Abstract
Eucommiae Cortex (EC), bark of Eucommia ulmoides, has been known as a traditional medicine to regulate hypertension and immune system. Because silk of gum in the EC blocks the release of active ingredients, EC generally has been utilized after processing with carbonization or salt-water to breakdown it. This study aimed to investigate the differences of non-processed EC and processed EC on antioxidant and anticancer properties. Antioxidant capacity was assessed by measuring the content of total polyphenols, reducing power, and ABTS radical scavenging effect. And anticancer effects were examined by evaluating the viability of pancreatic cancer cells and wound healing ability. The results demonstrated that processed EC contained more content of polyphenols and exhibited more potent reducing power and radical scavenging effect than non-processed EC. In addition, processed EC more efficiently inhibited proliferation and migration of pancreatic cancer cells. These results suggest that processing of medicinal plants can improve the biological properties such as antioxidant or anticancer activity, which may lead to the development of herbal medicine treatment technology.
Eucommiae Cortex (EC) (bark of Eucommia ulmoides, Eucommiaceae) has been used as a tonic herb for treatment of hypertension in Korea, Japan and China.1 EC are commonly known as Du-zhong, which has long been known as traditional medicine for hypercholesterolemia, hypertension, and fatty liver.2 Recently, it has been reported many pharmacological effects of EC including anti-hypertention,3 anti-tumor,4 anti-inflammatory,5 anti-obesity,6 and anti-osteoporosis.7 In addition, EC has been found to be rich of bioactive compounds such as chlorogenic acid, quercetin, rutin, geniposidic acid, and pinoresinol diglucoside.8
The processed EC is widely recommended due to break the silk of gum which prevents the extraction of active compounds from the bark of EC.910 For process of EC, carbonization and stir-frying with salt water are generally used methods. It has been reported that the salt-processed EC (stir-fried with salt water) showed the increased extraction of chlorogenic acid9 while carbonized EC had loss of pinoresinol diglucoside.910 Although the changes in amounts of components from processed EC have been reported, the alterations in activities of processed EC have rarely been described.
To investigate the differences on biological activities of non-processed EC and processed EC, we assessed the polyphenol content, reducing power, and radical scavenging potential of non-processed EC (N-EC), carbonized EC (C-EC), and salt-processed EC (S-EC) for antioxidant effect. Also, anti-cancer properties of non-processed and processed EC were examined by evaluation of cancer cell proliferation, cell cycle analysis and cancer cell migration. Our findings may be useful for development of processing technology for herbal medicine by recognizing the changed pharmacological properties of processed EC.
Non-processed Eucommiae Cortex (N-EC), carbonized EC (C-EC), and salt processed EC (S-EC) were purchased from herbal drug store (Herbal pharmacist Jae Ho Lee, Wonju, Korea) in September 2016. C-EC and S-EC were prepared as follows: Eucommiae Cortex (EC) was baked with stirring at low temperature until it turns black (C-EC); 2 g of salt per 100 g of EC was dissolved in water and sprayed on EC, allowed to stand until absorbed and then baked with stirring to dry (S-EC). The voucher specimens (No. EU201609C001, EU201609CP001, and EU201609CP002 for N-EC, C-EC, and S-EC, respectively) were deposited in the herbarium of School of Industrial Bio-Pharmaceutical Science at Semyung University. Dried materials (450 g of each samples) of N-EC, C-EC, and S-EC were extracted three times with methanol (4.5 L) at 40 ℃ for 3 h in an ultrasonic bath. The combined extracts were concentrated using a rotary evaporator under vacuum to generate the methanol extract of N-EC (38 g), C-EC (66 g), and S-EC (37 g). The resulting dried extracts were dissolved in methanol (for measurement of antioxidant activity) or in DMSO (for cell treatment) and stored at 4 ℃ until use.
