Abstract
Alzheimer's disease is a chronic neurodegenerative disorder with no curative treatment. The commercially available drugs, which target acetylcholinesterase, are not satisfactory. The aim of this study was to investigate the cholinesterase inhibitory activity of Solenostemma argel aerial part. Eight compounds were isolated and identified by NMR: kaempferol-3-O-glucopyranoside (1), kaempferol (2), kaempferol-3-glucopyranosyl(1→6)rhamnopyranose (3) p-hydroxybenzoic acid (4), dehydrovomifoliol (5), 14,15-dihydroxypregn-4-ene-3,20-dione (6), 14,15-dihydroxy-pregn-4-ene-3,20-dione-15β-D-glucopyranoside (7) and solargin I (8). Two of them (compounds 2 and 3) could inhibit over 50 % of butyrylcholinesterase activity at 100 µM. Compound (2) displayed the highest inhibitory effect against acetylcholinesterase (AChE) and butyrylcholinesterase (BChE) with a slight selectivity towards the latter. Molecular docking studies supported the in vitro results and revealed that (2) had made several hydrogen and π-π stacking interactions which could explain the compound potency to inhibit AChE and BChE.
Alzheimer's disease (AD) is a chronic neurodegenerative disorder that is characterized by a deficient cholinergic neurotransmission.1 The current treatments use acetylcholinesterase (AChE) inhibitors but these drugs have side effects and bioavailability issues.23 Therefore, better cholinesterase inhibitors are needed. Work on medicinal plants has led to the isolation of several cholinesterase (ChE) inhibitors such as galantamine and physostigmine.4 Other ChE inhibitors were found in the Apocynaceae family such as pregnane glycosides.5 Those unusual compounds were also reported in the genus Solenostemma.6 Solenostemma argel (Delile) Hayne (Apocynaceae) is a desert plant found in the south of Algeria.7 It has been used traditionally to treat various diseases including bronchitis, neuralgia and sciatica.8 In addition, previous studies reported interesting antimicrobial, antiproliferative and anti-inflammatory activities of this species.6910 No cholinesterase inhibitory activity of this plant has been reported. The aim of this study was to investigate the cholinesterase inhibitory activity of the aerial parts of Solenostemma argel by bioguided fractionation to purify and identify active compounds and to test them in vitro and in silico on AChE and BChE.
NMR spectra (1H, 500 MHz; 13C, 500 MHz) were recorded on a Bruker Advance 500 spectrometer. Mass spectra (HR-ESI-MS) were performed on a PE Sciex API 3000 triple quadrupole mass spectrometer equipped with an ion spray turbo source (Thermo Fisher Scientific Extractive). The HPLC apparatus comprised a Shimadzu SCL-10 Avp pump and an SPD-M20A photodiode detector with an analytical and a preparative RP18 column (Silica Upti-prep Strategy, 250 mm × 22 mm; particle size 5 µm, Grace, France). TLC was performed on silica gel 60 F254 plates (Merck, Darmstadt, Germany) and the absorbance of the reaction was measured using Nest 196 Microplate Spectrostar Nano (BMG Labtech).
Aerial parts of Solenostemma argel were harvested in March 2013 in the area of Djanet (Tassili of N'Ajjers massif; South-East of Algeria). The botanical identification of the plant was done by Dr. Bazri K, Department of Plant Biology, Faculty of Biology and Natural Sciences, University of Frères Mentouri, Constantine, Algeria. A voucher specimen (Sol.2013.31) has been deposited at the Herbarium of Laboratory of Applied Biochemistry, University of Frères Mentouri, Constantine, Algeria.
The fresh plant was dried in the shade and then crushed using an electric blender to obtain a fine powder. 500 g of the powder were macerated in MeOH/H2O (80:20). This extract was concentrated to dryness and the crude extract (71.4 g) was suspended in H2O and partitioned successively with petroleum ether, CHCl3, EtOAc and n-BuOH (3 × 500 mL each), then exhaustively concentrated to yield petroleum ether (1.2 g), CHCl3 (21.4 g), EtOAc (2.1 g) and n-BuOH (31.4 g) extracts.
