Abstract
Mosapride accelerates gastric emptying by acting on 5-hydroxytryptamine type 4 (5-HT4) receptor and is frequently used in the treatment of gastrointestinal (GI) disorders requiring gastroprokinetic efficacy. We tested the effect of mosapride on 5-hydroxytryptamine type 3 (5-HT3) receptor currents because the 5-HT3 receptors are also known to be expressed in the GI system and have an important role in the regulation of GI functions. Using the whole-cell voltage clamp method, we compared the currents of the 5-HT3 receptors when 5-HT was applied alone or was co-applied with mosapride in cultured NCB-20 cells known to express 5-HT3 receptors. The 5-HT3 receptor current amplitudes were inhibited by mosapride in a concentration-dependent manner. Mosapride blocked the peak currents evoked by the application of 5-HT in a competitive manner because the EC50 shifted to the right without changing the maximal effect. The rise slopes of 5-HT3 receptor currents were decreased by mosapride. Pre-application of mosapride before co-application, augmented the inhibitory effect of mosapride, which suggests a closed channel blocking mechanism. Mosapride also blocked the opened 5-HT3 receptor because it inhibited the 5-HT3 receptor current in the middle of the application of 5-HT. It accelerated desensitization of the 5-HT3 receptor but did not change the recovery process from the receptor desensitization. There were no voltage-, or use-dependency in its blocking effects. These results suggest that mosapride inhibited the 5-HT3 receptor through a competitive blocking mechanism probably by binding to the receptor in closed state, which could be involved in the pharmacological effects of mosapride to treat GI disorders.
Mosapride is a gastroprokinetic agent that increases gastrointestinal (GI) motility by stimulating contraction of the GI tract [12]. Although not completely understood, the prokinetic mechanism of mosapride is mediated by agonist action on the 5-hydroxytryptamine (5-HT, serotonin) type 4 (5-HT4) receptor in the enteric nervous system that facilitate cholinergic excitatory neurotransmission and thereby, increase propulsive motility [3]. However, several studies have shown that some of benzamide gastroprokinetics also inhibited fast transient depolarization to 5-HT mediated by 5-HT type 3 (5-HT3) receptors in the myenteric neurons [456] and suggested the 5-HT3 receptor antagonistic properties of these drugs.
5-HT3 receptors belong to the Cys-loop receptor family of ligand-gated ion channels [7], are permeable to cations, and can induce a fast inward current in neurons [8]. 5-HT3 receptors are expressed in neural tissues throughout the central and the peripheral nervous system [910], including enteric neurons in the GI tract [1112], and have been proposed as a potential drug treatment target for various neurologic disorders [131415]. The activation of 5-HT3 receptors in the GI tract is known to be involved in the regulation of GI motility, visceral sensation, secretion, and certain variants of the HTR3 gene are associated with a number of functional GI diseases, such as irritable bowel syndrome (IBS), gastroesophageal reflux disease, and anorexia [13]. Besides the established role of 5-HT3 receptor antagonists in anti-emetic drugs [16], a meta-analysis study by Zheng et al. [17] reported that 5-HT3 receptor antagonists were effective treatments for nonconstipated IBS or diarrhea-predominant IBS. The mixed action of mosapride, i.e., an agonist of the 5-HT4 receptor and an antagonist of the 5-HT3 receptor, could be essential to understanding whether it is beneficial or harmful in the treatment of GI motility disorders, especially diarrhea-predominant IBS. However, to our knowledge, there have been no reports evaluating the direct effects of mosapride on 5-HT3 receptor ion channel function through the patch clamp technique. Accordingly, we tested the effects of mosapride on the 5-HT3 receptor using whole-cell voltage clamp recording combined with a fast drug application technique.
Mosapride citrate was purchased from Santa Cruz Biotechnology (Santa Cruz, CA, USA). Serotonin hydrochloride and all other chemicals were purchased from Sigma-Aldrich (St. Louis, MO, USA). Cell culture reagents were purchased from Gibco (Thermo Fisher Scientific, Waltham, MA, USA).
NCB-20 neuroblastoma cells were provided by Dr. Lovinger (National Institute on Alcohol Abuse and Alcoholism [NIAAA], Bethesda, MD, USA). The NCB-20 cells were maintained under previously described conditions [18]. Frozen cell stocks were maintained in liquid nitrogen and thawed as needed. The cells were grown in medium containing 89% Dulbecco's Modified Eagle's Medium, 10% fetal bovine serum, and 1% hypoxanthine aminopterin thymidine supplement and were cultured in an incubator at 37℃ with 5% CO2. The cells were seeded onto 35-mm culture dishes at least 2 days prior to recording. Culture medium was changed to extracellular solution 1–2 h before recording and the cells were transferred onto cover glasses and moved to the recording chamber.
