Abstract
Purpose
We investigate biases in the assessments of left ventricular function (LVF), by compressed sensing (CS)-cine magnetic resonance imaging (MRI).
Materials and Methods
Cardiovascular cine images with short axis view, were obtained for 8 volunteers without CS. LVFs were assessed with subsampled data, with compression factors (CF) of 2, 3, 4, and 8. A semi-automatic segmentation program was used, for the assessment. The assessments by 3 CS methods (ITSC, FOCUSS, and view sharing (VS)), were compared to those without CS. Bland-Altman analysis and paired t-test were used, for comparison. In addition, real-time CS-cine imaging was also performed, with CF of 2, 3, 4, and 8 for the same volunteers. Assessments of LVF were similarly made, for CS data. A fixed compensation technique is suggested, to reduce the bias.
Results
The assessment of LVF by CS-cine, includes bias and random noise. Bias appeared much larger than random noise. Median of end-diastolic volume (EDV) with CS-cine (ITSC or FOCUSS) appeared −1.4% to −7.1% smaller, compared to that of standard cine, depending on CF from (2 to 8). End-systolic volume (ESV) appeared +1.6% to +14.3% larger, stroke volume (SV), −2.4% to −16.4% smaller, and ejection fraction (EF), −1.1% to −9.2% smaller, with P < 0.05. Bias was reduced from −5.6% to −1.8% for EF, by compensation applied to real-time CS-cine (CF = 8).
Conclusion
Loss of temporal resolution by adopting missing data from nearby cardiac frames, causes an underestimation for EDV, and an overestimation for ESV, resulting in underestimations for SV and EF. The bias is not random. Thus it should be removed or reduced for better diagnosis. A fixed compensation is suggested, to reduce bias in the assessment of LVF.
References
1. Lamb HJ, Doornbos J, van der Velde EA, Kruit MC, Reiber JH, de Roos A. Echo planar MRI of the heart on a standard system: validation of measurements of left ventricular function and mass. J Comput Assist Tomogr. 1996; 20:942–949.


5. Lustig M, Donoho D, Pauly JM. Sparse MRI: The application of compressed sensing for rapid MR imaging. Magn Reson Med. 2007; 58:1182–1195.


6. Candes EJ, Wakin MB. An introduction to compressive sampling. IEEE Signal Process Mag. 2008; 25:21–30.


7. Pruessmann KP, Weiger M, Scheidegger MB, Boesiger P. SENSE: sensitivity encoding for fast MRI. Magn Reson Med. 1999; 42:952–962.


8. Liang D, Liu B, Wang J, Ying L. Accelerating SENSE using compressed sensing. Magn Reson Med. 2009; 62:1574–1584.


9. Otazo R, Kim D, Axel L, Sodickson DK. Combination of compressed sensing and parallel imaging for highly accelerated first-pass cardiac perfusion MRI. Magn Reson Med. 2010; 64:767–776.


10. Feng L, Grimm R, Block KT, et al. Golden-angle radial sparse parallel MRI: combination of compressed sensing, parallel imaging, and golden-angle radial sampling for fast and flexible dynamic volumetric MRI. Magn Reson Med. 2014; 72:707–717.


11. Benkert T, Feng L, Sodickson DK, Chandarana H, Block KT. Free-breathing volumetric fat/water separation by combining radial sampling, compressed sensing, and parallel imaging. Magn Reson Med. 2017; 78:565–576.


12. Park J, Hong HJ, Yang YJ, Ahn CB. Fast cardiac CINE MRI by iterative truncation of small transformed coefficients. Investig Magn Reson Imaging. 2015; 19:19–30.


13. Aurigemma G, Reichek N, Schiebler M, Axel L. Evaluation of aortic regurgitation by cardiac cine magnetic resonance imaging: planar analysis and comparison to Doppler echocardiography. Cardiology. 1991; 78:340–347.


14. Rodevan O, Bjornerheim R, Ljosland M, Maehle J, Smith HJ, Ihlen H. Left atrial volumes assessed by three- and two-dimensional echocardiography compared to MRI estimates. Int J Card Imaging. 1999; 15:397–410.
15. Bak SH, Kim SM, Park S, Kim M, Choe YH. Assessment of left ventricular function with single breathhold magnetic resonance cine imaging in patients with arrhythmia. Investig Magn Reson Imaging. 2017; 21:20–27.