After a preliminary cholinesterase inhibitory screening of various extracts by bioautography, the chloroform extract was selected for further studies and purification of pure compounds.
The chloroform extract was fractionated through an open column chromatography on silica gel using two gradients: Toluene/EtOAc (0 to 100% EtOAc) and then EtOAc/MeOH (0 to 100% MeOH). This led to 23 fractions that were all tested with the TLC acetylcholinesterase assay. Among the most active fractions (10 - 19), fraction 17 was submitted to another open column chromatography with a gradient of Heptane/EtOAc of increasing polarity and then EtOAc/MeOH. Two of its active subfractions led to the isolation of two pure compounds (1 and 4) by preparative HPLC. Fractions 10, 13 and 19 were further fractionated by preparative HPLC (C18 column) and eluted with mixtures of water containing 0.1% formic acid (solvent A) and acetonitrile (solvent B) at a flow rate of 12 mL/min. This led to the isolation of compounds 2 and 5 from fraction 10, compound 6 from fraction 13 and compounds 3, 7 and 8 from fraction 19.
Yellow powder; 1H-NMR (CD3OD) δ: 8.08 (1 H, d, J = 8.70 Hz, H-2', H-6'), 6.91 (1 H, d, J = 8.70 Hz, H-3', H-5'), 6.43 (1 H, s, H-8), 6.22 (1 H, s, H-6), 5.72 (1 H, d, J = 7.10 Hz, H-1''), 3.91 (1 H, m, H-6''a), 3.73 (1 H, m, H-6''b), 3.56 (1 H, m, H-5''), 3.54 (1 H, m, H-2''), 3.52 (1 H, m, H-3''), 3.44 (1 H, m, H-4''). 13C-NMR (CD3OD) δ: 165.0 (C-7), 161.74 (C-5), 157.2 (C-2), 156.9 (C-9), 131.5 (C-3), 122.6 (C-1'), 115.2 (C-3', C-5'). HR-ESI-MS (negative ion) m/z 447.09430 [M-H]−(Calcd for C21H19O11).
Yellow powder; 1H-NMR (CD3OD) δ: 8.11 (1 H, d, J = 8.92 Hz, H-2', H-6'), 6.92 (1 H, d, J = 8.77 Hz, H-3', H-5'), 6.41 (1 H, s, H-8), 6.20 (1 H, s, H-6). 13C-NMR (CD3OD) δ: 176.1 (C-4), 164.4 (C-7), 161.14 (C-5), 159.17 (C-9), 156.86 (C-2), 136.2 (C-3), 129.2 (C-2', C-6'), 115.1 (C-3', C-5'), 103.4 (C-10), 98.1 (C-6), 93.1 (C-8). HR-ESI-MS (negative ion) m/z 285.0406 [M-H]−(Calcd for C15H9O6).
Yellow powder; 1H-NMR (CD3OD) δ: 8.07 (1 H, d, J = 9.00 Hz, H-2', H-6'), 6.91 (1 H, d, J = 9.00 Hz, H-3', H-5'), 6.40 (1 H, s, H-8), 6.20 (1 H, s, H-6), 5.78 (1 H, d, J = 7.10 Hz, H-1''), 5.25 (1 H, d, J = 1.3,H-1'''), 4.01 (1 H, m, H-2'''), 3.77 (1 H,m, H-6''a), 3.65 (1 H, m, H-2''),3.58 (1 H, m, H-3'''), 3.52 (1 H, m, H-6''b), 3.44 (1 H, m, H-5'''), 3.43 (1 H, m, H-3''), 3.35 (1 H, m, H-4'', H-4'''), 3.29 (1 H, m, H-5''), 3.44 (1 H, m, H-4''), 0.99 (3 H, d, J = 6.20, H-6'''). 13C-NMR (CD3OD) δ: 179.3 (C-4), 165 (C-7), 164.8 (C-5), 159.9 (C-4'), 157.2 (C-2, C-9), 133.3 (C-3), 130.3 (C-2', C-6'), 121.8 (C-1'), 104.8 (C-10), 101.23 (C-1'''), 99.4 (C-1''), 99.3 (C-6), 93.2 (C-8), 78.69 (C-2''), 77.5 (C-3'''), 76.1 (C-4''), 72.6 (C-4'''), 71.0 (C-3''), 70.6 (C-2'''), 70.38 (C-5''), 68.9 (C-5'''), 61.23 (C-6''), 16.2 (C-6'''). HR-ESI-MS (negative ion) m/z 593.1512 [M-H]−(Calcd for C27H29O15).