Whole-cell patch-clamp recordings were performed at room temperature (25℃) on the stage of an inverted microscope (DM IRM; Leica, Wetzler, Germany). The cells were continuously perfused with an extracellular solution containing (in mM) 150 NaCl, 2.5 KCl, 2.5 CaCl2, 0.1 MgCl2, 10 N-(2-hydroxyethyl) piperazine-N′-2-ethansulfonic acid (HEPES), and 10 D-glucose (pH adjusted to 7.4 with NaOH and osmolality adjusted to 340 mOsm/kg with sucrose) at a rate of 2–3 ml/min. Patch pipettes were made from borosilicate glass capillaries (1B150F-4; World Precision Instruments, Sarasota, FL, USA) which were pulled with a horizontal micropipette puller (P-97; Sutter Instrument Co., Novato, CA, USA). The pipette tips had resistances of around 2 MΩ when filled with internal solution containing (in mM) 140 CsCl, 2 MgCl2, 5 ethylene glycol bis(2-aminoethylether)-N,N,N′,N′-tetraacetic acid, and 10 HEPES (pH adjusted to 7.4 with CsOH, osmolality adjusted to 310 mOsm/kg with sucrose). Whole-cell currents were recorded using an Axopatch 200B amplifier and pClamp 9 software (Molecular Devices, Sunnyvale, CA, USA). 5-HT3 receptor currents were recorded at a holding potential of −50 mV unless otherwise stated. The signals were filtered at 2 kHz, digitized at 10 kHz, and saved on a PC using DigiData 1322 and pClamp 9 software (Molecular Devices). The liquid junction potentials were zeroed before a gigaohm seal was formed. The capacitive currents were compensated with analog compensation, but leak subtraction was not used in this study.
After a whole-cell configuration, the cells were lifted up and positioned in front of one side of two theta glass micropipette barrels, which were pulled from theta glass capillary tubes (Clark Borosilicate Theta; Warner Instruments, Hamden, CT, USA; 2 mm outer diameter, 1.4 mm inner diameter, 0.2 mm septum thickness) to an outer diameter of ~300 mm and continuously perfused with extracellular solution. The extracellular solution, which contained agonists with or without mosapride, flowed through a different side of the theta glass micropipette. The solutions were rapidly switched around the cell by the linear movement of the theta glass micropipette mounted on a piezoelectric translator (P-601 PiezoMove Z Actuator; Physik Instrumente, Karlsruhe, Germany) driven by a Piezo Servo Controller (E-625, Physik Instrumente) and triggered by pClamp 9 software (Molecular Devices), which displaced the theta glass micropipette laterally in msec time resolution. Therefore, the cells could be exposed to the drug-containing extracellular solution for a defined period of time, then rapidly returned to the drug-free extracellular solution. The solution flow was driven by gravity from the reservoirs and solution switching was controlled by a perfusion valve control system (VC-8; Warner Instruments). The drugs were diluted with extracellular solution to the final concentration from stock solutions in distilled water (5-HT) or dimethyl sulfoxide (mosapride). The solutions were freshly prepared prior to each experiment.
The current peak amplitudes were measured using Clampfit 10 software (Molecular Devices), by determining the differences in currents before and during agonist application. Since peak amplitudes of the 5-HT3 receptor currents induced by agonist application varied between cells, the data were normalized to the maximal values in each cell. The rise slopes of currents were also analyzed to evaluate 5-HT3 receptor activation using the built-in statistical tools in Clampfit 10. Receptor desensitization was measured as the decay in 5-HT3 receptor current during agonist application for 10 sec, then the currents decay slopes were analyzed.
The concentration-response data were fitted to a sigmoid curve, calculated by the four-parameter logistic equation using Prism 8 software (GraphPad Software, San Diego, CA, USA).
For the EC50 of 5-HT:
where the bottom is the minimal response, the top is the maximal response by 5-HT, X is the logarithmic concentrations of 5-HT, and EC50 is the 5-HT concentration that gives a half-maximal response.
For the IC50 of mosapride:
where the bottom is the maximally inhibited response by mosapride, the top is the maximal response by 5-HT alone, X is the logarithmic concentrations of mosapride and IC50 is the concentration of mosapride resulting in a half-maximal response.
Interaction kinetics between the drug and channel are described on the basis of a first-order blocking scheme [19]. The apparent rate constants for association (k+1) and dissociation (k−1) were calculated from the following equations:
where τD is the drug-induced time constant, calculated from single exponential fits to the decay of currents by mosapride (for 5 sec) 3 sec after 5-HT application.
All averaged values are presented as means ± standard error of the mean. Statistical significance was determined by Student's t-tests. p-values < 0.05 were considered statistically significant.