16. Ngo TA, Lu Z, Carneiro G. Combining deep learning and level set for the automated segmentation of the left ventricle of the heart from cardiac cine magnetic resonance. Med Image Anal. 2017; 35:159–171.


17. White HD, Norris RM, Brown MA, Brandt PW, Whitlock RM, Wild CJ. Left ventricular end-systolic volume as the major determinant of survival after recovery from myocardial infarction. Circulation. 1987; 76:44–51.


18. Lorenz CH, Walker ES, Morgan VL, Klein SS, Graham TP Jr. Normal human right and left ventricular mass, systolic function, and gender differences by cine magnetic resonance imaging. J Cardiovasc Magn Reson. 1999; 1:7–21.


19. Wu E, Ortiz JT, Tejedor P, et al. Infarct size by contrast enhanced cardiac magnetic resonance is a stronger predictor of outcomes than left ventricular ejection fraction or end-systolic volume index: prospective cohort study. Heart. 2008; 94:730–736.


20. Muthurangu V, Lurz P, Critchely JD, Deanfield JE, Taylor AM, Hansen MS. Real-time assessment of right and left ventricular volumes and function in patients with congenital heart disease by using high spatiotemporal resolution radial k-t SENSE. Radiology. 2008; 248:782–791.


21. Miller S, Simonetti OP, Carr J, Kramer U, Finn JP. MR Imaging of the heart with cine true fast imaging with steady-state precession: influence of spatial and temporal resolutions on left ventricular functional parameters. Radiology. 2002; 223:263–269.


22. Hsiao A, Lustig M, Alley MT, et al. Rapid pediatric cardiac assessment of flow and ventricular volume with compressed sensing parallel imaging volumetric cine phase-contrast MRI. AJR Am J Roentgenol. 2012; 198:W250–259.


23. Goebel J, Nensa F, Schemuth HP, et al. Compressed sensing cine imaging with high spatial or high temporal resolution for analysis of left ventricular function. J Magn Reson Imaging. 2016; 44:366–374.


24. Vincenti G, Monney P, Chaptinel J, et al. Compressed sensing single-breathhold CMR for fast quantification of LV function, volumes, and mass. JACC Cardiovasc Imaging. 2014; 7:882–892.
25. Bassett EC, Kholmovski EG, Wilson BD, et al. Evaluation of highly accelerated realtime cardiac cine MRI in tachycardia. NMR Biomed. 2014; 27:175–182.


26. Lin ACW, Strugnell W, Riley R, et al. Higher resolution cine imaging with compressed sensing for accelerated clinical left ventricular evaluation. J Magn Reson Imaging. 2017; 45:1693–1699.


27. Kido T, Kido T, Nakamura M, et al. Compressed sensing realtime cine cardiovascular magnetic resonance: accurate assessment of left ventricular function in a single-breathhold. J Cardiovasc Magn Reson. 2016; 18:50.


28. Bluemke DA, Boxerman JL, Atalar E, McVeigh ER. Segmented K-space cine breathhold cardiovascular MR imaging: Part 1. Principles and technique. AJR Am J Roentgenol. 1997; 169:395–400.
29. Scheffler K, Lehnhardt S. Principles and applications of balanced SSFP techniques. Eur Radiol. 2003; 13:2409–2418.


30. Jung H, Sung K, Nayak KS, Kim EY, Ye JC. k-t FOCUSS: a general compressed sensing framework for high resolution dynamic MRI. Magn Reson Med. 2009; 61:103–116.


31. Markl M, Hennig J. Phase contrast MRI with improved temporal resolution by view sharing: k-space related velocity mapping properties. Magn Reson Imaging. 2001; 19:669–676.


32. Lang RM, Bierig M, Devereux RB, et al. Recommendations for chamber quantification: a report from the American Society of Echocardiography's Guidelines and Standards Committee and the Chamber Quantification Writing Group, developed in conjunction with the European Association of Echocardiography, a branch of the European Society of Cardiology. J Am Soc Echocardiogr. 2005; 18:1440–1463.


Fig. 1.
Reconstructions for CS and parallel imaging, are performed sequentially. First, (a) reconstruction for CS is performed, and folded image is obtained, and then (b) SENSE reconstruction is applied to the folded image, to obtain unfolded image. Only even phase encoding locations are used.