White powder; 1H-NMR (CD3OD) δ: 7.88 (2 H, d, J = 8.47 Hz, H-2, H-6), 6.79 (2 H, d, J = 8.47 Hz, H-3, H-5). 13C-NMR (CD3OD) δ:171.6 (COOH), 161.16 (C-4), 131.4 (C-2, C-6), 123.8 (C-1), 114.41 (C-3, C-5). HR-ESI-MS (negative ion) m/z 137.1221 [M-H]− (Calcd for C7H5O3).
Yellow powder; 1H-NMR (CD3OD) δ: 7.02 (1 H, d, J = 15.92 Hz, H-7), 6.46 (1 H, d, J = 15.92 Hz, H-8), 5.96 (1 H, m, H-4), 2.63 (1 H, d, J = 17.83 Hz, H-2), 2.33 (3 H, s, H-10), 1.92 (3 H, d, J = 1.41 Hz, H-13), 1.08 (3 H, s, H-12), 1.03 (3H, s, H-11). 13C-NMR (CD3OD) δ: 199.30 (C-9), 199.0 (C-3), 163.3 (C-5), 146.97 (C-7), 130.4 (C-8), 126.7 (C-4), 78.53 (C-6), 49.14 (C-2), 41.68 (C-1), 26.21 (C-10), 23.34 (C-12), 22.13 (C-11), 17.74 (C-13). HR-ESI-MS (negative ion) m/z 221 [M-H]− (Calcd for C13H17O3).
White powder; 1H-NMR (CD3OD) δ: 5.74 (1 H, s, H-4), 4.45 (1 H, dd, J = 7.55, 9.07 Hz, H-15), 2.81 (1 H, dd, J = 4.99, 10.08 Hz, H-17), 2.50 (1 H, m, H-2), 2.42 (1 H, m, H-16a), 2.34 (1 H, m, H-6a), 2.29 (1 H, m, H-6b), 2.27 (3 H,s, H-21), 2.20 (1 H, m, H-7a), 2.13 (1 H, m, 1 a), 1.80 (1 H, m, H-8), 1.74 (1 H, m, 1b), 1.70 (1 H, m, 16b), 1.60 (1 H, m, 12 a), 1.58 (1 H, m ,11 a), 1.50 (1 H, m, 12b), 1.49 (1 H, m, 7b), 1.48 (1 H, m, 11b), 1.39 (1 H, m, H-9), 1.25 (3 H, s, 19), 1.05 (3 H, s, 18). 13C-NMR (CD3OD) δ: 217.6 (C-20), 201.3 (C-3), 173.9 (C-5), 123.1 (C-4), 82.0 (C-14), 73.8 (C-15), 60 (C-17), 49.4 (C-9), 40.8 (C-8), 39.0 (C-10), 38.3 (C-12), 36.0 (C-1), 35.1 (C-16), 33.9 (C-6), 33.7 (C-2), 31.6 (C-21), 27.4 (C-7), 21.0 (C-11), 17.3 (C-19), 15.6 (C-18). HR-ESI-MS (negative ion) m/z 345.20594 [M-H]− (Calcd for C21H30O4).
