Abstract
PURPOSE
Fabrication of zirconia restorations with ideal mechanical properties in a short period is a great challenge for clinicians. The purpose of the study was to investigate the effect of heating rate on the mechanical and microstructural properties of monolithic zirconia.
MATERIALS AND METHODS
Forty monolithic zirconia specimens were prepared from presintered monolithic zirconia blanks. All specimens were then assigned to 4 groups according to heating rate as Control, Group 15℃, Group 20℃, and Group 40℃. All groups were sintered according to heating rates with the sintering temperature of 1500℃, a holding time of 90 minutes and natural cooling. The phase composition was examined by XRD analysis, three-point bending test was conducted to examine the flexural strength, and Weibull analysis was conducted to determine weibull modulus and characteristic strength. Average grain sizes were determined by SEM analysis. One-way ANOVA test was performed at a significance level of 0.05.
RESULTS
Only tetragonal phase characteristic peaks were determined on the surface of analyzed specimens. Differences among the average grain sizes of the groups were not statistically significant. The results of the three-point bending test revealed no significant differences among the flexural strength of the groups (P>.05). Weibull modulus of groups was ranging from 3.50 to 4.74. The highest and the lowest characteristic strength values were obtained in Group 20℃ and Control Group, respectively.
Zirconia restoration has been widely used in dentistry due to its high esthetics and excellent mechanical properties.123 Zirconia, a polymorphic material, exists in three forms, monoclinic, tetragonal, and cubic. Monoclinic phase is stable from room temperature up to 1170℃. Above this temperature monoclinic to tetragonal transformation occurs and tetragonal phase remains stable up to 2370℃. Finally, the cubic phase occurs up to the melting point of 2680℃.456 Tetragonal phase could be stable in room temperature by alloying the metastable zirconia with stabilizing oxides as Y2O3.5 As a result of stress and aging in oral conditions, tetragonal to monoclinic (t-m) phase transformation exists with a local rise in volume around 4 – 5%.7 The compressive stresses associated with the volumetric expansion create a barrier to crack propagation on the surface of the material and this phenomenon is called transformation toughening.8910 However, if the phase transformation continues into the depths of the material, crack growth enhance to some extent and catastrophic failures could exist. Therefore, the stability of the tetragonal phase is crucial for the long-term success of zirconia ceramics.8
Several factors influence the stability of zirconia ceramics such as the concentration of the stabilizing agents, the stabilizer distribution, the grain size, and distribution of zirconia.11 These parameters are interdependent and mainly influenced by fabrication procedures.512131415 It was stated that the flexural strength and durability of the conventional zirconia materials decrease with the increase of grain size.4 Computer-aided design and computer-aided manufacturing (CAD/CAM) generated zirconia restorations could be produced with two milling process as soft milling (from presintered blocks) and hard milling (from densely sintered blocs).4 Due to its advantages, soft milling is widely used in dental field and the these restorations are sintered to have full density.16 Sintering parameters (holding time, heating rate etc.), which are the major factor for obtaining stable dental restorations, predominantly influence the final properties of the zirconia restorations.101517 Although the long term success of the zirconia restorations has been proven, there are still major problems about conventional zirconia (first generation) restorations such as chipping.23 Therefore, CAD/CAM generated monolithic zirconia (second generation) restorations without veneering porcelain have been increasingly used in clinical practice for overcoming the mechanical problems. Monolithic zirconia enhances the esthetic of posterior restoration. Conventional zirconia is translucent by the optimization of sintering parameters with increasing the sintering temperature and time, but the higher sintering temperature negatively affects the low-temperature degradation.15 Therefore, the second generation of zirconia has been developed with nanoscale grain size. Zirconia manufacturing with nanoscale grain sizes starts with well dispersed homogeneous nanopowders containing controlled concentrations of dopants. As a result of reduced grain size, nanosized zircona showed enhanced mechanical properties and translucency.18 The increasing demand for chairside dentistry and the introduction of monolithic zirconia restorations (second generation) with shortened sintering procedures by manufacturers increased the interest on the effect of sintering parameters. Various studies examined the influence of holding time and temperature on the characteristics of zirconia ceramics, but the effect of heating rate on characteristics of zirconia ceramics is unclear.419202122
The aim of the study was to investigate the effect of heating rate on the mechanical and microstructural properties of monolithic zirconia ceramics. The first null hypothesis was that heating rate has no effect on the flexural strength of the zirconia ceramics. The second null hypothesis was that the heating rate has no effect on the microstructure and grain size of zirconia ceramics.
The schematic view of the study set-up was shown in Fig. 1. Totally, 40 preshaded (A2) monolithic zirconia specimens with the dimension of 20 × 5 × 1.5 ± 0.05 mm were prepared from pre-sintered monolithic zirconia blanks (Upcera ST-Color, Shenzhen Upcera Dental Technology Co., Ltd., Shenzhen, China) using a precision cutting device (Micracut 201, Metkon Instruments Inc., Bursa, Turkey) under water cooling. No power analysis was conducted and the sample size of each group was based on results of previous studies.192122
