Abstract
Background/Aims
Pancreatic cancer has a very poor prognosis, and early diagnosis is a way to increase the survival rate of patients. The purpose of this study was to develop pancreatic cancer-specific peptides for imaging studies.
Methods
Three pancreatic cancer cell lines, MIA PaCa-2, UACC-462, and BxPC-3, and a control cell line, CCD841, were used. Biopannings were performed on MIA PaCa-2 using a phage display library. After this, the peptides were synthesized and labeled with fluorescein isothiocyanate (FITC). Immunocytochemistry (ICC), enzyme-linked immunosorbent assay (ELISA), and fluorescence-activated cell sorter (FACS) were performed to examine the specific binding. To examine its therapeutic applications, a photosensitizer, chlorin e6 (Ce6), was conjugated on the peptide and photodynamic therapy was performed. Cell survival was investigated using a [3-(4,5-Dimethylthiazol-2-yl)-2,5-Diphenyltetrazolium Bromide] assay.
Results
After three biopannings, the phages were amplified from 1.4×104 to 3.2×105 plaque-forming units. The most strongly binding phage was selected from the ELISA and ICC results. FITC-labeled peptide, M5, in the three pancreatic cancer cell lines showed significantly higher immunofluorescence in the ICC experiments than that of CCD841. The higher binding ability to MIA PaCa-2 cells was confirmed from FACS analysis, which showed a right shift compared to CCD841. M5 bound to Ce6 showed a significantly lower cell survival rate than that of Ce6 alone in photodynamic therapy, which was observed consistently as a change in the tumor size and fluorescence intensity in MIA PaCa-2 cell-implanted animal models.
Figures and Tables
Fig. 1
ELISA assays were performed to select a phage that adhered specifically to MIA PaCa-2 cells among nine randomly selected phage candidate groups. ELISA, enzyme-linked immunosorbent assay.
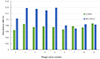
Fig. 2
Immunohistochemical staining was performed using Alexa 488 to confirm the binding affinity of the phage clone to pancreatic cancer (A) phage 2; (B) phage 3; (C) phage 4; (D) phage 5. The binding site of the phage expresses as green fluorescence; and (E) CCD841, MIA PaCa-2, UACC-462, and BxPC-3 cells, which were subjected to immunofluorescence staining to confirm the cell binding ability of the M5 peptide. The M5 peptide was labeled with FITC, and the cells were stained with DAPI (Magnification ×200). FITC, fluorescein isothiocyanate; DAPI, 4′,6-diamidino-2-phenylindole.
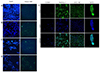
Fig. 3
Structure of the peptide and peptide HPLC profile. (A) Structure of the M5 peptide, (B) structure of the peptide M5 coupled with the photosensitizer Ce6, (C) HPLC analysis of peptide M5, (D) HPLC analysis of peptide M5 and the Ce6 conjugate peptide. Ce6, chlorin e6; HPLC, high-performance liquid chromatography.
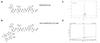
Fig. 4
(A–D) Fluorescence activated cell sorter of the CCD841 and MIA PaCa-2, UACC-462, BxPC-3 cells bound with the control and M5 peptide: blue line-control, and red line-M5 peptide. FITC, fluorescein isothiocyanate.
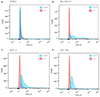
Fig. 5
Cellular uptake of the photosensitizer, Ce6, in the Ce6-treated group, in which the Ce6-treated group and M5-peptide were combined, was confirmed in the control cell CCD841 and the pancreatic cancer cell line MIA PaCa-2. 4 µg/mL and 12 µg/mL, respectively. (A) CCD841 cell Ce6 alone and M5-Ce6 treatment group, (B) MIA PaCa-2 cell Ce6 alone and M5-Ce6 treatment group. DAPI, 4′,6-diamidino-2-phenylindole; Ce6, chlorin e6.
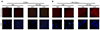
Fig. 6
MTT cytotoxicity assay confirmed the laser irradiation effect of the M5 peptide and M5-Ce6. (A) Laser irradiation in CCD841 cells, (B) laser non-irradiation in CCD841 cells. (C) Laser irradiation in MIA PaCa-2 cells, (D) laser non-irradiation in MIA PaCa-2 cells. Ce6, chlorin e6; MTT, 3-(4,5-Dimethylthiazol-2-yl)-2,5-Diphenyltetrazolium Bromide.
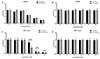
Fig. 7
In vivo study using nude mice implanted with MIA PaCa-2 cells. (A) Comparison of the fluorescence intensity between chlorin e6 (Ce6) group (left) and M5-conjugated chlorin e6 (M5-Ce6) group (right) by the optical in vivo imaging system-IVIS. Ce6 and M5-Ce6 were injected into the tumors with a volume of 150 µL (4 µM). In vivo fluorescence just after the injection of the photosensitizers (middle) and after one hour (bottom). (B) Fluorescence intensity ratio of the tumors between the two groups (each n=5). (C) Change in tumor size before and 48 hours after photodynamic therapy of the two groups (each n=3). (D) Microscopic findings. M5-Ce6 group showed more necrosis (right top, H&E, ×100) than the Ce6-pretreated group (left top, H&E, ×100). Cleaved caspase-3 positive cells were also observed abundantly in the M5-Ce6 group (right bottom, Cleaved Cas-3, ×100) than the Ce6 group (left bottom, Cleaved Cas-3, ×100).
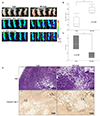
Notes
Financial support This research was supported by the Basic Science Research Program through the National Research Foundation of Korea, funded by the Ministry of Education, Science and Technology (NRF-2017R1D1A1B03035104) and Research Fund of Seoul St. Mary's Hospital, The Catholic University of Korea.
References
2. Ryu JK. The early detection of pancreatic cancer: whom and how? Korean J Pancreas Biliary Tract. 2015; 20:198–203.