Folin-Ciocalteu's phenol reagent, gallic acid, L-ascorbic acid, 2,2′-azino-bis(3-ethylbenzothiazoline-6-sulfonic acid) diammonium salt (ABTS), potassium persulfate, potassium ferricyanide, ferric chloride, sodium carbonate, dimethylsulfoxide (DMSO), pinoresinol diglucoside were purchased from Sigma-Aldrich (St. Louis, MO, USA). Water and acetonitrile of HPLC grade were purchased from TEDIA High Purity Solvents (Farifield, OH, USA). Methanol (reagent grade) was from Duksan Pure Chemicals Co. Ltd. (Ansan, Korea).
High Performance liquid chromatography (HPLC) was performed by Agilent HPLC 1260 system (Agilent, Santa Clara, CA, USA). Zorbax Eclipse Plus C18 (5 µm, 4.6 × 250 mm, Agilent) column was used and mobile phase is consisted of A (0.1% aqueous acetic acid) and B (acetonitrile with 0.1% acetic acid) with a gradient elution as follows: 0 – 10 min, 3% – 10% B; 10 – 20 min, 10% – 15% B; 20 – 40 min, 15% – 20% B; 40 – 50 min, 20% – 25% B; 50 – 60 min, 25% – 90% B; 60 – 70 min, 90% B; 70 – 80 min, 90% – 3% B; 80 – 90 min, 3% B. The analysis was carried out at flow rate of 1.0 mL/min with UV detection absorbance at 230 nm. The standard solution of pinoresinol diglucoside was prepared and diluted with methanol for the calibration curve. Calibration curve was generated by plotting the peak areas versus the corresponding concentrations of pinoresinol diglucoside. The regression equations were calculated in the form of y = ax + b, where y and x correspond to peak area and compound concentration, respectively. The limit of detection (LOD) and limit of quantitation (LOQ) of pinoresinol diglucoside were measured at signal-to-noise ratios of 3 and 10, respectively.
Total polyphenol content was measured by Folin-Ciocalteu assay.11 Briefly, 100 µL of each extract solution was mixed with 50 µL of Folin-Ciocalteu's phenol reagent and 300 µL of 20% sodium carbonate. The mixture was incubated at 25 ℃. After 1 h, the absorbance was measured at 725 nm. Gallic acid solutions were used to perform the calibration curve and total polyphenol content was expressed as mg gallic acid equivalent (GAE) per g of extract.
To assess the ABTS radical scavenging activity, ABTS radical cation decolorization method was carried out with minor modifications.12 Briefly, 7.4 mM of ABTS and 2.6 mM potassium persulfate were mixed and incubated at room temperature for 24 h in dark to produce the ABTS radical cation (ABTS·+). The ATBS·+ solution was diluted with PBS to obtain the absorbance of 0.70 ± 0.02 at 732 nm. An aliquot (50 µL) of each extract solution was mixed with 950 µL of the diluted ATBS·+ solution and incubated for 10 min in the dark. The absorbance was measured at 732 nm and ABTS radical scavenging activity (%) was calculated as; %=[1−(O.D. of sample/O.D. of control)] × 100.
The reducing power of each extract was accessed using the method described by Oyaizu (1986).13 The mixture of each extract solution (200 µL), 0.2M PBS (200 µL) and 1% potassium ferricyanide solution (200 µL) was reacted at 50 ℃ for 20 min. After adding 200 µL of 10% trichloroacetic acid solution to the mixture, centrifugation was performed at 4000 rpm and then upper layer of the solution was mixed with 150 µL of 0.1% ferric chloride solution. The absorbance was measured at 700 nm
Human pancreatic cancer cell line, PANC-1 cells (American Type Culture Collection, Manassas, VA, USA) were cultured in DMEM supplemented with 10% FBS, 100 U/mL penicillin, and 100 µg/mL streptomycin (Life technologies, Frederick, MD, USA) at 37 ℃ in 5% CO2.
To assess the effect of test samples against viability of PANC-1 pancreatic cancer cells, 3-(4,5-dimethylthizol-2-yl)-2,5-diphenyl-tetrasolium bromide (MTT, Sigma-Aldrich, St. Louis, MO, USA) assay was performed. Briefly, PANC-1 cells were plated at a density of 2 × 103 cells/well in a 96-well plate. Cells were treated with various concentrations of test samples for 48 h. And then, MTT solution (5 mg/mL) was treated for 4 h at 37 ℃ in 5% CO2 and formed formazan crystals were lysed with DMSO. The absorbance at 540 nm was measured by using a microplate reader (Molecular Devices, CA, USA). The percentage of viable cells was determined in relation to the vehicle-treated group.