Fig. 2.
Normalized differences for EDV and ESV for 8 volunteers are depicted, as a function of CF for 3 CS methods. Mean and median of the normalized differences, are shown in broken and solid lines, respectively.
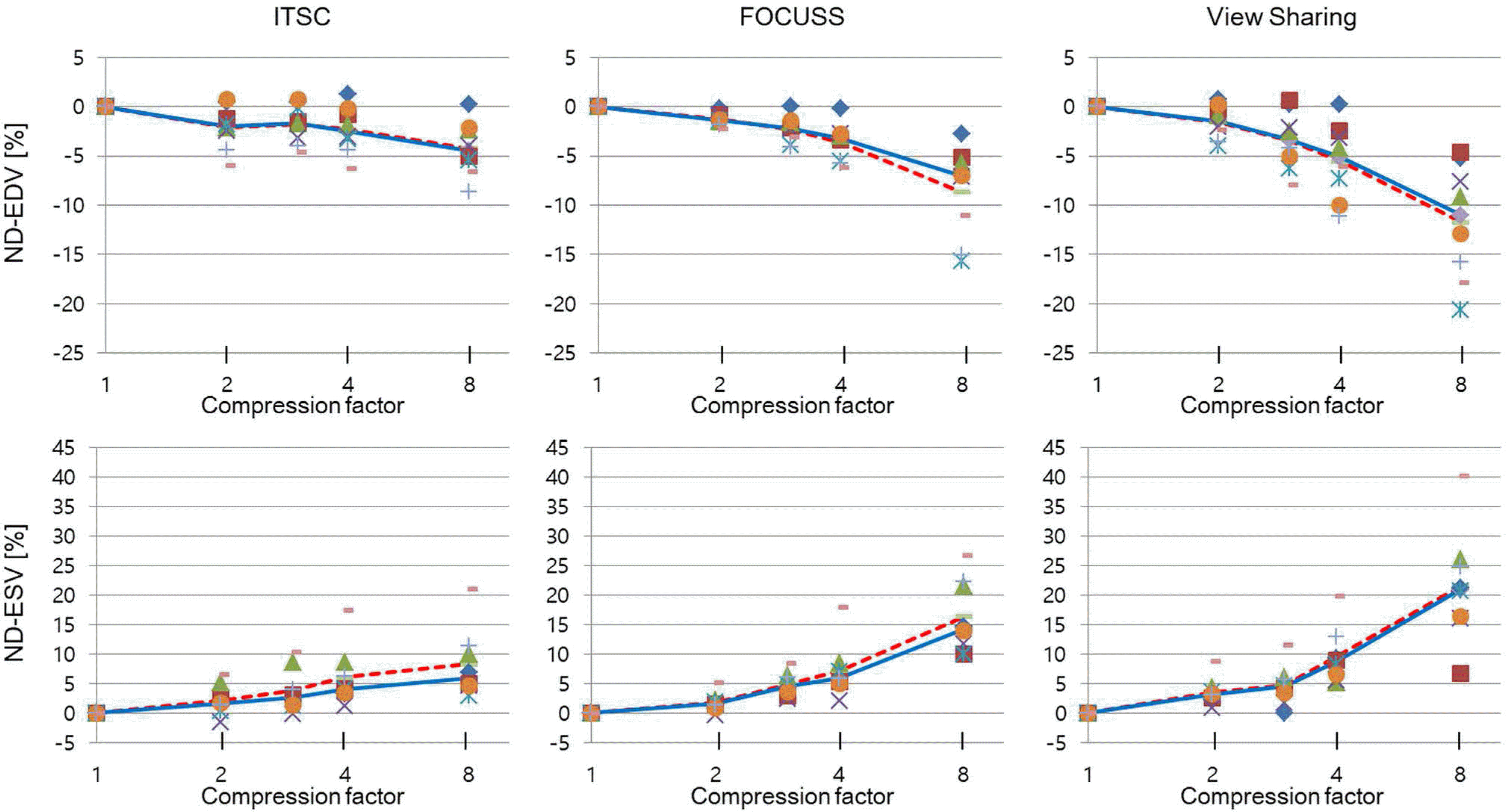
Fig. 3.
Normalized differences for SV and EF for 8 volunteers are shown, as a function of CF for 3 CS