To study the direct effects of mosapride on the 5-HT3 receptor induced currents, we examined the concentration-dependent effect of mosapride (0.3, 1, 3, 10, 30 µM) on the currents by 3 µM 5-HT, a concentration near the EC50 of 5-HT3 receptor currents reported in our previous study [18]. The application of 30 µM mosapride for 5 sec did not induce any current (Fig. 1A). The coapplication of mosapride decreased peak amplitudes of 3 µM 5-HT-evoked currents in a concentration-dependent manner (Fig. 1A). The IC50 of mosapride that resulted in peak amplitude reduction, calculated using equation (2) in the Materials and Methods, was 4.03 ± 0.04 µM with a Hill coefficient of −1.45 ± 0.13 (Fig. 1B, n = 10). Mosapride also decreased the current rise slopes, an indicator of activation of the 5-HT3 receptor [20]. The inhibition of the rise slope was concentration dependent, seen at low concentrations of mosapride (0.3, 1, 3 µM) in the tested range (Fig. 1C). We then studied whether mosapride inhibited the 5-HT3 receptor in a competitive manner. Fig. 2A presents the representative 5-HT3 receptor currents by 1, 3, 10, 30 µM of 5-HT with or without co-application of mosapride (10 µM, near the IC50 based on this study). The co-application of mosapride inhibited the currents activated by 1–30 µM 5-HT (Fig. 2B). The EC50 value of peak currents amplitudes by the 5-HT alone, calculated using equation (1), was 1.88 ± 0.10 µM with a Hill coefficient of 2.55 ± 0.12 (n = 10). The EC50 value of the peak currents amplitudes by the coapplication of 5-HT with 10 µM of mosapride was 4.80 ± 0.32 µM with a Hill coefficient of 2.60 ± 0.26 (n = 8). The co-application of 10 µM mosapride increased the EC50 (p < 0.001, unpaired t-test), however, the Hill coefficients were not significantly different statistically (p = 0.8532). The peak currents amplitudes induced by 30 µM of 5-HT did not show significant difference between 5-HT application alone and 5-HT co-applied with 10 µM mosapride (97.0 ± 2.8% and 95.4 ± 3.2% of the 10 µM 5-HT response, respectively; p = 0.7282, unpaired t-test). These findings suggest that mosapride inhibited the 5-HT3 receptor in a competitive manner.
In order to determine whether mosapride affected the closed state of the 5-HT3 receptor, we compared the extent of 5-HT3 receptor current blocking by pretreatment with 10 µM mosapride for 1 min prior to the co-application of 10 µM 5-HT with the co-application of 5-HT and mosapride without pretreatment. There were significant differences in the inhibition of peak currents when the co-application of 5-HT and mosapride after 1 min pretreatment (31.1 ± 4.1% of 5-HT alone) compared to coapplication without pretreatment (85.0 ± 2.9%) (Fig. 3B, n = 10, p < 0.001, paired t-test). These results suggest that mosapride probably bound to the closed state and blocked channel opening of the 5-HT3 receptors. To address the effects of mosapride on the 5-HT3 receptor in detail, i.e., whether it binds to the closed or open state of ion channels, we next tested the effects of mosapride during the application of 3 µM 5-HT. Different doses (1, 3, 10, 30 µM) of mosapride were co-treated 3 sec after 5-HT application for 5 sec, then 5-HT3 receptors were reactivated upon termination of the mosapride application. The currents activated by 3 µM 5-HT were decreased following the subsequent application of mosapride in a concentration-dependent manner (Fig. 4). These results suggest that mosapride bound to the open state of 5-HT3 receptors, then accelerated the decay of 5-HT3 receptor currents by increasing desensitization, i.e., an open-channel blocking mechanism. The rate of current decay by mosapride (for 5 sec) 3 sec after the 5-HT application was fitted to a single exponential function and the time constants (τD) were taken to estimate the drug-openchannel interaction kinetics. The relationship between τD and concentration of mosapride was described by equation (3) in Materials and Methods. The slope of this linear function yielded an association rate constant (k+1 = 0.02 µM−1sec−1) and the intercept at the y axis gave a dissociation rate constant (k−1 = 0.27 sec−1, Fig. 4B, n = 9). From equation (4), the apparent Kd (=k−1/ k+1) was 12.5 µM and it was much higher than the IC50 of 4.03 µM shown in Fig. 1B.
