Abstract
Objective
The aim of this study was to evaluate the amount of tooth movement and histologic changes with different corticotomy designs and micro-osteoperforation in rabbits.
Methods
The sample consisted of 24
rabbits divided into three experimental groups (triangular corticotomy [TC] and indentation corticotomy [IC] with flap, and flapless micro-osteoperforations [MP]) and a control. A traction force of 100 cN was applied by connecting the first premolars to the incisors. The amount of tooth movement was measured. Kruskal-Wallis test was used to assess differences in tooth movement between the groups. Micro-computed tomography, hematoxylin and eosin staining, and tartrate-resistant acidic phosphatase (TRAP) analysis were performed. Analysis of variance was applied to assess differences in TRAP-positive osteoclast count between the groups.
Acceleration of orthodontic tooth movement is an increasing need, especially considering the faster pace of life and the increasing demands from adult orthodontics. Several modalities123 have been suggested to increase the rate of tooth movement, of which surgical techniques have gained much attention. The rationale underlying the surgical acceleration of tooth movement is the rapid acceleratory phenomenon (RAP) that occurs at the injury site. RAP involves recruitment of osteoclasts and osteoblasts to the injury site to assist wound healing. This results in a transient localized demineralization-remineralization in the bone involved in the injury process. The combined effect of the two processes is claimed to result in accelerated tooth movement because the resistance of the dense cortical bone to orthodontic tooth movement is eliminated.456
Corticotomy—the intentional cutting of cortical bone—has long been used to accelerate tooth movement.789 Corticotomy originally involved buccal and lingual flap reflections followed by bone decortication. Several modifications were performed to the procedures, wherein one-side flap reflection was adopted. The cortical cuts were in the form of perforations, vertical cuts, or combinations of both. Other studies have reported less invasive flapless techniques such as micro-osteoperforation and computer-guided corticotomy.101112131415 Several authors have confirmed that micro-osteoperforation significantly reduced the duration of orthodontic treatment.1314 However, animal studies showed that the effects of flapless perforations on the rate of tooth movement were inconsistent.1415 Therefore, different designs for micro-osteoperforation were suggested to accelerate tooth movement.
Severe atrophy of the alveolar ridge due to tooth loss is a major obstacle to tooth movement. Molar protraction to close the edentulous space of an adjacent extracted tooth may be a viable treatment option, mitigating the future restorative costs of alternative procedures. However, previous studies reported that the increased thickness of the cortical bone in these atrophied areas makes root parallelism difficult to achieve, especially in the mandible. It may also decrease tooth movement, resulting in tipping instead of bodily movement,1617 or may even lead to buccal and lingual bone dehiscence with molar protraction.18
Recently, Kook et al.7 summarized three methods of corticotomy to facilitate mandibular molar protraction in atrophied alveolar ridges. They reported circumscribed design involving only a limited surgical area of the target tooth.
The proper design and the extent of bone injury required to achieve the desired tooth movement still remains an unsolved dilemma. No studies have compared the effects of corticotomy versus micro-osteoperforation and their associated biological responses in homogenous animal models.
The aim of this study was to evaluate the amount of tooth movement and histologic changes with different corticotomy designs and micro-osteoperforations in rabbits.
The sample consisted of right and left mandibular halves of 24 female New Zealand white rabbits, weighing 3.6 to 4.3 kg. A total of 48 mandibular halves were randomly divided into three experimental and one control groups. The animal protocol was approved by Uijeongbu St. Mary's Hospital, Catholic University of Korea-Institutional Animal Care (UJA2015-12A).
Three different types of interventions were performed mesial to the mandibular first premolar (PM): triangular corticotomy (TC), indentation corticotomy (IC), and micro-osteoperforation (MP) (Figure 1).
In the TC group, a 1.0-mm deep, 3.0-mm wide, 5.0-mm high bony defect was created after flap elevation using a 1-mm round bur. In the IC group, three indentations of 1.0-mm depth and 1.0-mm diameter were created 1-mm apart using a 0.8-mm round bur after flap elevation. In the MP group, two micro-osteoperforations with a diameter of 1.4 mm and depth of 3 mm were performed 2 mm apart through the gingiva with micro-screws (diameter 1.4 mm and length 10 mm, 14-G2-006, Dual Top Anchor System; Jeil Medical, Seoul, Korea). Traction force of 100 cN was applied bilaterally with nickel-titanium closed coil springs (Jin Sung Corp., Seoul, Korea) between the PMs and anterior teeth for 4 weeks. At the end of the fourth week, the rabbits were sacrificed under deep anesthesia with ketamine hydrochloride (Yuhan, Seoul, Korea).