White powder ; 1H-NMR (CD3OD) δ: 5.74 (1 H, s, H-4), 4.30 (1 H, d, J = 7.7 Hz, H-1'), 3.91 (1 H, dd, J = 2.20, 11.60 Hz, H-6'a), 3.58 (1 H, dd, J = 6.96, 11.62, H-6'b), 3.36 (1 H, t, J = 8.72, H-3'), 3.26 (1 H, m, H-5'), 3.20 (1 H, d, J = 1.62 Hz, H-4′), 3.19 (1 H, d, J = 1.45, H-2'), 2.75 (1 H, dd, J = 5.99, 9.60 Hz, H-17), 2.52 (1 H, m, H-2), 2.42 (1 H, m, H-16a), 2.35 (1 H, m, H-6a), 2.29 (1 H, m, H-6b), 2.28 (3 H, s, H-21), 2.17 (1 H, s, H-7a), 2.11 (1 H, m, 1a), 1.80 (1 H,m, H-8), 1.74 (1 H, m, 1b), 1.70 (1 H, m, 16b), 1.65 (1 H, m, 12a), 1.58 (1 H, m, 11a), 1.50 (1 H, m, 12b), 1.49 (1 H, m, 7a), 1.48 (1 H, m, 11b), 1.24 (3 H, s, 19), 1.05 (3 H, s, 18). 13C-NMR (CD3OD) δ: 215 (C-20), 201.4 (C-3), 174.1 (C-5), 122.5 (C-4), 100.5 (C-1′), 82.5 (C-14), 77.04 (C-5'), 76.4 (C-15), 76.0 (C-3′), 73.93 (C-2′), 70.73 (C-4'), 61.83 (C-6'), 59.69 (C-17), 49.4 (C-9), 40.9 (C-8), 38.77 (C-10), 38.3 (C-12), 36.0 (C-1), 35.28 (C-16), 33.9 (C-6), 33.7 (C-2), 30.2 (C-21), 29.3 (C-7), 20.60 (C-11), 16.89 (C-19), 15.07 (C-18). HR-ESI-MS (positive ion) m/z 531.25366 [M+Na]+ (Calcd for C27H40O9Na ).
White powder; 1H-NMR (CD3OD) δ:7.68 (1 H, d, J = 15.80 Hz, H-7'), 7.22 (1 H, d, J = 1.92 Hz, H-2'), 7.11 (1 H, dd, J = 1.92, 8.03 Hz, H-6'), 6.83 (1 H, d, J = 8.03, H-5'), 6.78 (2 H, s, H-2,H-6), 6.69 (1 H, d, J = 15.76 Hz, H-7), 6.43 (1 H, d, J = 15.80 Hz, H-8'), 6.37 (1 H, dd, J = 6.15, 15.76 Hz, H-8), 5.23 (1 H, d, J = 1.48 Hz, H1'''), 5.17 (1 H, d, J = 7.33Hz, H1''), 4.85 (2 H, dd, J = 1.06, 6.23 Hz, H-9), 4.00 (1 H, m, H-2'''), 3.91 (3 H, s, OMe at C-3'), 3.87 (6 H, s, 2 OMe at C-3,5), 3.74 (1 H, m, H6''a), 3.70 (1 H, m, H-2'', H-5''), 3.69 (1 H, m, H-3'''), 3.62 (1 H, m, H-6''b) 3.55 (1 H, t, H3''), 3.47 (1 H, m, H-5'''), 3.46 (1 H, m,H-4''), 3.35 (1 H,m, H-4'''), 1.11 (3 H, d, J = 6.36 Hz, H-6'''). 13C-NMR (CD3OD) δ: 168.8 (C-9'), 153.21 (C-3, C-5), 149.33 (C-3'), 148.12 (C-4'), 145.72 (C-7'), 133.49 (C-1), 133.48 (C-7), 132.9 (C-4), 126.43 (C-1'), 122.79 (C-8, C-6'), 115.3 (C-5'), 113.99 (C-8'), 110.4 (C-2'), 104.21 (C-2,C-6), 101.13 (C-1'', C-1'''), 78.6 (C-2''), 78.56 (C-5''), 78.49 (C-3'''), 77.7 (C-3''), 76.1 (C-4'''), 68.43 (C-5'''), 64.64 (C-9), 61.1 (C-6''), 55.66 (2 OMe at C-3,5), 55.5 (OMe at C-3'), 16.23 (C-6'''). HR-ESI-MS (negative ion) m/z 693.24048 [MH]−(Calcd for C33H41O16).