To examine if mosapride altered the desensitization of the 5-HT3 receptor, a long duration (10 sec) of high concentration 5-HT (10 µM), with or with mosapride, was applied to induce more profound desensitization following channel activation, then the current decay slopes were analyzed. Fig. 5A shows the superimposed current traces induced by 5-HT 10 µM for 10 sec with or without mosapride (10 µM). The decay slope of currents during the 5-HT application were increased by the co-application of mosapride (0.12 ± 0.02 pA/ms for 5-HT alone and 0.15 ± 0.03 pA/ms for mosapride co-application; Fig. 5B, n = 10, p < 0.01, paired ttest). These results suggest that mosapride accelerated 5-HT3 receptor desensitization. The effects of mosapride on the 5-HT3 receptor desensitization recovery time course were studied using two pulses of 5-HT application in variable inter-pulse intervals (1, 5, 10, 30, 60 sec), then the peak amplitudes of the second pulse were normalized to the first peak amplitude (paired-pulse ratio) and plotted against the inter-pulse intervals. Fig. 5 shows the representative superimposed current traces evoked by two pulses of 10 µM 5-HT for 5 sec in the inter-pulse intervals of 1, 5, 10, 30, 60 sec with (5D) or without (5C) 10 µM mosapride. A single exponential function was fitted to the 5-HT3 receptor desensitization recovery time course with a time constant of 3.85 ± 0.47 sec (n = 9) for 5-HT alone and 3.99 ± 0.44 sec (n = 9) for 5-HT and mosapride co-application (Fig. 5E, p = 0.5588, unpaired t-test). These results suggest that mosapride did not change the 5-HT3 receptor desensitization recovery time course.
In the present study, we examined the direct inhibitory action of mosapride, a gastroprokinetic drug, on 5-HT3 receptors naturally expressed on NCB-20 cells. Our results suggest that mosapride predominantly inhibited 5-HT3 receptor function by blocking channel opening, because mosapride reduced the peak current amplitudes accompanied by an attenuation in the current rise slopes, an indicator of the activation of 5-HT3 receptor ion channels [20], in a concentration-dependent manner by the mosapride co-application (Fig. 1). Thus, mosapride likely exerted its inhibitory effect on 5-HT3 receptor during the channel activation, i.e., channel opening. However, our finding that pretreatment with mosapride for 1 min augmented the inhibitory effect on the 5-HT3 receptors suggests the possibility that mosapride bound to closed-state 5-HT3 receptors also, and thereby, blocked the subsequent ion channel opening. To investigate the binding of mosapride with the 5-HT3 receptors during channel open state, mosapride was co-applied 3 sec after 5-HT application. At this time, the inward currents still remained, thus a large proportion of the 5-HT3 receptors probably stayed in an open state. The application of mosapride used in this protocol inhibited 5-HT3 receptor currents in a concentration-dependent manner based on time constants analysis. These results suggest that mosapride also bound to the open-state of 5-HT3 receptor, then accelerated channel desensitization. Taken together, these results suggest that mosapride acted on both the closed and open-state 5-HT3 receptors, then inhibited the 5-HT3 receptors. Also, mosapride bound to the agonist binding sites of the 5-HT3 receptor, both in the closed and open states, thus, competitively blocking the channel open or accelerating channel desensitization. In the same experiment, the calculated IC50 values of mosapride on 5-HT3 receptor currents (Fig. 4B) were three times larger than the IC50 values shown in Fig. 1B. The larger IC50 values for the open state of the 5-HT3 receptor suggest that mosapride had a higher affinity for this receptor in the closed state, thus, mosapride showed a strong inhibition in this state. This interpretation is supported by our findings that 1) the concentration-response curve of 5-HT on 5-HT3 receptor currents was shifted to the right, without a change in the maximal effect produced by mosapride (Fig. 2); and 2) its inhibitory effect on the 5-HT3 receptor was profoundly augmented by pretreatment with mosapride (Fig. 3). These are typical characteristics of channel blockers that competitively bind to the agonist binding sites of receptors, then block channel opening in ligand-gated ion channels [19]. Mosapride also altered the desensitization properties of 5-HT3 receptor channels, i.e., the slopes for current decay produced by the application of 5-HT for longer times (10 sec) were increased by mosapride but did not change the desensitization recovery time course (Fig. 4). In many ligandgated ion channels, desensitization is correlated with the receptor activation, i.e., if more channels are opened, they will be more strongly desensitized, and thus the rate of desensitization is increased in an agonist concentration dependently [20]. Therefore, the acceleration of receptor desensitization by mosapride in our experiment was possibly by secondary effects following the primary inhibition of opening. However, a direct effect of mosapride on the 5-HT3 receptor desensitization itself could not be excluded because some drugs have been known to modulate 5-HT3 receptor desensitization process directly [2122]. Thus, further studies clarifying the effect of mosapride on receptor desensitization process are necessary. In our study, mosapride inhibited the 5-HT3 receptor currents in a voltage-independent manner without a reversal potential change and there was no use-dependency in the inhibition (Supplementary Figs. 1 and 2). These results suggest that mosapride preferentially bound to the 5-HT3 receptor in a closed state and inhibited ion channel opening, because the voltage- and use- dependency of an inhibitory effect are considered evidences for an open channel blocking mechanism in ligandgated ion channels including 5-HT3 receptors [23]. Thus, this interpretation need to elucidate further, because our findings that the peak amplitude reduction was accompanied by a decrease in the rise slope (Fig. 1) and inhibition of 5-HT3 receptors in an open state by mosapride (Fig. 4) also suggest an open channel blocking mechanism.