4. Fong ZV, Tan WP, Lavu H, et al. Preoperative imaging for resectable periampullary cancer: clinicopathologic implications of reported radiographic findings. J Gastrointest Surg. 2013; 17:1098–1106.


5. Pannala R, Basu A, Petersen GM, Chari ST. New-onset diabetes: a potential clue to the early diagnosis of pancreatic cancer. Lancet Oncol. 2009; 10:88–95.


6. Kozuka S, Sassa R, Taki T, et al. Relation of pancreatic duct hyperplasia to carcinoma. Cancer. 1979; 43:1418–1428.


7. Lee JH, Cassani LS, Bhosale P, Ross WA. The endoscopist's role in the diagnosis and management of pancreatic cancer. Expert Rev Gastroenterol Hepatol. 2016; 10:1027–1039.


8. Chu LC, Goggins MG, Fishman EK. Diagnosis and detection of pancreatic cancer. Cancer J. 2017; 23:333–342.


9. Goonetilleke KS, Siriwardena AK. Systematic review of carbohydrate antigen (CA 19-9) as a biochemical marker in the diagnosis of pancreatic cancer. Eur J Surg Oncol. 2007; 33:266–270.


10. Bausch D, Thomas S, Mino-Kenudson M, et al. Plectin-1 as a novel biomarker for pancreatic cancer. Clin Cancer Res. 2011; 17:302–309.


11. Faca VM, Song KS, Wang H, et al. A mouse to human search for plasma proteome changes associated with pancreatic tumor development. PLoS Med. 2008; 5:e123.


12. Koopmann J, Fedarko NS, Jain A, et al. Evaluation of osteopontin as biomarker for pancreatic adenocarcinoma. Cancer Epidemiol Biomarkers Prev. 2004; 13:487–491.


13. Sidhu SS. Phage display in pharmaceutical biotechnology. Curr Opin Biotechnol. 2000; 11:610–616.


14. Wilson DR, Finlay BB. Phage display: applications, innovations, and issues in phage and host biology. Can J Microbiol. 1998; 44:313–329.


15. Rodi DJ, Makowski L. Phage-display technology--finding a needle in a vast molecular haystack. Curr Opin Biotechnol. 1999; 10:87–93.
16. Frei JC, Lai JR. Protein and antibody engineering by phage display. Methods Enzymol. 2016; 580:45–87.


17. Joshi BP, Wang TD. Targeted optical imaging agents in cancer: focus on clinical applications. Contrast Media Mol Imaging. 2018; 2018:2015237.