To identify the compounds responsible for the cholinesterase inhibitory activity, the method described previously by Yang et al. was used.8 Acetylcholinesterase (500 U) was dissolved in 500 mL of 0.05M Tris-HCl buffer at pH = 7.8 and 500 mg of Bovine Serum Albumin (BSA) were added to the solution. The stock solution was kept at 4℃. 1-Naphthyl acetate (150mg) was dissolved in a mixture of 40 mL ethanol and 60 mL distilled water. A solution of Fast Blue B salt (50 mg) in distilled water (100 mL) was prepared just before use. Samples were applied to a silica gel TLC plate (10 × 10 cm) and migration was conducted with CHCl3/MeOH/H2O (90:10:1). After complete removal of the solvents, the plate was sprayed with the enzyme and 1-naphthyl acetate subsequently. The TLC plate was then thoroughly dried again before incubation at 37 oC for 20 min. The plate was laid flat on plastic plugs in a plastic tank containing water, so that the atmosphere was kept humid, but without wetting the plate. Then the solution of Fast Blue Salt was sprayed onto the TLC plate, giving a purple coloration except where the acetylcholinesterase was inhibited (white spots). Galantamine was used as a reference compound.
Acetylcholinesterase inhibition was determined spectrophotometrically using acetylthiocholine iodide as substrate as described by an Ellman modified method.11 In this method, 20 µL of an acetylcholinesterase solution (final concentration 0.037U/mL in 0.1M phosphate buffer pH 7.4) were added to 227.3 µL of Ellman's reagent (final concentration of 0.15 mM in 0.1M phosphate buffer pH 7.4) and 2.7 µL of test compound solutions in DMSO. Then, 20 µL of an acetylthiocholine iodide (ATCI) solution solubilized in demineralized water (final concentration 0.22 mM) were added to initiate the enzymatic reaction. The final assay volume was 270 µL in each well. The plate was shaken for 2 s and the absorbance was measured at 412 nm after 30 minutes incubation at 25℃ using a microplate spectrophotometer. For control assays, test compound solutions were replaced by the solvent used in each well, i.e. 1% DMSO. The butyrylcholine iodide and the butyrylcholinesterase were used as substrate and enzyme respectively for BChE assay, all the other reagents and conditions being the same as for the AChE assay. Three independent experiments were performed for each compound. Galantamine was used as positive control. The percentage of ChE inhibition was calculated as follows: where Ac = absorbance of the control solution
As = absorbance of the sample
Molecular docking was performed on most purified compounds (1 - 3 and 6 - 8) and galantamine in order to understand their binding interactions with AChE and BChE active sites. In this purpose, the Glide 6.3 algorithm, in which the target atoms are fixed and the ligands are flexible, was used. Compounds were built using MarvinSketch, whereas Crystal structures of human AChE and BChE, at 2.0 Å resolution, were downloaded from Protein Data Bank (PDB ID: 4M0E and 2XQF respectively).1213 Only their catalytic chain A was kept and then prepared for docking using VMD software14 and Schrodinger's protein preparation wizard.15 This wizard served to add all hydrogen atoms and to control the protonation state and the side chains orientations, especially those of catalytic triad residues of AChE and BChE. VMD software allowed defining the binding sites of enzymes by selecting all residues with at least one heavy atom within 6 Å from the ligand of the target crystal structure. Docking calculations were done with default parameters in a stepwise manner with GLIDE Standard Precision (SP). The resulting poses were ranked according to their GlideScore, which was given as binding energy ΔG (kcal/mol); the lowest energy value is considered as the highest binding.