5-HT3 receptors modulate the release of various neurotransmitters from neurons, such as dopamine, GABA and glutamate [24], and are involved in a complex neuronal function in the nervous system [25]. In the peripheral nervous system, especially in the GI system, 5-HT3 receptors were widely expressed in the afferent neurons of the submucosal plexus and are known to be involved in GI functions, such as visceral perception, secretion, and motility [26]. The activation of 5-HT3 receptors in the extrinsic primary afferent neurons in the submucosal plexus has been reported to trigger a reflex inducing nausea and emesis [27]. Thus, 5-HT3 receptor antagonists exert their anti-emetic effect by blocking these receptors and are widely used in the treatment of chemotherapeutic agent-induced nausea [16]. 5-HT3 receptors are also known to mediate a small subset of fast excitatory postsynaptic potential of enteric neurons [28]. This can contribute to regulation of the propulsive motility of the gut, and if blocked, generates constipation, a common adverse effect of 5-HT3 receptor antagonists for using anti-emetic purposes [29]. Collectively, 5-HT3 receptor inhibition blocks visceral perception and propulsive motility and these actions can be exploited for the treatment of GI disorders, such as IBS. IBS is a complex disorder characterized by abdominal pain or discomfort associated with disordered defecation, either constipation, diarrhea, or mixed [30]. Hypersensitivity of visceral perception in the GI tracts is known to be a pathophysiological mechanism of IBS [26]. Therefore, blocking visceral perception mediated by 5-HT3 receptors in the submucosal extrinsic primary afferent neuron could be an important strategy for the treatment of IBS. Even the 5-HT4 receptor agonists are major gastroprokinetic drugs for the treatment of IBS [31] and the additional 5-HT3 receptor blocking could be very useful for the treatment of certain forms of IBS, like diarrhea-prominent IBS. Ramostron, cilansetron, ondansetron, and alosetron have been reported to be selective 5-HT3 receptor antagonists, effective for treating diarrhea-prominent IBD with rare serious adverse reactions [17]. Even though a receptor binding assay reported that mosapride showed a weak affinity for 5-HT3 receptors [32], mosapride exerted its prokinetic effect in the GI tract mainly through selective 5-HT4 receptor agonist activity, and thus was effective in improving overall symptoms in patients with GI disorders, including chronic gastritis, gastro-esophageal reflux diseases, and functional dyspepsia [2]. Our patch clamp study showing that mosapride directly inhibited 5-HT3 receptor function expressed in NCB-20 cells provides evidence that mosapride has substantial 5-HT3 receptor antagonist activity, as well as 5-HT4 receptor agonist activity, and could contribute to the above mentioned pharmacological actions of mosapride. Moreover, this combined effect on the 5-HT receptor, 5-HT3 receptor antagonist activity as well as 5-HT4 receptor agonist activity, suggests that the clinical use of mosapride could be extended to the treatment of IBS, especially diarrhea-prominent types.
In summary, we showed that mosapride directly inhibited 5-HT3 receptor-induced currents in a competitive manner by binding to both the closed and open states of the receptor, but preferentially to the closed state. In addition to the known pharmacologic activity of mosapride, selective 5-HT4 receptor agonist activity, the additional 5-HT3 receptor antagonist activity could also contribute to the therapeutic effects of mosapride for the treatment of functional GI disorders.
Figures and Tables
Fig. 1
Concentration-dependent inhibition of 5-hydroxytryptamine type 3 (5-HT3) receptor currents by mosapride.
(A) Representative current traces induced by 3 µM 5-HT co-applied with 0.3, 1, 3, 10, 30 µM mosapride. The open horizontal bar indicates the drug application period. Mosapride alone did not induce any current even at high concentration (indicated by arrowhead). (B) Averaged concentration-dependent inhibition of mosapride on the 5-HT3 receptor current peak amplitude. The data were normalized values (○) to the peak amplitude induced by 3 µM 5-HT alone and a line was obtained from fitting these data to equation (2) in the Methods. (C) Averaged concentration-dependent block of the 5-HT3 receptor current rise slope by mosapride. Mosapride concentration-dependently decreased the rise slopes of currents at low concentrations (0.3, 1, 3 µM). The data are expressed as means ± standard error of the mean.