Mandibular dental models of all rabbits were made from elastomeric impressions taken immediately after sacrifice. The amount of tooth movement was measured using Adobe Photoshop software (Adobe Systems Inc., San Jose, CA, USA) from the center of the distal surface of the first PM to the center of the mesial surface of the second PM on standardized photographs of the dental models with a ruler (to scale the photo into a 1:1 ratio) taken by a Canon camera (EOS 450D; Canon, Toyko, Japan).
After dissection, micro-computed tomography (mirco-CT) images were obtained from four mandibles (each group represented by two specimens) for morphometric analysis using SkyScan 1.6 system (Bruker-SkyScan, Kontich, Belgium). Scans were acquired at 130 kV and 45 µA and reconstructed using NRecon 1.6.10.4 (Bruker-SkyScan) with a beam hardening correction of 20%. 3D CT-analyzer software (Bruker-SkyScan) was used for the evaluation of bone volume, bone fraction (bone volume/tissue volume), bone surface and trabeculae number, thickness, and separation.
For each specimen, two sites were evaluated: the intervention site, the region bounded posteriorly by the mesial surface of the first PM root and anteriorly by a line located 3 mm mesially; and the non-intervention site, the region bounded anteriorly by the distal surface of the first PM root and posteriorly by the mesial surface of the second PM root. At both sites, the region of interest extended vertically coronal to the plane passing through the apex of the first PM root parallel to the occlusal plane (Figure 2). After micro-CT imaging, samples were further processed in an undecalcified procedure.
Specimens from the second PMs extending anteriorly to 10 mm mesial of the first PM were obtained. The embedded blocks were trimmed and sectioned using an Exakt machine (BS-3000N; Exakt, Norderstedt, Germany) and examined by fluorescence microscopy.
Specimens of the same dimensions as the undecalcified ones were obtained from the remaining 20 rabbits, and fixed in 10% formaldehyde, decalcified in 10% ethylenediaminetetraacetic acid (EDTA-2Na, pH 7.4), embedded in paraffin, and sectioned horizontally to obtain 4-µm-thick slices. The sections from the middle one-third of the PM roots were stained with hematoxylin and eosin (H&E) or processed for osteoclast count.
Tartrate-resistant acidic phosphatase (TRAP) staining was performed to verify the presence of osteoclasts.19 One section from each specimen were treated with acetate buffer and stained by ALP Solution (Sigma-Aldrich Corporation, St. Louis, MO, USA) and light green (L-1886; Sigma-Aldrich) staining. TRAP-positive osteoclasts were counted on light microscope images in the regions of interest, two 1 × 1 mm squares on the mesial side of the first PM and three 1 × 1 mm squares in between the first and second PMs.
Statistical analysis was performed using SPSS 19.0 (IBM Corp., Armonk, NY, USA). Tooth movement data did not follow a normal distribution when assessed by the Shapiro-Wilk test. The Grubbs' test and boxplot method were applied to detect outliers; two values in the control group were identified as outliers and were therefore replaced by the mean of the group before proceeding with further analysis. The Kruskal-Wallis test was used to assess differences in tooth movement between the groups, followed by Mann-Whitney U tests between the pairs of groups for post-hoc analysis using Bonferroni correction. Analysis of variance test with Bonferroni post-hoc for multiple comparisons was applied to assess differences in TRAP-positive osteoclast count. Statistical significance was determined at p < 0.05.
Photos of ten randomly selected mandibles were remeasured 2 weeks later by the same examiner. Intraexaminer reliability was assessed by determining the intraclass correlation coefficient (ICC) value, and an ICC > 0.997 was obtained.
There was a significant intergroup difference in tooth movement (p = 0.024). The IC group (2.52 mm) and TC group (2.48 mm) showed the largest amount of tooth movement, followed by the MP group (2.27 mm) and control group (1.72 mm). The control group showed significantly less tooth movement than the IC and MP groups (p = 0.013 and p = 0.011, respectively). There were no significant differences between the experimental groups. The amount of tooth movement in the IC, TC, and MP groups increased by 46.5%, 44.2%, and 32.0%, respectively, compared to the tooth movement in the control group (Table 1).
Only bone fraction values showed differences between the groups. At the intervention site, the area mesial to the first PM, the control group showed the highest bone fraction (29.4%), followed by the MP group (27.1%), IC group (20.5%), and TC group (19.2%).