PANC-1 cells were plated at a density of 2.5 × 105 cells/60 mm dish and incubated overnight. Cells were treated with test samples for 24 h. And then cells were harvested with trypsin-EDTA and fixed with 70% ethanol at 4 ℃ for overnight. Fixed cells were stained with propidium iodide (PI, BD Bioscience, San Jose, CA, USA) in the presence of RNase A for 45 min in the dark. Cell cycle analysis was performed by flow cytometry (BD Bioscience, San Jose, CA, USA).
Effect of samples on cancer cell migration was assessed by a wound-healing assay. PANC-1 pancreatic cancer cells (1 × 104 cells/well) were plated on 24 well plate for 12 h and grown until 90% confluence. The monolayers were scratched off using a sterile 100 µL pipet tip, and then cells were incubated at 37 ℃ with test samples (50 µg/mL). After 20 h and 40 h, cells migration into the wounded areas were observed. Images were captured using an inverted microscope at 0 h, 20 h and 40 h after scratching. The areas of the scratches were analyzed using ImageJ (US National Institutes of Health, Bethesda, MD, USA).
In Korean Pharmacopoeia 11th edition, quality regulation of Eucommiae Cortex has been stipulated to contain pinoresinol diglucoside not less than 0.05%. In order to investigate whether the content of marker component was changed, quantification of pinoresinol diglucoside in N-EC, C-EC, and S-EC was performed by HPLC system (Fig. 1). The chromatographic peak in the sample solution was identified by comparing its retention time with that of the standard. The content (wt %) of (1) for N-EC, C-EC, and S-EC was 1.79%, 1.64%, and 0.77%, respectively. The coefficient of correlation (r2) was 0.9997 (linear range: 5–500 µg/mL) and the regression equation was y = 15.254x − 38.493. The LOD and LOQ were 0.83 µg/mL and 2.76 µg/mL, respectively. Based on chromatographic result, the content of pinoresinol diglucoside in C-EC and S-EC was decreased. It had been reported that the decreased content of pinoresinol diglucoside was measured in processed EC although the content of chlorogenic acid was increased in salt-processed EC.91014 Tao et al. (2014) described that charcoal processed EC and salted processed EC exhibited the reduced quantities of chlorogenic acid, geniposide and geniposidic acid as well as pinoresinol diglucoside.15 These results mean that content of the ingredients may be different by processing methods.
To examine whether antioxidant activity of the processed EC has been changed compared with that of non-processed one, total polyphenol contents of N-EC, C-EC and S-EC were measured by Folin-Ciocalteu assay. As seen in Table 1, C-EC and S-EC showed higher total polyphenol content (12.86 and 22.46mg GAE/g of extract, respectively) than N-EC (7.60 mg GAE/g of extract). From this result, we can postulate that more hydrophilic constituents were extracted from the carbonized or salt-treated EC which was processed to break the silk of gum in the bark of EC. And then we investigated the ABTS radical scavenging activity and reducing power of N-EC, C-EC, and S-EC. As shown in Fig. 2A, C-EC and S-EC showed potent ABTS radical scavenging activity (27.8% and 83.8% at 200 µg/mL, respectively) while N-EC showed 15.1% of scavenging activity at 200 µg/mL. In addition, N-EC, C-EC, and S-EC displayed 0.441, 0.618 and 1.187 of O.D. values at 200 µg/mL, respectively, which means S-EC had the highest reducing power (Fig. 2B). These data are in agreement with report that the powerful antioxidant effect is positively correlated with the polyphenol content.216 From these result, C-EC and S-EC have the higher level of polyphenol content, reducing power, and ATBS radical scavenging activity than those of N-EC. Taken together, C-EC and S-EC presented higher level of polyphenol content and more powerful antioxidant effect, which means that more constituents were extracted from the processed EC and antioxidant activity of processed EC was altered.