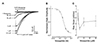
Fig. 2
Competitive inhibition of mosapride on the 5-hydroxytryptamine type 3 (5-HT3) receptor currents.
(A) Representative traces of 5-HT3 receptor currents induced by 1, 3, 10, 30 µM 5-HT in the presence (black traces) or absence of 10 µM of mosapride (gray traces), near IC50 seen in Fig. 1B. The open horizontal bars indicate the drug application period. (B) Averaged concentration-response curve of 5-HT3 receptor currents in the presence (●) or absence (○) of mosapride. The data were normalized to the peak amplitude induced by 10 µM 5-HT and lines were obtained by fitting these data to equation (1) in the Methods. The EC50 of 5-HT was increased from 1.88 ± 0.10 µM (n = 10) to 4.80 ± 0.32 µM (n = 8) by mosapride (p < 0.001, unpaired t-test) without Hill coefficient changes. There were no significant differences in the peak amplitudes induced by 30 µM 5-HT alone and the co-application of mosapride with 30 µM 5-HT (p = 0.7282, unpaired t-test). The data are expressed as means ± standard error of the mean.

Fig. 3
Closed channel blocking effect of mosapride on the 5-hydroxytryptamine type 3 (5-HT3) receptor.
(A) Representative trace of 5-HT3 receptor currents induced by 5-HT (10 µM) alone, co-application of mosapride (10 µM) and 5-HT, and co-application 1 min after mosapride pretreatment. The open horizontal bar indicates the 5-HT application period and the closed bar indicates mosapride application. (B) Averaged inhibitory effect on the peak amplitude of 5-HT3 receptor currents. Pretreatment for 1 min significantly increased the inhibitory effect of mosapride (n = 10, *p < 0.001, paired t-test). The data are expressed as means ± standard error of the mean.
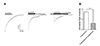
Fig. 4
Effects of mosapride on the open state of 5-hydroxytryptamine type 3 (5-HT3) receptor.
(A) Superimposed sample traces show the 5-HT (3 µM, 13 sec, open horizontal bar)-evoked currents and the effect of subsequent application of mosapride co-application (0.3, 1, 3, 10, 30 µM, 5 sec, closed horizontal bar) on these currents. (B) The current decay by mosapride (for 5 sec) 3 sec after 5-HT application was fitted to a single exponential function, and the time constants (τD) were taken as an approximation of the drug-open channel interaction kinetics. The relationship between τD and mosapride concentration was described by equation (3) in the Methods. The slope of this function yielded an association rate constant (k+1 = 0.02 µM−1sec−1) and the intercept at the ordinate gave a dissociation rate constant (k−1 = 0.27 sec−1, n = 9). From equation (4) in the Methods, the calculated IC50 was 12.5 µM. The data are expressed as means ± standard error of the mean.
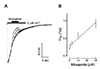
Fig. 5
Effect of mosapride on the desensitization of 5-hydroxytryptamine type 3 (5-HT3) receptor and the time course of desensitization recovery.
(A) Representative 5-HT3 receptor currents induced by 10 µM of 5-HT for 10 sec with (black trace) or without (gray trace) 10 µM mosapride. The open horizontal bars indicate the drug application period. (B) Averaged bar graph shows the effects of mosapride on the current decay slope after the long application of 5-HT to test the effect of mosapride on 5-HT3 receptor desensitization (n = 10, *p < 0.01, paired t-test). (C, D) Superimposed sample current traces evoked by two pulses of 10 µM 5-HT for 5 sec in the inter-pulse intervals of 1, 5, 10, 30, 60 sec with (D) or without (C) 10 µM mosapride. Gray arrowheads indicate the first application and the black arrowheads indicate the second application of 5-HT. (E) Averaged data of the paired-pulse ratio (the second peak amplitudes / the first peak amplitude) plotted against the inter-pulse intervals. A single exponential function was fitted to the data and compared to the time constants of 5-HT alone (○, n = 9) and the co-application 5-HT and mosapride (●, n = 9). Mosapride did not change the time course of desensitization recovery of 5-HT3 receptor (p = 0.5588, unpaired t-test). The data are expressed as means ± standard error of the mean.
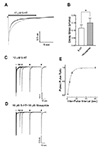
ACKNOWLEDGEMENTS
This research was supported by the Basic Science Research Program through the National Research Foundation of Korea (NRF) funded by the Ministry of Science (NRF-2017R1D1A1B03034551). We thank Dr. Lovinger (NIAAA) for providing us with NCB-20 cells.
Notes
Author contributions Conception and design of study: Y.S.P. and K.W.S. Conducting experiments, acquisition of data, analysis, and interpretation of data: Y.S.P. Drafting the manuscript: Y.S.P. Revising the manuscript critically for important intellectual content: K.W.S. Final approval of the version to be submitted: K.W.S.