18. Kaminski MF, Regula J, Kraszewska E, et al. Quality indicators for colonoscopy and the risk of interval cancer. N Engl J Med. 2010; 362:1795–1803.


19. Hsiung PL, Hardy J, Friedland S, et al. Detection of colonic dysplasia in vivo using a targeted heptapeptide and confocal microendoscopy. Nat Med. 2008; 14:454–458.


20. Gu YL, Lan C, Pei H, Yang SN, Liu YF, Xiao LL. Applicative value of serum CA19-9, CEA, CA125 and CA242 in diagnosis and prognosis for patients with pancreatic cancer treated by concurrent chemoradiotherapy. Asian Pac J Cancer Prev. 2015; 16:6569–6573.


21. Honda K, Katzke VA, Hüsing A, et al. CA19-9 and apolipoprotein-A2 isoforms as detection markers for pancreatic cancer: a prospective evaluation. Int J Cancer. 2019; 144:1877–1887.
22. DeWitt J, Devereaux B, Chriswell M, et al. Comparison of endoscopic ultrasonography and multidetector computed tomography for detecting and staging pancreatic cancer. Ann Intern Med. 2004; 141:753–763.


23. Joshi BP, Dai Z, Gao Z, et al. Detection of sessile serrated adenomas in the proximal colon using wide-field fluorescence endoscopy. Gastroenterology. 2017; 152:1002–1013.e9.


24. Bang JY, Magee SH, Ramesh J, Trevino JM, Varadarajulu S. Randomized trial comparing fanning with standard technique for endoscopic ultrasound-guided fine-needle aspiration of solid pancreatic mass lesions. Endoscopy. 2013; 45:445–450.


25. Bang S. Surveillance and early diagnosis of pancreatic cancer. Korean J Pancreas Biliary Tract. 2015; 20:1–4.


26. Takaishi S, Okumura T, Tu S, et al. Identification of gastric cancer stem cells using the cell surface marker CD44. Stem Cells. 2009; 27:1006–1020.


27. Moris M, Dawson DW, Jiang J, et al. Plectin-1 as a biomarker of malignant progression in intraductal papillary mucinous neoplasms: a multicenter study. Pancreas. 2016; 45:1353–1358.
28. Wang J, Zheng M, Xie Z. Carrier-free core-shell nanodrugs for synergistic two-photon photodynamic therapy of cervical cancer. J Colloid Interface Sci. 2019; 535:84–91.


29. Zullo SW, Zeitouni NC, Segal RJ. Long-term efficacy of combination vismodegib and photodynamic therapy for multiple basal cell carcinomas. Photodiagnosis Photodyn Ther. 2018; 24:164–165.


30. Lee KH. Chemotherapy and targeted therapy with management of related complications in pancreatic cancer. Korean J Pancreas Biliary Tract. 2015; 20:5–13.


31. Moore MJ, Goldstein D, Hamm J, et al. Erlotinib plus gemcitabine compared with gemcitabine alone in patients with advanced pancreatic cancer: a phase III trial of the National Cancer Institute of Canada Clinical Trials Group. J Clin Oncol. 2007; 25:1960–1966.


32. Neoptolemos JP, Stocken DD, Friess H, et al. A randomized trial of chemoradiotherapy and chemotherapy after resection of pancreatic cancer. N Engl J Med. 2004; 350:1200–1210.


33. Yabar CS, Winter JM. Pancreatic cancer: a review. Gastroenterol Clin North Am. 2016; 45:429–445.
34. Huggett MT, Jermyn M, Gillams A, et al. Phase I/II study of verteporfin photodynamic therapy in locally advanced pancreatic cancer. Br J Cancer. 2014; 110:1698–1704.


35. Bown SG, Rogowska AZ, Whitelaw DE, et al. Photodynamic therapy for cancer of the pancreas. Gut. 2002; 50:549–557.


36. Choi JH, Oh D, Lee JH, et al. Initial human experience of endoscopic ultrasound-guided photodynamic therapy with a novel photosensitizer and a flexible laser-light catheter. Endoscopy. 2015; 47:1035–1038.