The best docked conformation was selected and analyzed using Maestro software version 11.3 of the Schrödinger suite.
The extracts obtained from the aerial parts of S. argel were subjected to enzyme inhibitory assays against AChE and BChE. The chloroform extract was more active than the crude extract against both enzymes (Table 1) and its AChE activity was higher than those recently reported for a species from the same family of S. argel (Vinca minor L.).16 Therefore, this chloroform extract was chosen for further phytochemical studies. We also confirmed these results with a TLC bioautography assay and revealed several active compounds in the crude extract and the chloroform extract (Fig. 1). Using the same assay during a bio-guided fractionation, we isolated 8 compounds from the chloroform extract (Fig. 2). Their structures were elucidated by spectroscopic methods including NMR (1D and 2D), HR-ESI-MS and by comparison with published data, confirming the proposed structures of kaempferol-3-O-glucopyranoside (1),17 kaempferol (2),18 kaempferol-3-glucopyranosyl-(1→6)-rhamnopyranose (3),19 p-hydroxybenzoic acid (4),20 dehydrovomifoliol (5),21 14,15-dihydroxypregn-4-ene-3,20-dione (6),6 14,15-dihydroxy-pregn-4-ene-3,20-dione-15β-D-glucopyranoside (7),6 and solargin I (8)22 respectively. To our best knowledge, this is the first report of compounds 4 and 5 in the Apocynaceae family.
Compounds 1 - 3 and 6 - 8 were tested on both AChE and BChE with a microplate assay (Table 2). All of them were slightly active (> 20% inhibition) on at least one enzyme. We did not have sufficient amounts of compounds 4 and 5 for the microplate assay. Nevertheless, p-hydroxybenzoic acid (4) isolated from an aquatic plant (Nelumbo nucifera) has demonstrated good and noncompetitive AChE inhibition (IC50 = 20.07 µM) and presented less potent BChE inhibition (IC50 = 62.29 µM).23 Furthermore, dehydrovomifoliol (5) isolated from the dichloromethane extract of Gloiopeltis furcata (a marine algae), had a moderate activity on AChE (IC50 = 3.09 µg/mL) and a weak activity on BChE (IC50 = 95.08 µg/mL).24 Together, these results indicate that the phenolic acid (4) and the volatile norisoprenoid (5) could contribute to the ChE inhibitory activity of S. argel extracts.
Compounds 6 and 7 were among the most active on AChE, although these activities seemed lower compared to some other pregnanes from plants. For instance, Lee et al. reported that several pregnane glycosides isolated from the roots of Cynanchum atratum (Apocynaceae), cynatroside A, B and C, were potent AChE inhibitors (IC50 of 6.4, 3.6 and 52.3 µM respectively).4 Apart from compound 6, all the active compounds were more potent on BChE compared to AChE. This selectivity in the activity towards the cholinesterase could be attributed, at least in part, to the structural difference of the aromatic gorge of the two enzymes. BChE also inactivates the neurotransmitter ACh and could be a target for AD treatment by improving cognition and modulating pathological markers of this disease.24 Studies revealed that the BChE level in specific brain regions is increased with progression of AD while the AChE level decreases.25 There is currently no BChE selective inhibitor being employed clinically.26 Hence, a BChE selective inhibitor might be useful in the later stages of AD.
Among all the isolated compounds, kaempferol (2) was the most active on both enzymes. Our results are consistent with the percentage of AChE inhibition by kaempferol previously described (percentage of inhibition at 50 µM= 14.6).27 In addition, Bahrani et al.28 tested kaempferol in vitro against AChE and in vivo in mice and they observed a significant inhibitory activity and an improved memory, respectively. Epidemiological evidence suggests that higher consumption of flavonoids is associated with lower incidence of AD.29 Similarly, S. argel contains multipotent components that might be useful in the treatment of AD.