References
1. Yoshida N, Ito T, Karasawa T, Itoh Z. AS-4370, a new gastrokinetic agent, enhances upper gastrointestinal motor activity in conscious dogs. J Pharmacol Exp Ther. 1991; 257:781–787.
2. Curran MP, Robinson DM. Mosapride in gastrointestinal disorders. Drugs. 2008; 68:981–991.
3. Yoshikawa T, Yoshida N, Mine Y, Hosoki K. Affinity of mosapride citrate, a new gastroprokinetic agent, for 5-HT4 receptors in guinea pig ileum. Jpn J Pharmacol. 1998; 77:53–59.
4. Wade PR, Mawe GM, Branchek TA, Gershon MD. Use of stereoisomers of zacopride to analyze actions of 5-hydroxytryptamine on enteric neurons. Am J Physiol. 1991; 260(1 Pt 1):G80–G90.


5. Nemeth PR, Ort CA, Zafirov DH, Wood JD. Interactions between serotonin and cisapride on myenteric neurons. Eur J Pharmacol. 1985; 108:77–83.


6. Nemeth PR, Gullikson GW. Gastrointestinal motility stimulating drugs and 5-HT receptors on myenteric neurons. Eur J Pharmacol. 1989; 166:387–391.


7. Wu ZS, Cheng H, Jiang Y, Melcher K, Xu HE. Ion channels gated by acetylcholine and serotonin: structures, biology, and drug discovery. Acta Pharmacol Sin. 2015; 36:895–907.


8. Sugita S, Shen KZ, North RA. 5-hydroxytryptamine is a fast excitatory transmitter at 5-HT3 receptors in rat amygdala. Neuron. 1992; 8:199–203.
9. Morales M, Battenberg E, de Lecea L, Sanna PP, Bloom FE. Cellular and subcellular immunolocalization of the type 3 serotonin receptor in the rat central nervous system. Brain Res Mol Brain Res. 1996; 36:251–260.


10. Holbrook JD, Gill CH, Zebda N, Spencer JP, Leyland R, Rance KH, Trinh H, Balmer G, Kelly FM, Yusaf SP, Courtenay N, Luck J, Rhodes A, Modha S, Moore SE, Sanger GJ, Gunthorpe MJ. Characterisation of 5-HT3C, 5-HT3D and 5-HT3E receptor subunits: evolution, distribution and function. J Neurochem. 2009; 108:384–396.
11. Miyake A, Mochizuki S, Takemoto Y, Akuzawa S. Molecular cloning of human 5-hydroxytryptamine3 receptor: heterogeneity in distribution and function among species. Mol Pharmacol. 1995; 48:407–416.
12. Akuzawa S, Miyake A, Miyata K, Fukutomi H. Comparison of [3H] YM060 binding to native and cloned rat 5-HT3 receptors. Eur J Pharmacol. 1996; 296:227–230.
13. Walstab J, Rappold G, Niesler B. 5-HT3 receptors: role in disease and target of drugs. Pharmacol Ther. 2010; 128:146–169.
14. Thompson AJ. Recent developments in 5-HT3 receptor pharmacology. Trends Pharmacol Sci. 2013; 34:100–109.
15. Fakhfouri G, Rahimian R, Ghia JE, Khan WI, Dehpour AR. Impact of 5-HT3 receptor antagonists on peripheral and central diseases. Drug Discov Today. 2012; 17:741–747.
16. Machu TK. Therapeutics of 5-HT3 receptor antagonists: current uses and future directions. Pharmacol Ther. 2011; 130:338–347.
17. Zheng Y, Yu T, Tang Y, Xiong W, Shen X, Jiang L, Lin L. Efficacy and safety of 5-hydroxytryptamine 3 receptor antagonists in irritable bowel syndrome: a systematic review and meta-analysis of randomized controlled trials. PLoS One. 2017; 12:e0172846.


18. Park YS, Myeong SH, Kim IB, Sung KW. Tricyclic antidepressant amitriptyline inhibits 5-hydroxytryptamine 3 receptor currents in NCB-20 cells. Korean J Physiol Pharmacol. 2018; 22:585–595.


19. Snyders D, Hondeghem L, Bennett P. Mechanisms of drug-channel interaction. In : Fozzard HA, Haber E, Jennings RB, Katz AM, Morgan HE, editors. The heart and cardiovascular system: scientific foundations, vol. 2. New York: Raven Press;1992.
20. Zhou Q, Verdoorn TA, Lovinger DM. Alcohols potentiate the function of 5-HT3 receptor-channels on NCB-20 neuroblastoma cells by favouring and stabilizing the open channel state. J Physiol. 1998; 507 (Pt 2):335–352.