Oxidative stress-mediated cellular injuries by free radicals such as superoxide radical anion, hydroxyl radicals, singlet oxygen, and hydrogen peroxide were involved in aging, neurodegenerative diseases17 and cancer.18 Antioxidant diets or phytochemicals have been important role in chemoprevention and chemotherapeutics.19 Thus, we examined the anti-cancer properties of processed EC which showed high potential of antioxidant effect. First, we measured the cell viability of cancer cells in presence of N-EC, C-EC, and S-EC by MTT assay. The viabilities of PANC-1 pancreatic cancer cells were concentration-dependently affected by N-EC, C-EC, and S-EC. While N-EC showed the cancer cell viability with 65% at 200 µg/mL, C-EC and S-EC displayed 40% and 23% of cancer cell viabilities, respectively (Fig. 3). This observation showed that S-EC showed the lowest cancer cell viability and the most potent suppression of cancer cell proliferation, which indicated that processed EC exhibited more powerful inhibition against cancer cell proliferation than non-processed one. Zhu et al. (1997) reported that processed EC more potently inhibited the cortisol-induced T cell reduction or more strongly enhanced phagocytic activity of macrophages than non-processed EC.20 These results suggested that processed EC had the improved biological activity by repressing cancer cell proliferation or boosting the immune system.
Next, we evaluated the effect of processed EC on cell cycle distribution in PANC-1 cells by flow cytometry to investigate if processed EC-mediated suppression of cancer cell proliferation was due to its cell cycle intervention. Fig. 4 showed that N-EC, C-EC, and S-EC caused cells to accumulate in the G2/M phase with 22, 25 and 40%, respectively, compared with control cells (18%). Furthermore, S-EC induced the most significant accumulation of cells in G2/M phase and a decrease of cell papulation in the G1 phase. These results disclosed that the processed EC, particularly, S-EC potently caused the cell cycle arrest in G2/M phase in PANC-1 cancer cells, resulting to inhibit the cancer cell proliferation.
Since cancer cell migration is crucial to metastasis,21 the inhibitory effect of processed EC on cancer cell migration was evaluated by a wound healing assay (Fig. 5). We measured the wound size of 0 h and this was calculated as 100%. After 20 h and 40 h, the wound sizes of vehicle were reduced as 86.8% and 37.1%, respectively. N-EC displayed 82.7% (20 h) and 33.8% (40 h) of wound size at 50 µg/mL. However, C-EC and S-EC were 88.2% and 97.4% of wound size at 20 h, and 49.3% and 53.4% of wound size at 40 h, respectively. The decreasing ratio of wound size was interrupted by C-EC and S-EC, which means that C-EC and S-EC were active in inhibiting the cell migration. This result showed that the processed EC demonstrated more potent inhibitory effect on cancer cell migration than non-processed EC.
From Eucommia ulmoides, over 150 compounds have been isolated, including lignans, iridoids, phenolics, steroids, terpenoids and flavonoids. Some of these compounds exhibit various bioactivities such as antioxidant, antihypertensive, anti-obesity, anti-inflammatory, anti-cancer, and neuroprotective effects.822 Astragalin (kaempferol-3-O-β-D-glucoside), a natural flavonoid from various medicinal plants including Eucommiae Cortex, Mori Radicis Cortex, and Astragali Radix, has been reported to alleviate the production of ROS and the endotoxin-induced oxidative stress.2223 It also modulates inflammatory responses by regulating the NF-κB and MAPK signaling pathway.2425 In addition, astragalin is known as an anti-oncogenic compound against cancer proliferation by inhibiting the ERK1/2 and Akt signaling.26 Rutin, another flavonoid from Eucommiae Cortex, Citri Unshius Pericarpium, and Sophorae Flos, has been known as vitamin P which has been explored for a number of pharmacological effects.22272829 Rutin has been observed for its promotion of the macrophage phagocytic ability.30 Also, rutin has been known to detain cancer cell proliferation by cell cycle arrest and/or apoptosis, along with inhibition of angiogenesis, and/or metastasis in cancer system.3132 In this study, we have demonstrated the enhanced potency of processed EC on antioxidant and anticancer properties. And identification of the chemical constituents for the improved biological activities should be uncovered in further study.