methods. Mean and median are shown in broken and solid lines.
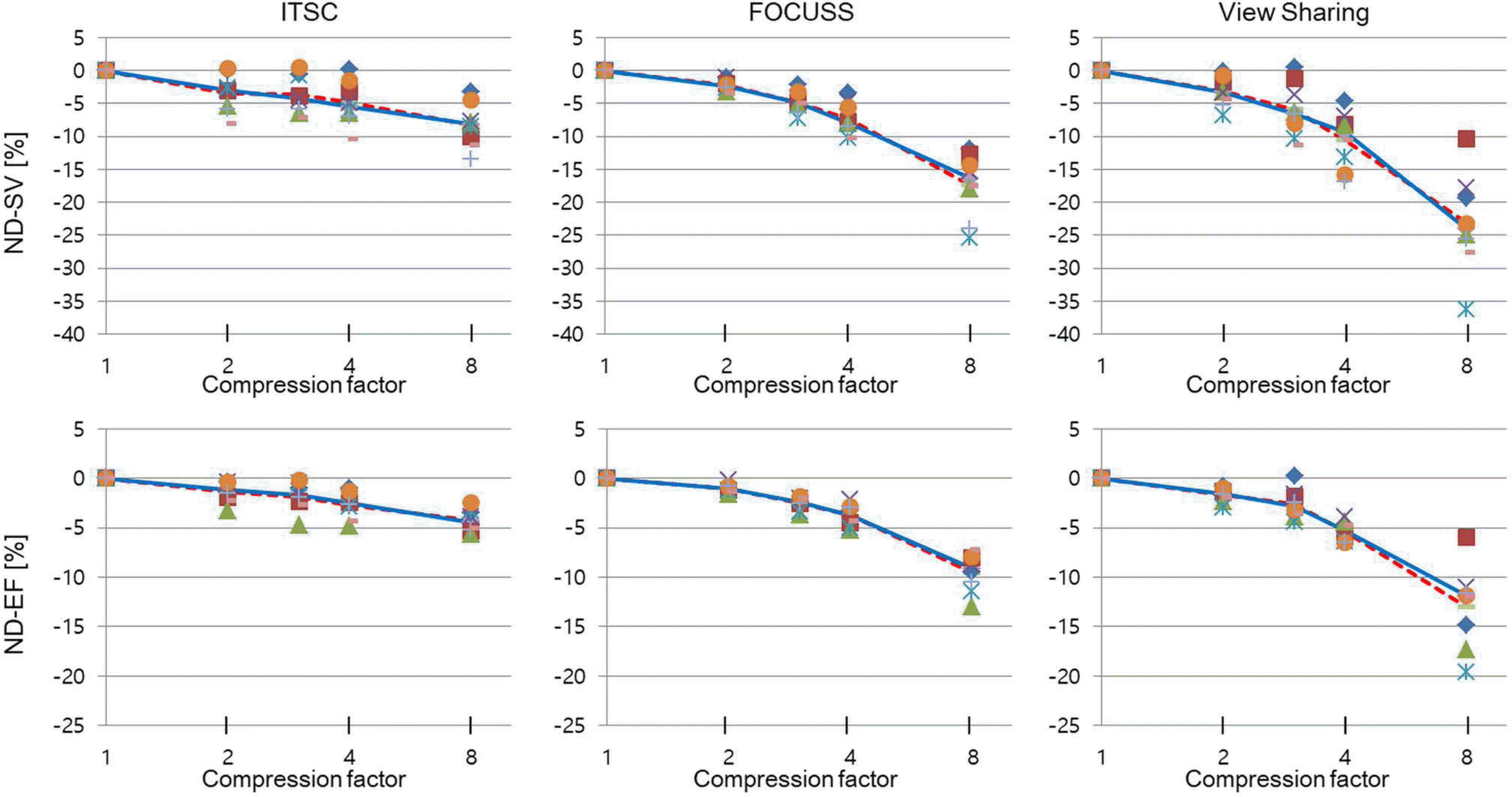
Fig. 4.
Bland-Altman plots for assessments of LVF by CS-cine (FOCUSS, CF = 4) and standard cine: (a) EDV, (b) ESV, (c) SV, and (d) EF. Mean of difference (‘Mean’) and range of 95% confidence interval of the mean difference (CIMD), are shown in blue solid and dashed