All specimens were then randomly divided into 4 groups (n = 10) according to heating rate as Control (10℃/min), Group 15℃ (15℃/min), Group 20℃ (20℃/min), and Group 40℃ (40℃/min). All groups were sintered according to heating rates with the sintering temperature of 1500℃, a holding time of 90 minutes, and natural cooling by a sintering furnace (Vita Zyrcomat 6000 MS, Vita Zahnfabrik). After the sintering process, final dimensions of the specimens were 16 × 4 × 1.2 ± 0.02 mm. A digital micrometer was used to measure the final dimensions of all specimens (Digital Micrometer IP65, Mitutoyo Europe GmbH, Neuss, Germany).
The effect of heating rates on the phase composition and t-m transformation were crystallographically examined using a diffractometer (Rigaku SmartLab, Rigaku Corporation) on one randomly selected sample from each group. All samples were subjected to Cu Ka radiation. The voltage and current were set to 40 kV and 30 mA. Diffraction profiles were recorded within the range of 5 – 90°, with a continuous θ/2θ scan with a step size of 0.05°, and a scan speed of 4.0 deg/min.
Three-point bending test was conducted using a universal testing machine (Shimadzu AGS-X, Shimadzu Scientific Instruments) to examine the flexural strength of the zirconia specimens. The test was conducted according to ISO 6872:201523 at a crosshead speed of 1 mm/min, with a 12 mm support distance and a loading rod that was 2 mm in diameter. The following formula was used for the calculation of the flexural strength:
(σ: flexural strength, N: fracture load (in N), l: distance between supports (in mm), b: width of the specimen (in mm), d: thickness of the specimen (in mm))
The Weibull analysis was conducted to obtain Weibull modulus and characteristic strength using the flexural strength data. The following formula was used for determination of Weibull distribution:
(Pf: fracture probability, σ: flexural strength, σ0: characteristic strength (the strength occurring at a probability of failure of 63.2%), and m: Weibull modulus)
One randomly selected sample from each group was polished and ultrasonically cleaned in an isopropanol solution. Then, samples were thermally etched for 20 min at 1350℃ and sputtered with gold/palladium. Scanning electron microscope (Quanta 450 FEG, FEI) was used at 30,000 magnification, operating at 5 kV with a working distance of 8.1 mm to image the grains boundaries. The mean grain size was determined using linear intercept technique with the following formula:
where D̄ is the average grain size, C the total length of test line used, and N the number of intercepts. The invariable proportion, 1.56, is an essential correction factor for polycrystalline ceramics.
Shapiro-Wilks test was performed to determine the distribution of data and the data showed normal distribution. Therefore, One-way ANOVA test was performed at a significance level of 0.05 using a software (SPSS version 20 Inc., IBM Corp. Chicago, IL, USA).
XRD analysis revealed that only tetragonal phase characteristic peaks were determined on the surface of analyzed specimens and no t-m transformation was determined within the groups (Fig. 2). The mean grain size of the subgroups was shown in Table 1. According to the results of the One-way ANOVA test conducted, no statistical differences were found among the average grain size of the subgroups (P > .05) and SEM images of the subgroups were shown in Fig. 3.
The results of three-point bending test and Weibull analysis were shown in Table 1. The results of the three-point bending test revealed no significant differences between the flexural strength of the groups (P > .05). Weibull modulus of groups was ranging from 3.50 to 4.74. Group 20℃ had the highest and Group 15℃ had the lowest Weibull modulus. Three-point flexural strength results and characteristic strength values were in the same order. The highest and the lowest characteristic strength values were obtained in Group 20℃ and Control Group, respectively. Weibull analysis plots were presented in Fig. 4.
This study was designed to compare the flexural strength of zirconia ceramics, produced in shorter sintering duration by modifying the heating rate. According to the results of the study, no significant differences were found among the flexural strength of groups. Therefore, the first null hypothesis of the study, that heating rate has no effect on the flexural strength of the zirconia ceramics, was accepted. XRD analysis showed that phase compositions of the groups were similar and no monoclinic phase was observed. Besides, SEM analysis revealed that no significant differences were found among the grain sizes of groups. Thus, the second null-hypothesis of the study, that the heating rate has no effect on the microstructure and grain size of zirconia ceramics, was also accepted.