In the non-intervention site, the area between the first and second PM, the control group showed the highest bone fraction (53.8%), followed by the MP group (50.5%), TC group (43.3%), and IC group (42.7%) (Figure 3).
At the intervention site, the average TRAP-positive osteoclast count was 20.0, 18.1, and 17.1 in the TC, IC, and MP groups, respectively, but it was 15.1 in the control group. The TC and IC groups showed more osteoclasts and pre-osteoclasts. However, these differences were not statistically significant (p > 0.05) (Figure 4).
At the non-intervention site, the four groups showed relatively similar count numbers, namely, 15.7, 15.0, and 13.2 in the TC, IC, and MP groups, respectively, and 13.5 in the control group (Figure 5).
Previous studies have introduced several corticotomy designs to reduce treatment time.7814 Recently, Kook et al.7 summarized three methods of corticotomy to facilitate mandibular molar protraction in atrophied alveolar ridges. They reported a circumscribed design involving a limited surgical area of target tooth only but with a higher risk of root injury. In contrast, TC is associated with less risk of root injury, but it requires a large flap. They also reported that an indentation design reduced the risk of root injury, but the distal area was not strongly affected by RAP. However, to date, no study has evaluated the effects of these corticotomy designs on treatment outcomes.
In our study, tooth movement was measured on digital photos using software to reduce the error arising from caliper positioning as in a previous method.20 Some studies have reported that corticotomy significantly increased tooth movement.89 In our study, the amount of tooth movement increased by 44.2%, 46.5%, and 32.0%, respectively, in the TC, IC, and MP groups, and these increases in the TC, IC, and MP groups were significantly greater than that in the control group, but were not significantly different from each other. In agreement with these findings, Chen et al.21 showed a significant difference in the amount of tooth movement between the corticotomy group (0.89 mm) and control group (0.80 mm) after 14 days of traction. Meanwhile, Alkebsi et al.22 reported no significant increase in the rate of tooth movement in the micro-osteoperforation group in comparison with a control group. The differences between the results of both studies might be due to differences in the study designs, especially the position of the micro-osteoperforation in relation to the roots.
Cheung et al.23 evaluated the bone fraction in rats using micro-CT by comparing the MP side to the control and found a statistically significant decrease in the MP side. In agreement, our micro-CT analysis demonstrated smaller bone fractions in the TC and IC groups, which showed 65.3% and 69.7% less mineralization, respectively, compared to the control group, while the mineralization in the MP group was only 8.5% less. This might be due to the larger amount of bone removal associated with corticotomy. Nevertheless, our sample analyzed by micro-CT was small and therefore statistical comparisons were not possible.
In our study, TRAP-positive osteoclasts were observed in all groups. A previous study reported a significant increase in osteoclasts in the MP side in comparison with the control side in rats.22 In addition, Chen et al.21 showed rapid increases in the osteoclast numbers and TRAP expression at day 3 after corticotomy in comparison with the control group in rabbits. Then, the number of osteoclasts on the corticotomy side dropped to the same level as on the control side at day 5, while the TRAP expression value dropped on the seventh day, which was followed by a small increase in the second week. This may suggest that most of the biological responses after corticotomy might have occurred in rabbits during the first 2 weeks.
Interestingly, RAP usually enhances the rate of tooth movement to a peak point at 1.3 months in human patients, after which tooth movement gradually reduces to reach normal levels by the third month.2425 Since rabbits' bone metabolism is three times faster than that of human beings,262728 4 weeks would correspond to 3 months in human participants. Therefore, our measurements taken 4 weeks postoperatively might have missed recording the increase in TRAP-positive cells, explaining the lack of significant differences between the experimental and control groups. Further studies including records from several time points, especially in the early stages, might be recommended.
In contrast to the findings of previous studies,1323 the corticotomy groups showed more root resorption than the MP and control groups on H&E slides. However, this visual finding is weak evidence and should be considered with caution. Chan et al.29 reported that micro-osteoperforation resulted in greater total root resorption than control. However, the difference was not clinically significant (0.169 mm3), and there might be some bias due to confounding factors, including the direction and the amount of the forces and the duration of the experiment. A future study evaluating volumetric root resorption might shed more light on that effect. In addition, further randomized clinical trials on micro-osteoperforation might be recommended to evaluate the effects of this treatment modality.