lines. The 95% confidence interval of the difference (CID), is also shown in dark-brown dotted lines. P-value is shown next to the mean of difference. Significant biases (P < 0.005) are found for all EDV, ESV, SV, and EF.
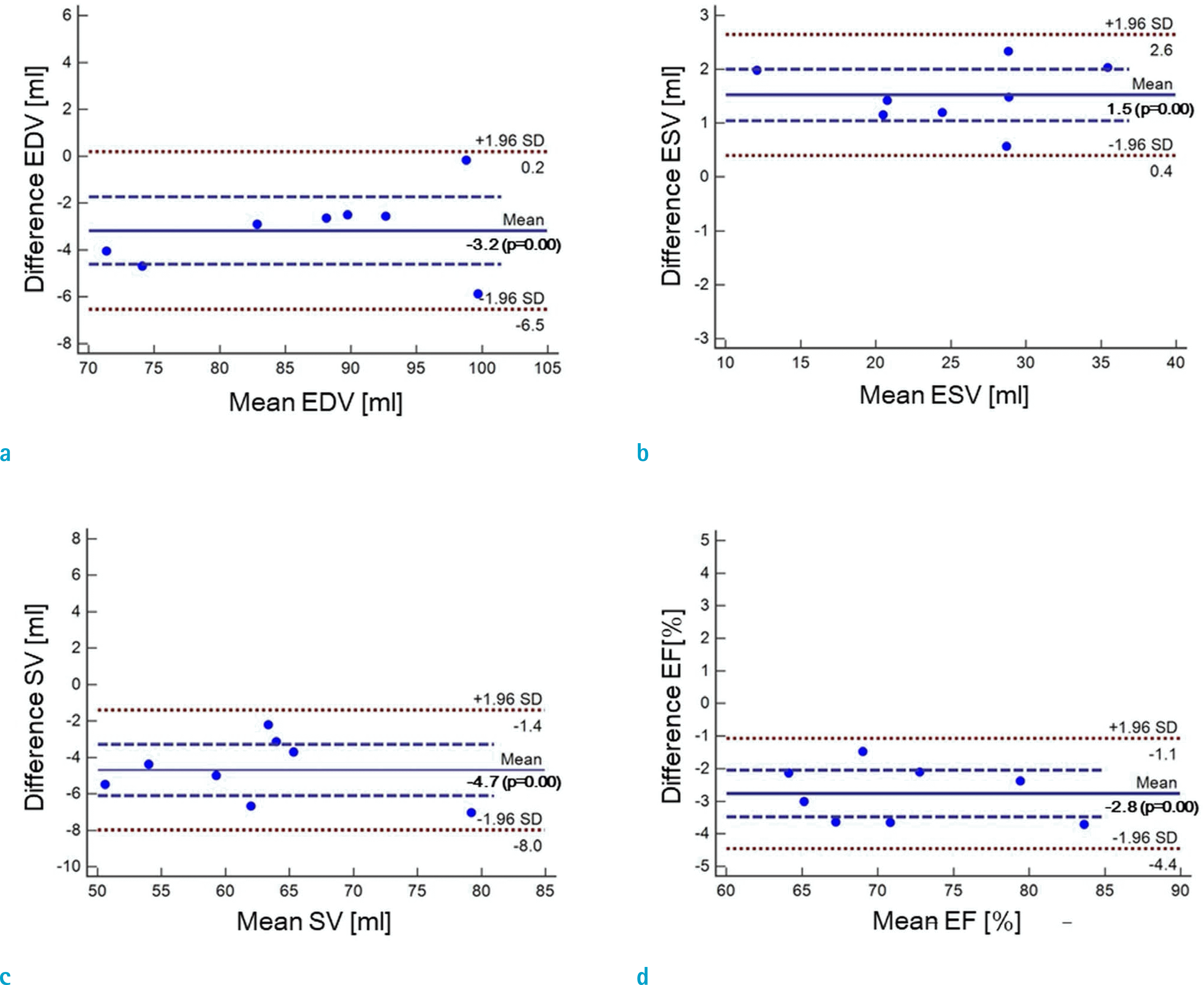
Fig. 5.
Bland-Altman plots for assessments of LVF by real time CS-cine (ITSC, CF = 8) and standard