The main advantage of the zirconia ceramics is its high flexural strength.24 In previous studies, the flexural strength of zirconia ceramics varied between 443 MPa and 1540 MPa as a result of sintering conditions, surface treatments and chemical composition.491011121322 In a previous study, Stawarcyzk et al.25 reported that measured flexural strength of monolithic zirconia specimens varied between 611 – 784 MPa and concluded that monolithic zirconia (second generation) has lower flexural strength values than conventional zirconia ceramics. In the current study, similar to a previous study,25 the mean flexural strength of monolithic zirconia specimens ranged between 612 MPa and 637 MPa and mean flexural strengths of all tested groups were above minimum value (500 MPa) for three-unit prosthesis involving molar restorations according to the ISO 6872:2015. In contrast, these specimens were not suitable for four or more units restoration (ISO minimum value: 800 MPa) according to ISO 6872:2015. Weibull analysis was conducted to determine the reliability of the groups and Weibull modulus of the groups was ranging from 3.50 to 4.74. Group 20℃ had the highest and Group 15℃ had the lowest Weibull modulus. A low modulus is related with a wide strength distribution and materials with low Weibull modulus are unpredictable. Therefore, the groups with lower Weibull modulus can fail at any stress level lower than mean flexural strength values. On the other hand, previous studies626 concluded that the better mechanical properties of zirconia ceramics depend on the transformation toughening mechanism. Kosmac et al.12 suggested that Y-TZP surfaces required surface treatments in clinical practice. Guazzato et al.10 concluded that improvement of the flexural strength of zirconia ceramics has been related to the increased amount of monoclinic phase after surface treatments. In current study, no surface treatments were applied to the specimens and no monoclinic phase was determined within the groups. Thus, lower flexural strength values acquired in this study than reported previously 4101222 could be related to the lack of surface treatments and transformation toughening and the microstructure of the zirconia ceramics used in this study.
Tetragonal to monoclinic transformation could be stimulated by tensile stresses, surface treatments, and sintering conditions.56 The sintering temperature is the major factor that influences the microstructure and phase composition of zirconia ceramics,2127 and increasing in heating and cooling rates can cause tensile stresses on the ceramic structure. Jiang et al.21 concluded that zirconia ceramics could have a higher density at the final sintering temperature between 1450℃ and 1500℃. Stawarczyk et al.4 concluded that the highest flexural strength of zirconia ceramic (3rd generation) could be obtained at the final sintering temperature between 1400℃ and 1550℃. Besides, Ebeid et al.22 concluded that changes in sintering temperature (1460℃ to 1600℃) and holding time (1 hour to 4 hour) did not influence the flexural strength and phase composition of zirconia ceramics(2nd generation). Ersoy et al.11 also stated that only tetragonal phases were observed on the surface of the zirconia specimens (2nd generation) that were sintered in different duration and temperature. Additionally, Hjerppe et al.14 did not find any monoclinic phase on control group that was sintered at 1500℃ and they concluded that changes in heating rate with the sintering temperature of 1500℃ have no effect on the mechanical properties of third generation zirconia ceramics. In this study, all samples were sintered at 1500℃ with the holding time of 90 minutes and cooled naturally. Similar to that of Hjerppe et al.,14 the current study revealed that heating rate has no effect on the flexural strength and phase composition of the zirconia ceramics. Therefore, it can be concluded that the use of the ideal sintering temperature and time and the natural cooling may prevent phase transformations and stress formation and eliminate the possible effect of the heating rate on the mechanical properties of the zirconia ceramics regardless of the zirconia generations.
The final grain size affects the mechanical properties of zirconia ceramics.18 Gupta et al.28 revealed that the average grain size below 0.3 µm is critical to obtain the highest flexural strengths of zirconia ceramics. According to Kelly and Denry,29 this crucial grain-size point out a strength/toughness mechanism, although the simple flaw-related grain size effects commonly recognized for polycrystalline ceramics. It is stated that the sintering temperature and time are the major factors that affect the final particle size.4 In a study, Bravo-Leon et al.30 concluded that spontaneous phase transformation, initiated by the particle size larger than 1 µm, decreased the flexural strength of zirconia. Besides, Kelly and Denry29 stated that when the average grains size of zirconia ceramics go below 0.2 µm, t → m transformation is never again conceivable, which results in unsuitable impact, for example, decreased flexural strength. Ebeid et al.22 stated that there is no phase transformation and no changes in mechanical properties of zirconia samples with particle sizes ranging from 0.55 to 1 µm. In this study, in accordance with the previous studies,4192427 the heating rate did not have a significant effect on particle size and no monoclinic phase were found on the surface of samples with the particle size ranging 0.38 to 0.39 µm.
The use of the only brand of monolithic zirconia and not investigating changes in monolithic zirconia due to aging in oral condition were the limitations of this study. Additionally, the effect of different and more complicated sintering protocols with different heating rates suggested by manufacturers, which may result in different effect on the optical, mechanical, and structural properties of monolithic zirconia, should be further investigated.
Within the limitation of the study, it can be concluded that the heating rate has no effect on the flexural strength of monolithic zirconia. The heating rate has no significant effect on phase transformation and grain size of monolithic zirconia. Manufacturing zirconia restorations with increased heating rate reduce sintering time and have no detrimental effect on the monolithic zirconia in terms of flexural strength.
References
1. Kim HK, Kim SH. Effect of the number of coloring liquid applications on the optical properties of monolithic zirconia. Dent Mater. 2014; 30:e229–e237. PMID: 24853434.


