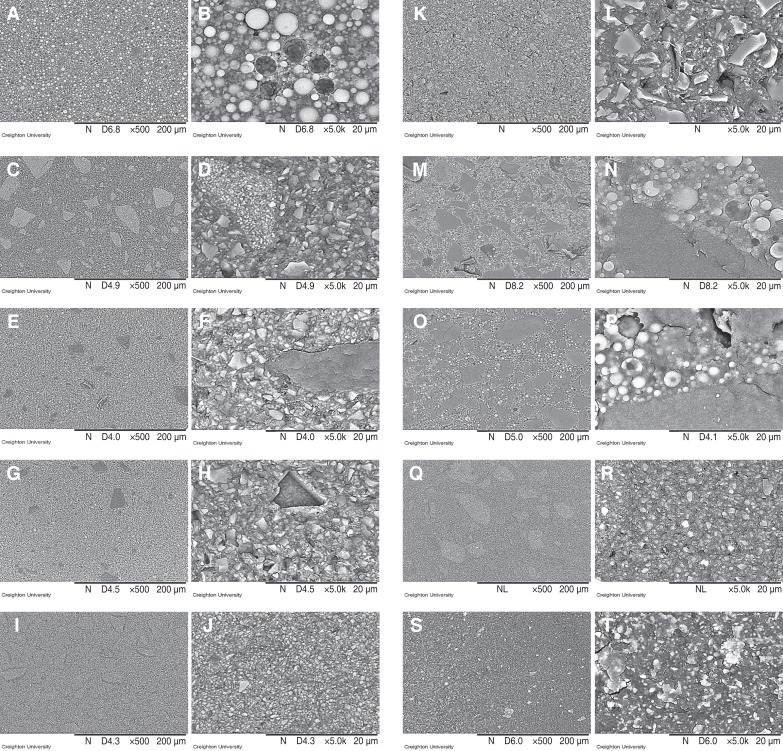
Table 1
Indirect composite resins used in this study
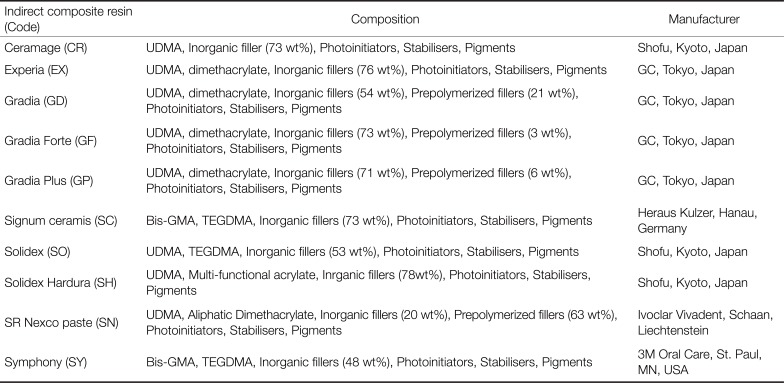
Table 2
Simulated localized wear of indirect composite resins
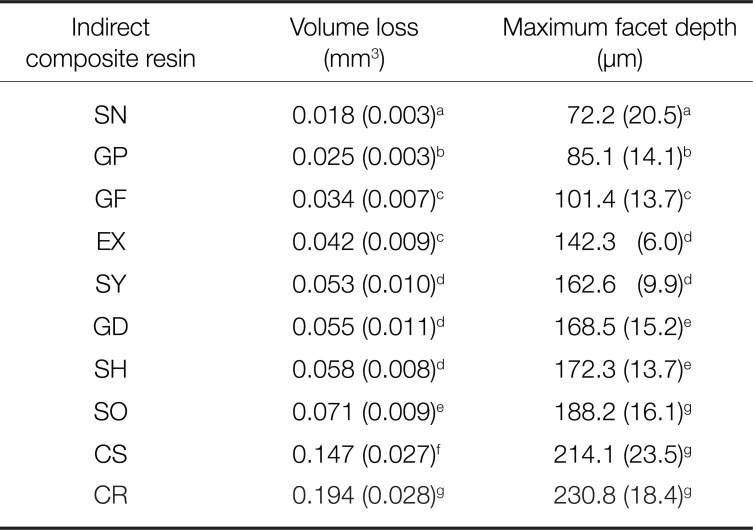
Table 3
Simulated generalized wear of indirect composite resins
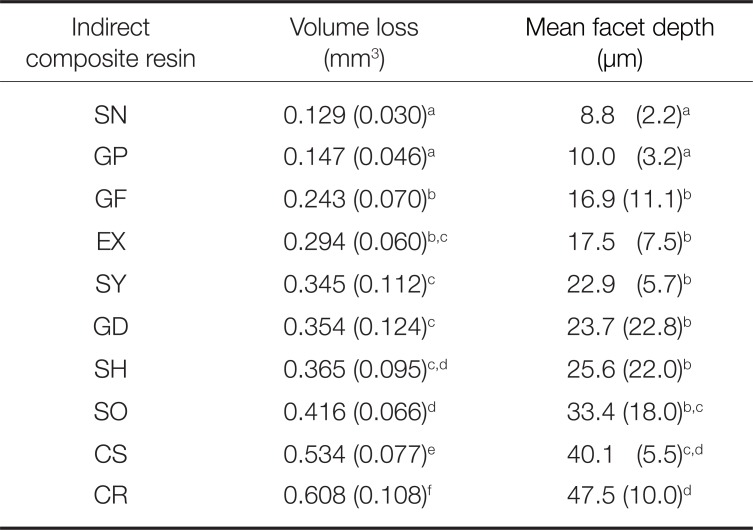