Alikhani et al.11 reported that micro-osteoperforation using a disposable device designed for this purpose by PROPEL 1.5 mm in diameter reduced the duration of orthodontic treatment. In agreement, the micro-osteoperforation performed in rabbits with a mini-screw 1.4 mm in diameter resulted in significantly greater tooth movement than that in control after 4 weeks of traction.
Recently, Owen et al.30 reported that elevation of the flap alone decreased the amount and density of medullary bone and accelerates tooth movement; however, these effects were limited. Meanwhile, our study showed no significant differences between the flapless micro-osteoperforation and corticotomy (after flap elevation) groups, which suggests similar effects for both treatment modalities. Therefore, micro-osteoperforation might be recommended because of its less invasiveness.
Future studies on micro-osteoperforation might be recommended to assess the effect of the number, diameter, and frequency of the micro-osteoperforations, their direction (occlusogingival versus buccolingual), and their proximity to roots.
• There was no statistically significant difference in the amount of tooth movement between IC, TC, and MP; however, the IC and MP groups showed significantly greater tooth movement than the control group.
• The micro-osteoperforation without flap group showed no significant difference in the number of TRAP-positive osteoclasts in comparison with that of the TC and IC groups.
Further well-designed and rigorous randomized clinical trials with longer follow-up periods are recommended to confirm these findings in human subjects.
Figures and Tables
Figure 1
Different study groups. A, Control group (no surgical intervention); B, triangular corticotomy (TC) group; C, indentation corticotomy (IC) group; D, micro-osteoperforation (MP) group.
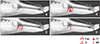
Figure 2
Specimen sites for micro-computed tomography imaging. A, The intervention site, mesial area of the first premolar; B, the non-intervention site, the area between the first and second premolars.
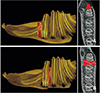
Figure 3
Percentage of bone volume (BV)/tissue volume (TV) in the four groups.
P1, Mesial area of the first premolar; P1–P2, area between the first and second premolars; TC, triangular corticotomy; IC, indentation corticotomy; MP, micro-osteoperforation.
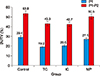
Figure 4
Microphotograph of periodontal tissues with tartrate-resistant acidic phosphatase (TRAP) staining (×200). A, Control group; B, triangular corticotomy (TC) group; C, indentation corticotomy group; D, micro-osteoperforation group. TRAP-positive osteoclasts can be observed on the compression side along the resorbed surface of the alveolar bone. The TC group shows several clusters of TRAP-positive osteoclasts, while the control group forms a straight band.
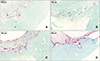
Figure 5
Comparison of tartrate-resistant acidic phosphatase (TRAP)-positive osteoclast counts.
P1, Mesial area of the first premolar; P1–P2, area between the first and second premolars; TC, triangular corticotomy; IC, indentation corticotomy; MP, micro-osteoperforation.
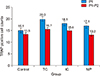
Figure 6
Microphotograph of buccolingual section of the mesial periodontium of the first premolar with H&E staining (×40) of decalcified specimens. A, Control group; B, triangular corticotomy (TC) group; C, indentation corticotomy (IC) group; D, micro-osteoperforation group. Note the enlarged periodontal ligament (PDL) space in B and C, and the increased number of odontoclasts on some teeth surfaces, especially in the TC (B) and IC (C) groups, which might have caused the enlarged PDL space by its increased activity. The figure suggests elevated root resorption, particularly in the TC and IC groups.
ALV, Alveolar bone.
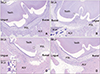
Figure 7
Microphotograph of buccolingual section of the mesial periodontium of the first premolar with H&E staining (×40) of undecalcified specimens. A, Control group; B, triangular corticotomy (TC) group; C, indentation corticotomy (IC) group; D, micro-osteoperforation (MP) group. There was more pronounced root and alveolar bone resorption in the TC (B) and the IC group (C) compared to the MP group (D).
PDL, Periodontal ligament; ALV, alveolar bone.
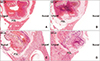
Notes
References
1. Igarashi K, Mitani H, Adachi H, Shinoda H. Anchorage and retentive effects of a bisphosphonate (AHBuBP) on tooth movements in rats. Am J Orthod Dentofacial Orthop. 1994; 106:279–289.