The molecular docking studies were performed for compounds tested on the microplate assay using the Glide program. The results of molecular docking between enzymes and ligands are shown in Table 2. The experimental data for ChE inhibitory activities in vitro showed a satisfactory agreement with the molecular docking results (Table 2). Among the tested compounds, 8 possessed the lowest free energy of binding against both enzymes (-6.001 for AChE and -7.254 for BChE) while the free energy of binding for compound 2 was the highest (-9.869 for AChE and -9.346 for BChE). The most promising inhibitor 2 from in vitro and in silico assays was selected for further investigation of its binding mode into AChE and BChE at the active site 3D space to get insight into the intermolecular interactions using the Glide program. As shown in Fig. 3, compound 2 mainly covers the peripheral site (PAS) in the entrance of AChE active gorge, making a hydrogen bond with Phe 295 and three π-π stackings with Tyr 341 and Trp 286. However, this compound covers BChE catalytic site in a rational orientation, making 2 π-π stackings with Trp 82 and His 438, which is considered as a critical member of BChE catalytic triad (Fig. 3).11 This interaction with the catalytic site can explain the selectivity of compound 2 towards BChE instead of AChE in the in vitro assay.
The bioguided fractionation of S. argel extracts led to the isolation of three flavonoids (1, 2 and 3), a phenolic acid (4), a norisoprenoid (5), two steroids (6, 7) and an acylated phenolic glycoside (8). To the best of our knowledge, this is the first report on the cholinesterase inhibitory activity of S. argel extracts and of four of its compounds: the original pregnanes14,15-dihydroxy-pregn-4ene-3,20-dione (6) and 14,15-dihydroxy-pregn-4-ene-3,20-dione-15-β-D glucopyranoside (7), kaempferol-3-glucopyranosyl-(1→6)-rhamnopyranose (3), and solargin I (8). In addition, all of these compounds were shown or reported to inhibit, at least slightly (> 20%), the activity of one cholinesterase. Among them, two isolated flavonoids (2 and 3) were active on both AChE and BChE with a slight specificity for the latter (> 50% inhibition). Molecular modeling study confirmed that compound 2 showed more potent inhibitory activities than other compounds against both enzymes. Since a dual AChE and BChE inhibitor demonstrates symptomatic efficacy in AD and flavonoids can be multipotent agents in combating AD,30 S. Argel could deserve further investigation for helping patients with this neurodegenerative disease.
Figures and Tables
Fig. 3
Binding mode prediction of compound 2 into the entire AChE (A) and BChE (B) active pocket. Purple arrows head from the donor to the acceptor of hydrogen bonds and green lines π-π stackings. The gray circle dimensions are proportional to the accessibility to the solvent.
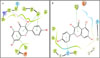
Acknowledgments
The authors wish to thank the cooperation program between Algeria and France (PROFAS B+) for the financial support of this research, and two analytical platforms: LARMN (University of Lille, France, Pr. N. Azaroual) and CUMA (University of Lille, France, Pr. J.F. Goossens) for access to equipment. We are also thankful to the members of these platforms as well as J. Samaillie for their skillful technical assistance.
References
1. Machado LP, Carvalho LR, Young MCM, Cardoso-Lopes EM, Centeno DC, Zambotti-Villela L, Colepicolo P, Yokoya NS. Rev Bras Farmacogn. 2015; 25:657–662.