2. Choi YS, Kim SH, Lee JB, Han JS, Yeo IS. In vitro evaluation of fracture strength of zirconia restoration veneered with various ceramic materials. J Adv Prosthodont. 2012; 4:162–169. PMID: 22977725.
3. Triwatana P, Nagaviroj N, Tulapornchai C. Clinical performance and failures of zirconia-based fixed partial dentures: a review literature. J Adv Prosthodont. 2012; 4:76–83. PMID: 22737311.


4. Stawarczyk B, Ozcan M, Hallmann L, Ender A, Mehl A, Hämmerlet CH. The effect of zirconia sintering temperature on flexural strength, grain size, and contrast ratio. Clin Oral Investig. 2013; 17:269–274.


5. Lughi V, Sergo V. Low temperature degradation -aging- of zirconia: A critical review of the relevant aspects in dentistry. Dent Mater. 2010; 26:807–820. PMID: 20537701.


6. Piconi C, Maccauro G. Zirconia as a ceramic biomaterial. Biomaterials. 1999; 20:1–25. PMID: 9916767.


7. Studart AR, Filser F, Kocher P, Gauckler LJ. Fatigue of zirconia under cyclic loading in water and its implications for the design of dental bridges. Dent Mater. 2007; 23:106–114. PMID: 16473402.


8. Steffen AA, Dauskardt R, Ritchie R. Cyclic fatigue life and crack-growth behavior of microstructurally small cracks in magnesia-partially stabilized zirconia ceramics. J Am Ceram Soc. 1991; 74:1259–1268.
9. Papia E, Jimbo R, Chrcanovic BR, Andersson M, Vult von Steyern P. Surface structure and mechanical properties of impaction-modified Y-TZP. Dent Mater. 2014; 30:808–816. PMID: 24962988.


10. Guazzato M, Quach L, Albakry M, Swain MV. Influence of surface and heat treatments on the flexural strength of Y-TZP dental ceramic. J Dent. 2005; 33:9–18. PMID: 15652163.


11. Ersoy NM, Aydoğdu HM, Değirmenci BÜ, Çökük N, Sevimay M. The effects of sintering temperature and duration on the flexural strength and grain size of zirconia. Acta Biomater Odontol Scand. 2015; 1:43–50. PMID: 28642900.


12. Kosmac T, Oblak C, Jevnikar P, Funduk N, Marion L. The effect of surface grinding and sandblasting on flexural strength and reliability of Y-TZP zirconia ceramic. Dent Mater. 1999; 15:426–433. PMID: 10863444.
14. Hjerppe J, Vallittu PK, Fröberg K, Lassila LV. Effect of sintering time on biaxial strength of zirconium dioxide. Dent Mater. 2009; 25:166–171. PMID: 18632146.


15. Hallmann L, Ulmer P, Reusser E, Louvel M, Hämmerlea CHF. Effect of dopants and sintering temperature on microstructure and low temperature degradation of dental Y-TZP-zirconia. J Eur Ceram Soc. 2012; 32:4091–4104.


16. Guess PC, Att W, Strub JR. Zirconia in fixed implant prosthodontics. Clin Implant Dent Relat Res. 2012; 14:633–645. PMID: 21176095.