cine: (a) EDV, (b) ESV, (c) SV, and (d) EF. Significant biases are found for all EDV, ESV, SV, and EF (P < 0.02).
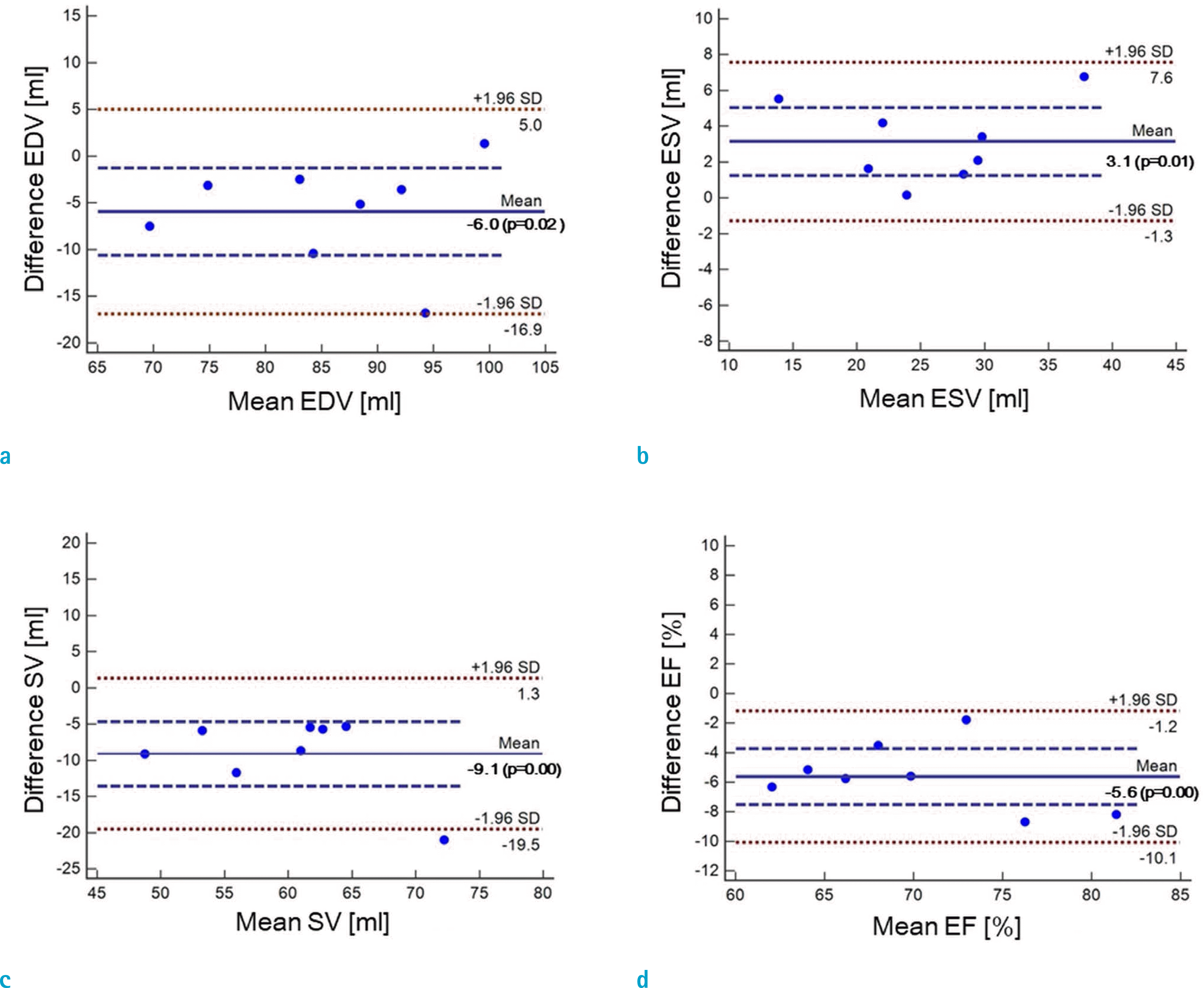
Fig. 6.
Bland-Altman plots for assessments of LVF by CS-cine (FOCUSS, CF = 4) and standard cine after compensation: (a) EDV, (b) ESV, (c) SV, and (d) EF. As shown, biases are reduced substantially for all EDV, ESV, SV, and EF.