2. Işeri H, Kişnişci R, Bzizi N, Tüz H. Rapid canine retraction and orthodontic treatment with dentoalveolar distraction osteogenesis. Am J Orthod Dentofacial Orthop. 2005; 127:533–541. quiz 625.


3. Liou EJ, Huang CS. Rapid canine retraction through distraction of the periodontal ligament. Am J Orthod Dentofacial Orthop. 1998; 114:372–382.


4. Gantes B, Rathbun E, Anholm M. Effects on the periodontium following corticotomy-facilitated orthodontics. Case reports. J Periodontol. 1990; 61:234–238.


5. Chung KR, Oh MY, Ko SJ. Corticotomy-assisted orthodontics. J Clin Orthod. 2001; 35:331–339.
6. Suya H. Corticotomy in orthodontics. In : Hosl E, Baldauf A, editors. Mechanical and biological basics in orthodontic therapy. Heidelberg: Huthig Buch Verlag;1991. p. 207–226.
7. Kook YA, Lee W, Kim SH, Chung KR. Corticotomy-assisted space closure in adult patients with missing lower molars. J Clin Orthod. 2013; 47:85–95. quiz 139.
8. McBride MD, Campbell PM, Opperman LA, Dechow PC, Buschang PH. How does the amount of surgical insult affect bone around moving teeth? Am J Orthod Dentofacial Orthop. 2014; 145:S92–S99.


9. Mostafa YA, Mohamed Salah, Mehanni S, ElBokle NN, Heider AM. Comparison of corticotomy-facilitated vs standard tooth-movement techniques in dogs with miniscrews as anchor units. Am J Orthod Dentofacial Orthop. 2009; 136:570–577.


10. Teixeira CC, Khoo E, Tran J, Chartres I, Liu Y, Thant LM, et al. Cytokine expression and accelerated tooth movement. J Dent Res. 2010; 89:1135–1141.


11. Alikhani M, Raptis M, Zoldan B, Sangsuwon C, Lee YB, Alyami B, et al. Effect of micro-osteoperforations on the rate of tooth movement. Am J Orthod Dentofacial Orthop. 2013; 144:639–648.