2. Orhan IE, Orhan G, Gurkas E. Mini Rev Med Chem. 2011; 11:836–842.
3. Zemek F, Drtinova L, Nepovimova E, Sepsova V, Korabecny J, Klimes J, Kuca K. Expert Opin Drug Saf. 2014; 13:759–774.
4. Lee KY, Sung SH, Kim YC. Helv Chim Acta. 2003; 86:474–483.
5. Kamel MS, Ohtani K, Hasanain HA, Mohamed MH, Kasai R, Yamasaki K. Phytochemistry. 2000; 53:937–940.
6. Ounaissia K, Pertuit D, Mitaine-Offer AC, Miyamoto T, Tanaka C, Delemasure S, Dutartre P, Smati D, Lacaille-Dubois MA. Fitoterapia. 2016; 114:98–104.
7. Shafek RE, Shafik NH, Michael HN. Asian J Plant Sci. 2012; 11:143–147.
8. Yang Z, Zhang X, Duan D, Song Z, Yang M, Li SJ. Sep Sci. 2009; 32:3257–3259.
9. Plaza A, Perrone A, Balestrieri C, Balestrieri ML, Bifulco G, Carbone V, Hamed A, Pizza C, Piacente S. Tetrahedron. 2005; 61:7470–7480.
10. Ibrahim ME, Ahmed SS, El-Sawi SA, Khalid KA. J Essent Oil Bear Pl. 2014; 17:629–632.
11. Di Giovanni S, Borloz A, Urbain A, Marston A, Hostettmann K, Carrupt PA, Reist M. Eur J Pharm Sci. 2008; 33:109–119.
12. Cheung J, Gary EN, Shiomi K, Rosenberry TL. ACS Med Chem Lett. 2013; 4:1091–1096.
13. Wandhammer M, Carletti E, Van Der Schans M, Gillon E, Nicolet Y, Masson P, Goeldner M, Noort D, Nachon F. J Biol Chem. 2011; 286:16783–16789.
14. Humphrey W, Dalke A, Schulten K. J Mol Graph. 1996; 14:33–38.
15. Schrödinger L. Schrödinger Release 2015-1: Maestro (version 10.1). N.Y.: 2015.
16. Lobbens ES, Vissing KJ, Jorgensen L, van de Weert M, Jäger AK. J Ethnopharmacol. 2017; 200:66–73.
17. Wei Y, Xie Q, Fisher D, Sutherland IA. J Chromatogr A. 2011; 1218:6206–6211.
18. Park JS, Rho HS, Kim DH, Chang IS. J Agric Food Chem. 2006; 54:2951–2956.
19. Budzianowski J. Phytochemistry. 1990; 29:3643–3647.
20. Cho JY, Moon JH, Seong KY, Park KH. Biosci Biotechnol Biochem. 1998; 62:2273–2276.
21. Schievano E, Stocchero M, Morelato E, Facchin C, Mammi S. Metabolomic. 2012; 8:679–690.
22. Kamel MS. Phytochemistry. 2003; 62:1247–1250.
23. Jung HA, Jung YJ, Hyun SK, Min BS, Kim DW, Jung JH, Choi JS. Biol Pharm Bull. 2010; 33:267–272.
24. Fang Z, Jeong SY, Jung HA, Choi JS, Min BS, Woo MH. Chem Pharm Bull. 2010; 58:1236–1239.
25. Darvesh S. Curr Alzheimer Res. 2016; 13:1173–1177.
26. Mehta M, Adem A, Sabbagh M. Int J Alzheimers Dis. 2012; 2012:728983.
27. Guo AJY, Xie HQ, Choi RCY, Zheng KYZ, Bi CWC, Xu SL, Dong TTX, Tsim KWK. Chem Biol Interact. 2010; 187:246–248.
28. Bahrani H, Mohamad J, Paydar MJ, Rothan HA. Curr Alzheimer Res. 2014; 11:206–214.
29. Wan Othman WNN, Liew SY, Khaw KY, Murugaiyah V, Litaudon M, Awang K. Bioorg Med Chem. 2016; 24:4464–4469.
30. Kandiah N, Pai MC, Senanarong V, Looi I, Ampil E, Park KW, Karanam AK, Christopher S. Clin Interv Aging. 2017; 12:697–707.