17. Chevalier J. What future for zirconia as a biomaterial? Biomaterials. 2006; 27:535–543. PMID: 16143387.


18. Kaizer MR, Gierthmuehlen PC, Dos Santos MB, Cava SS, Zhang Y. Speed sintering translucent zirconia for chairside one-visit dental restorations: Optical, mechanical, and wear characteristics. Ceram Int. 2017; 43:10999–11005. PMID: 29097830.


19. Kim MJ, Ahn JS, Kim JH, Kim HY, Kim WC. Effects of the sintering conditions of dental zirconia ceramics on the grain size and translucency. J Adv Prosthodont. 2013; 5:161–166. PMID: 23755342.


20. Hjerppe J, Närhi T, Fröberg K, Vallittu PK, Lassila LV. Effect of shading the zirconia framework on biaxial strength and surface microhardness. Acta Odontol Scand. 2008; 66:262–267. PMID: 18645687.


21. Jiang L, Liao Y, Wan Q, Li W. Effects of sintering temperature and particle size on the translucency of zirconium dioxide dental ceramic. J Mater Sci Mater Med. 2011; 22:2429–2435. PMID: 21922331.


22. Ebeid K, Wille S, Hamdy A, Salah T, El-Etreby A, Kern M. Effect of changes in sintering parameters on monolithic translucent zirconia. Dent Mater. 2014; 30:e419–e424. PMID: 25262211.


23. ISO 6872. Dentistry-ceramic materials. Geneva; Switzerland: International Standards Organization (ISO);2015. Available at: https://www.iso.org/standard/74247.html.
24. Guazzato M, Albakry M, Ringer SP, Swain MV. Strength, fracture toughness and microstructure of a selection of all-ceramic materials. Part II. Zirconia-based dental ceramics. Dent Mater. 2004; 20:449–456. PMID: 15081551.


25. Stawarczyk B, Frevert K, Ender A, Roos M, Sener B, Wimmer T. Comparison of four monolithic zirconia materials with conventional ones: Contrast ratio, grain size, four-point flexural strength and two-body wear. J Mech Behav Biomed Mater. 2016; 59:128–138. PMID: 26751707.


26. Kosmac T, Oblak C, Jevnikar P, Funduk N, Marion L. The effect of surface grinding and sandblasting on flexural strength and reliability of Y-TZP zirconia ceramic. Dent Mater. 1999; 15:426–433. PMID: 10863444.
27. Denry I, Kelly JR. State of the art of zirconia for dental applications. Dent Mater. 2008; 24:299–307. PMID: 17659331.


28. Gupta TK, Lange FF, Bechtold JH. Effect of stress-induced phase transformation on the properties of polycrystalline zirconia containing metastable tetragonal phase. J Mater Sci. 1978; 13:1464–1470.


29. Kelly JR, Denry I. Stabilized zirconia as a structural ceramic: an overview. Dent Mater. 2008; 24:289–298. PMID: 17624420.


Fig. 2
XRD diffractograms of Groups; (A) Control Group, (B) Group 15℃, (C) Group 20℃ and (D) Group 40℃. (t) indicates the tetragonal characteristic peaks.
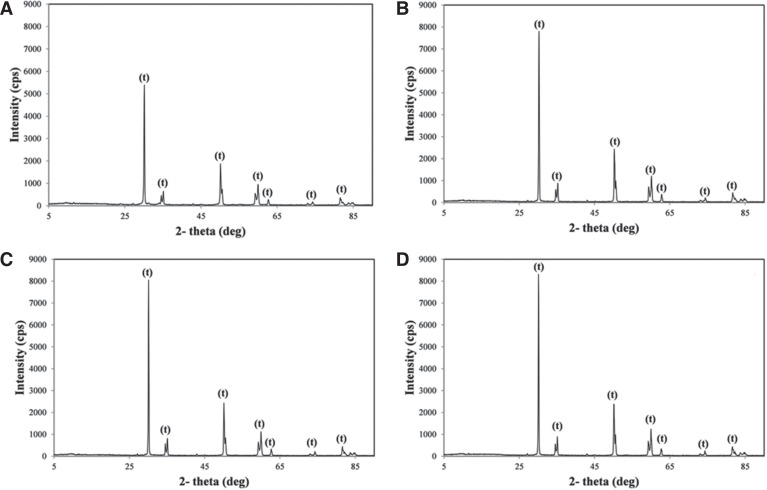
Table 1
Flexural strength (MPa), Characteristic strength (σ0), Weibull modulus (m), and mean grain size values for groups. Same superscript letters in the same column indicate no statistical difference (P > .05)