21. van Hooft JA, van der Haar E, Vijverberg HP. Allosteric potentiation of the 5-HT3 receptor-mediated ion current in N1E-115 neuroblastoma cells by 5-hydroxyindole and analogues. Neuropharmacology. 1997; 36:649–653.
22. Neijt HC, Plomp JJ, Vijverberg HP. Kinetics of the membrane current mediated by serotonin 5-HT3 receptors in cultured mouse neuroblastoma cells. J Physiol. 1989; 411:257–269.
23. Gunthorpe MJ, Lummis SC. Diltiazem causes open channel block of recombinant 5-HT3 receptors. J Physiol. 1999; 519 Pt 3:713–722.
24. Nayak SV, Rondé P, Spier AD, Lummis SC, Nichols RA. Calcium changes induced by presynaptic 5-hydroxytryptamine-3 serotonin receptors on isolated terminals from various regions of the rat brain. Neuroscience. 1999; 91:107–117.


25. Thompson AJ, Lummis SC. 5-HT3 receptors. Curr Pharm Des. 2006; 12:3615–3630.
26. Gershon MD, Tack J. The serotonin signaling system: from basic understanding to drug development for functional GI disorders. Gastroenterology. 2007; 132:397–414.


27. Gregory RE, Ettinger DS. 5-HT3 receptor antagonists for the prevention of chemotherapy-induced nausea and vomiting A comparison of their pharmacology and clinical efficacy. Drugs. 1998; 55:173–189.
28. Galligan JJ, LePard KJ, Schneider DA, Zhou X. Multiple mechanisms of fast excitatory synaptic transmission in the enteric nervous system. J Auton Nerv Syst. 2000; 81:97–103.


29. Mawe GM, Hoffman JM. Serotonin signalling in the gut--functions, dysfunctions and therapeutic targets. Nat Rev Gastroenterol Hepatol. 2013; 10:473–486.


30. Longstreth GF, Thompson WG, Chey WD, Houghton LA, Mearin F, Spiller RC. Functional bowel disorders. Gastroenterology. 2006; 130:1480–1491.


31. Lazaraki G, Chatzimavroudis G, Katsinelos P. Recent advances in pharmacological treatment of irritable bowel syndrome. World J Gastroenterol. 2014; 20:8867–8885.
SUPPLEMENTARY MATERIALS
Supplementary data including two figures can be found with this article online at http://pdf.medrang.co.kr/paper/pdf/Kjpp/Kjpp2019-23-05-15-s001.pdf.
Supplementary Fig. 1
Current-voltage (I–V) relationship of mosapride on 5-hydroxytryptamine (5-HT)3 receptor current inhibition. (A) Representative traces of 5-HT3 receptor currents induced by 3 µM 5-HT alone at holding potentials of −50–+30 mV (left) and 5-HT3 receptor currents induced by the co-application of 3 µM 5-HT with 10 µM mosapride at the same holding potentials (right). The open horizontal bars indicate the drug application period. (B) I–V plots of averaged normalized peak amplitudes induced by 5-HT with (●) or without (○) mosapride. The reversal potentials were 7.05 ± 0.37 mV (n = 11) for 5-HT alone, and 7.75 ± 0.53 mV (n = 11) for 5-HT with mosapride. The reversal potential was not significantly changed by co-application of mosapride (p = 0.2964, unpaired t-test). (C) Fractional block of mosapride (ratio of peak amplitude of 5-HT with mosapride / peak amplitude of 5-HT alone) as a function of the holding potentials (VHolding). The data are expressed as means ± standard error of the mean.
Supplementary Fig. 2
Use-dependency of mosapride inhibition on the 5-hydroxytryptamine (5-HT)3 receptor current. (A) Representative traces of 5-HT3 receptor currents induced by 10 µM 5-HT alone (upper), and currents induced by the co-application of 10 µM 5-HT with 10 µM mosapride (lower), for 2 sec at 30 sec interval, 10 times. Mosapride co-application was started at the fourth trace and stopped at the seventh trace, indicated by gray color traces. (B) Averaged plots of normalized peak amplitudes induced by 5-HT with (●) or without (○) mosapride versus the time. The ratio of the peak amplitudes of seventh trace (the last mosapride application) to the fourth peak amplitude (the first of mosapride application) was compared to analyze the use-dependency drug effects. There was no significant difference in the ratio between the application of 5-HT alone (n = 9, 1.01 ± 0.01) and the co-application of 5-HT and mosapride (n = 9, 0.99 ± 0.02) (p = 0.7341, unpaired t-test). The data are expressed as means ± standard error of the mean.