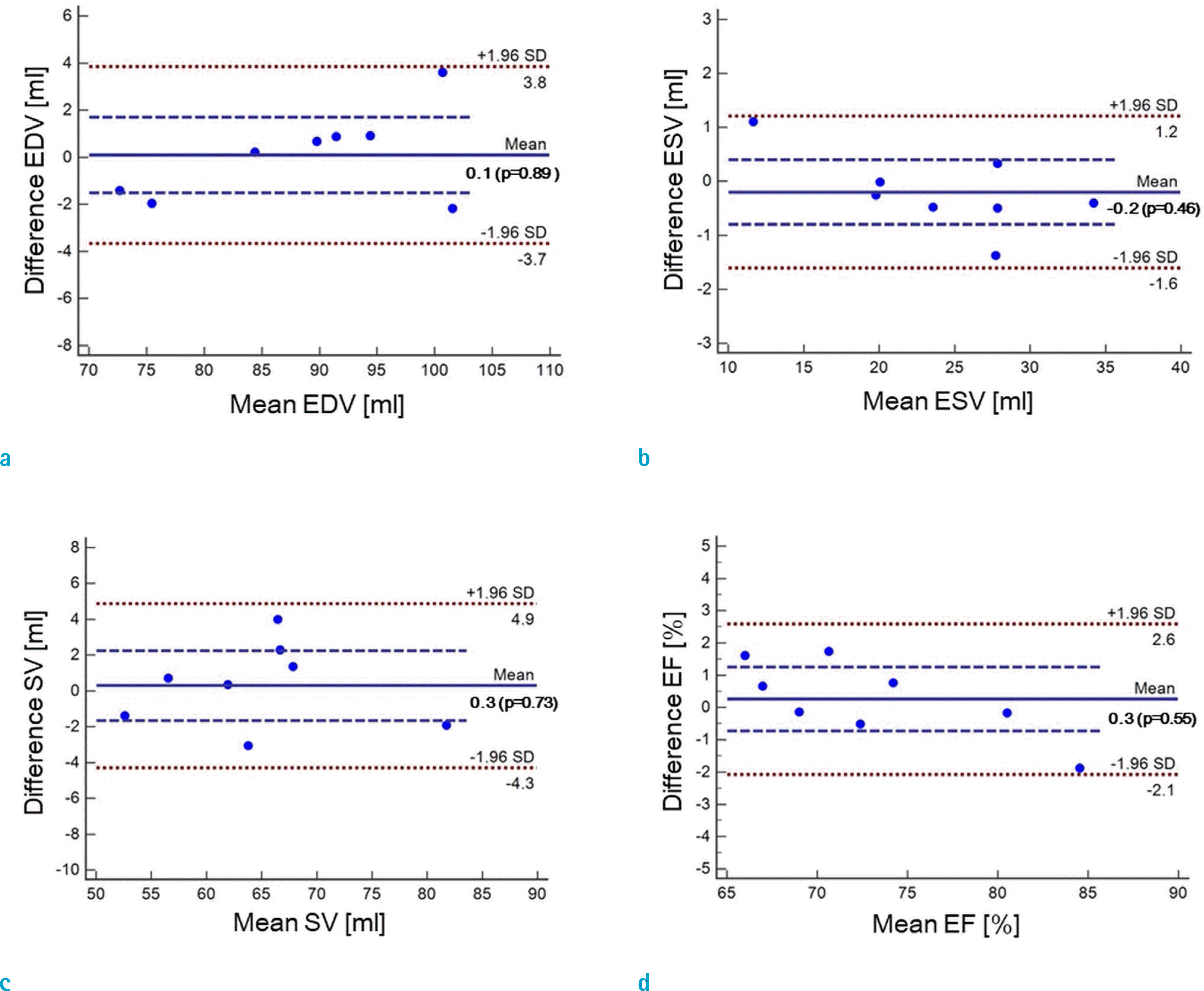
Fig. 7.
Bland-Altman plots for assessments of LVF by real-time CS-cine (ITSC, CF = 8) and standard cine after compensation: (a) EDV, (b) ESV, (c) SV, and (d) EF.
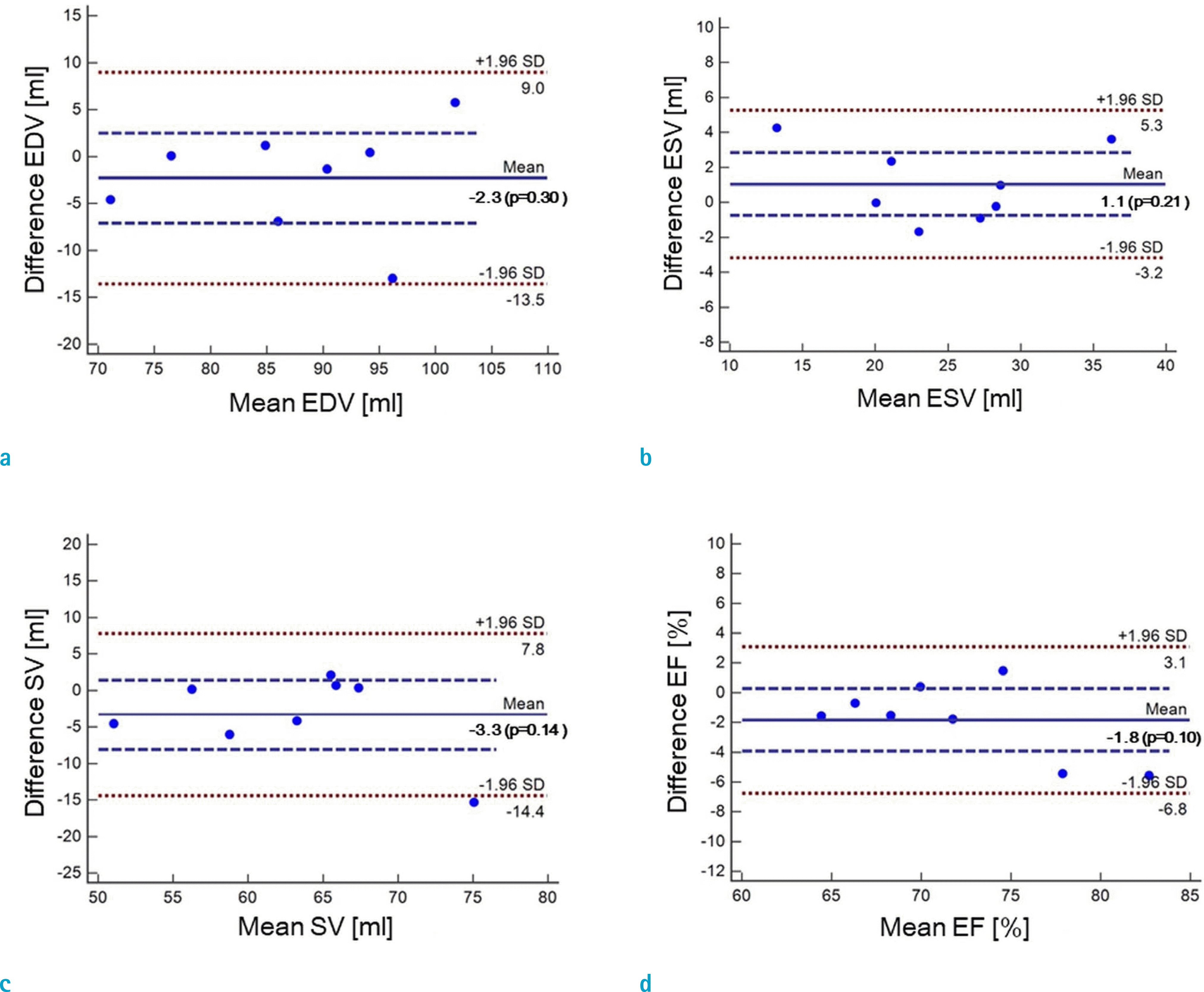
Table 1.
The Median, Mean, SD, and P Values of the Normalized Differences of LVF for 8 Volunteers are Summarized for 3 CS-cine Methods
CF = compression factor; CS = compressed sensing; EDV = end-diastolic volume; EF = ejection fraction; ESV = end-systolic volume; FOCUSS = FOCal Underdetermined System Solver; LVF = left ventricular function; ITSC = Iterative Truncation of Small transformed Coefficients; SD = standard deviation; SV = stroke volume; VS = view sharing