In conclusion, present study disclosed that processed EC, carbonized EC or salt-processed EC, displayed the upgraded capabilities of suppressing the cancer cell proliferation with disruption of cell cycle and inhibiting the cancer cell migration as well as high level of polyphenol content and antioxidant activity. Therefore, these findings indicate that the processing of medicinal plants is not only an ordinary pre-treatment for medicine preparation but also a pharmaceutical technique for improved potency of herbal medicine. Also, those suggest that the processed Eucommiae Cortex with enhanced potential can be a therapeutic source for treatment of cancers and oxidative stress mediated diseases.
Figures and Tables
Fig. 1
HPLC chromatograms of pinoresinol diglucose (A), non-processed Eucommiae Cortex (B), carbonized Eucommiae Cortex (C), and salt-processed Eucommiae Cortex (D).
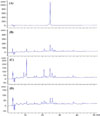
Fig. 2
The antioxidant effects of processed Eucommiae Cortex (EC). (A) The effect of non-processed EC (N-EC), carbonized EC (C-EC), and salt-processed EC (S-EC) on ABTS radical scavenging. (B) The reducing power of N-EC, C-EC, and S-EC. L-ascorbic acid (L-A, 100 µg/mL) was used as a positive control. Values were expressed as means ± S.D. *p < 0.05 indicates significant difference from the vehicle treatment.
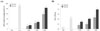
Fig. 3
The inhibitory effect of processed Eucommiae Cortex (EC) on pancreatic cancer cell proliferation. The effect of non-processed EC (N-EC), carbonized EC (C-EC), and salt-processed EC (S-EC) on pancreatic cancer cell viability by MTT assay. Cells were treated with various concentrations of test samples for 48 h. And then, MTT solution was treated and formed formazan crystals were lysed with DMSO. The absorbance at 540 nm was measured. Values were expressed as means ± S.D. *p < 0.05 indicates significant difference from the vehicle treatment.
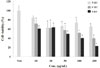
Fig. 4
The effect of processed Eucommiae Cortex (EC) on cell cycle distribution of PANC-1 pancreatic cancer cells. Cells were treated with non-processed EC (N-EC), carbonized EC (C-EC), and salt-processed EC (S-EC) for 24 h. Fixed cells were stained with propidium iodide in the presence of RNase A for 45 min in the dark. Cell cycle analysis was performed by flow cytometry.
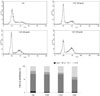
Fig. 5
The effect of processed Eucommiae Cortex (EC) on migration of PANC-1 pancreatic cancer cells. Migration of cancer cells by non-processed EC (N-EC), carbonized EC (C-EC), and salt-processed EC (S-EC) were evaluated by wound healing assay. Images are representative wound areas for three independent experiments that show similar results. Results were expressed as means ± S.D. *,# p < 0.05 indicates significant difference from the vehicle treatment.
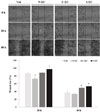
References
1. Dai X, Huang Q, Zhou B, Gong Z, Liu Z, Shi S. Food Chem. 2013; 139:563–570.
2. Hsieh CL, Yen GC. Life Sci. 2000; 66:1387–1400.
3. Lang C, Liu Z, Taylor HW, Baker DG. Am J Chin Med. 2005; 33:215–230.
4. Choi YH, Seo JH, Kim JS, Heor JH, Kim SK, Choi SU, Kim YS, Kim YK, Ryu SY. Korean J Pharmacogn. 2003; 34:308–313.
5. Kim MC, Kim DS, Kim SJ, Park J, Kim HL, Kim SY, Ahn KS, Jang HJ, Lee SG, Lee KM, Hong SH, Um JY. Am J Chin Med. 2012; 40:135–149.
6. Hirata T, Kobayashi T, Wada A, Ueda T, Fujikawa T, Miyashita H, Ikeda T, Tsukamoto S, Nohara T. Bioorg Med Chem Lett. 2011; 21:1786–1791.