12. Cassetta M, Altieri F, Pandolfi S, Giansanti M. The combined use of computer-guided, minimally invasive, flapless corticotomy and clear aligners as a novel approach to moderate crowding: a case report. Korean J Orthod. 2017; 47:130–141.


13. Charavet C, Lecloux G, Bruwier A, Rompen E, Maes N, Limme M, et al. Localized piezoelectric alveolar decortication for orthodontic treatment in adults: a randomized controlled trial. J Dent Res. 2016; 95:1003–1009.


14. Ruso S, Campbell PM, Rossmann J, Opperman LA, Taylor RW, Buschang PH. Bone response to buccal tooth movements-with and without flapless alveolar decortication. Eur J Orthod. 2014; 36:613–623.


15. Swapp A, Campbell PM, Spears R, Buschang PH. Flapless cortical bone damage has no effect on medullary bone mesial to teeth being moved. Am J Orthod Dentofacial Orthop. 2015; 147:547–558.


16. Lindskog-Stokland B, Hansen K, Ekestubbe A, Wennström JL. Orthodontic tooth movement into edentulous ridge areas--a case series. Eur J Orthod. 2013; 35:277–285.


17. Nagaraj K, Upadhyay M, Yadav S. Titanium screw anchorage for protraction of mandibular second molars into first molar extraction sites. Am J Orthod Dentofacial Orthop. 2008; 134:583–591.


18. Santos PBDD, Herrera Sanches FS, Ferreira MC, de Almeida ALPF, Janson G, Garib D. Movement of mandibular molar into edentulous alveolar ridge: a cone-beam computed tomography study. Am J Orthod Dentofacial Orthop. 2017; 151:907–913.


19. Kim T, Handa A, Iida J, Yoshida S. RANKL expression in rat periodontal ligament subjected to a continuous orthodontic force. Arch Oral Biol. 2007; 52:244–250.


20. Yu JY, Lee W, Park JH, Bayome M, Kim Y, Kook YA. Histologic effects of intentional-socket-assisted orthodontic movement in rabbits. Korean J Orthod. 2012; 42:207–217.


21. Chen YW, Wang HC, Gao LH, Liu C, Jiang YX, Qu H, et al. Osteoclastogenesis in local alveolar bone in early decortication-facilitated orthodontic tooth movement. PLoS One. 2016; 11:e0153937.


22. Alkebsi A, Al-Maaitah E, Al-Shorman H, Abu Alhaija E. Three-dimensional assessment of the effect of micro-osteoperforations on the rate of tooth movement during canine retraction in adults with Class II malocclusion: a randomized controlled clinical trial. Am J Orthod Dentofacial Orthop. 2018; 153:771–785.


23. Cheung T, Park J, Lee D, Kim C, Olson J, Javadi S, et al. Ability of mini-implant-facilitated micro-osteoperforations to accelerate tooth movement in rats. Am J Orthod Dentofacial Orthop. 2016; 150:958–967.


24. Buschang PH. Surgically facilitated orthodontics: what does the evidence say?. In : Kapila SD, Goonewardene M, Kim-Berman H, Koster KY, editors. Interdisciplinary therapy: using contemporary approaches for complex cases. Ann Arbor: The University of Michigan;2016. p. 135–165.
25. Aboul-Ela SM, El-Beialy AR, El-Sayed KM, Selim EM, El-Mangoury NH, Mostafa YA. Miniscrew implant-supported maxillary canine retraction with and without corticotomy-facilitated orthodontics. Am J Orthod Dentofacial Orthop. 2011; 139:252–259.


27. Mapara M, Thomas BS, Bhat KM. Rabbit as an animal model for experimental research. Dent Res J (Isfahan). 2012; 9:111–118.


28. Castañeda S, Largo R, Calvo E, Rodríguez-Salvanés F, Marcos ME, Díaz-Curiel M, et al. Bone mineral measurements of subchondral and trabecular bone in healthy and osteoporotic rabbits. Skeletal Radiol. 2006; 35:34–41.