7. Zhang R, Liu ZG, Li C, Hu SJ, Liu L, Wang JP, Mei QB. Bone. 2009; 45:553–559.
8. He X, Wang J, Li M, Hao D, Yang Y, Zhang C, He R, Tao R. J Ethnopharmacol. 2014; 151:78–92.
9. Chai X, Wang Y, Su Y, Bah AJ, Hu L, Gao Y, Gao X. J Pharm Biomed Anal. 2012; 57:52–61.
10. Seo CS, Kim JH, Shin HK, Kim BS. Korean J Pharmacogn. 2015; 46:123–132.
11. Singleton VL, Rossi JA. Am J Enol Vitic. 1965; 16:144–158.
12. Re R, Pellegrini N, Proteggente A, Pannala A, Yang M, Rice-Evans C. Free Radic Biol Med. 1999; 26:1231–1237.
13. Oyaizu M. Jpn J Nutr. 1986; 44:307–315.
14. Chen XY, Luo LI, Ren GC, Qu GY, Dong LS. West China J Pharm Sci . 2008; 5:592–593.
15. Tao Y, Sheng C, Li WD, Cai BC, Lu TL. Zhongguo Zhong Yao Za Zhi. 2014; 39:4352–4355.
16. Yen GC, Hsieh CL. J Agric Food Chem. 1998; 46:3952–3957.
17. Hu W, Wang G, Li P, Wang Y, Si CL, He J, Long W, Bai Y, Feng Z, Wang X. Chem Biol Interact. 2014; 224:108–116.
18. Milkovic L, Siems W, Siems R, Zarkovic N. Curr Pharm Des. 2014; 20:6529–6542.
19. Talero E, Avila-Roman J, Motilva V. Curr Pharm Des. 2012; 18:3939–3965.
20. Zhu Y, Hao W, Li X. Zhongguo Zhong Yao Za Zhi. 1997; 22:598–601.
21. Wells A, Grahovac J, Wheeler S, Ma B, Lauffenburger D. Trends Pharmacol Sci. 2013; 34:283–289.
22. Li Y, Han C, Wang J, Xiao W, Wang Z, Zhang J, Yang Y, Zhang S, Ai C. J Ethnopharmacol. 2014; 151:452–460.
23. Riaz A, Rasul A, Hussain G, Zahoor MK, Jabeen F, Subhani Z, Younis T, Ali M, Sarfraz I, Selamoglu Z. Adv Pharmacol Sci. 2018; 2018:9794625.
24. Soromou LW, Chen N, Jiang L, Huo M, Wei M, Chu X, Millimouno FM, Feng H, Sidime Y, Deng X. Biochem Biophys Res Commun. 2012; 419:256–261.
25. Cho IH, Gong JH, Kang MK, Lee EJ, Park JH, Park SJ, Kang YH. BMC Pulm Med. 2014; 14:122.
26. Chen M, Cai F, Zha D, Wang X, Zhang W, He Y, Huang Q, Zhuang H, Hua ZC. Oncotarget. 2017; 8:26941–26958.
27. Choi SY, Ko HC, Ko SY, Hwang JH, Park JG, Kang SH, Han SH, Yun SH, Kim S. J Biol Pharm Bull. 2007; 30:772–778.
28. Chen QC, Zhang WY, Jin W, Lee IS, Min BS, Jung HJ, Na M, Lee S, Bae K. Planta Med. 2010; 76:79–81.
29. Ganeshpurkar A, Saluja AK. Saudi Pharm J. 2017; 25:149–164.
30. Lin JP, Yang JS, Lu CC, Chiang JH, Wu CL, Lin JJ, Lin HL, Yang MD, Liu KC, Chiu TH, Chung JG. Leuk Res. 2009; 33:823–828.
31. Chen H, Miao Q, Geng M, Liu J, Hu Y, Tian L, Pan J, Yang Y. ScientificWorldJournal. 2013; 2013:269165.
32. Araújo JR, Gonçalves P, Martel F. Nutr Res. 2011; 31:77–87.