Abstract
Purpose
Materials and Methods
Results
Figures and Tables
Fig. 1
miR-140-3p expression according to HSC-T6 cell activation status. Expression of miR-140-5p was measured in HSC-T6 cells by qPCR. (A) Cells were incubated with 5% FBS for 48 h. The control was FBS-free cells. (B) Cells were treated with 10 ng/mL of PDGF-BB (PDGF) for 48 h. The control was no treatment cells. (C) Cells were exposed to TGF-β1 for 48 h. (D) Levels of the marker of hepatic stellate cell activation, α-SMA in TGF-β1 treated cells were detected using western blot assay. The control was no treatment cells. All experiments were performed in triplicate, and *p<0.05, †p<0.01. PDGF, platelet derived growth factor; α-SMA, α-smooth muscle actin; TGF-β1, transforming growth factor β1.

Fig. 2
miR-140-3p knockdown reduces cell fibrosis in HSC-T6 cells. miR-140-3p knockdown was obtained after transfection of anti-miR-140-3p into TGF-β1-induced HSC-T6 cells. (A) Relative expression levels of miR-140-3p. (B) Cell proliferation was measured with cell proliferation assay. (C) Cell apoptosis was measured on flow cytometry, and apoptosis rate was calculated. (D) Expressions of PCNA, caspase 3, and cleaved caspase 3 (cle-caspase 3) were detected using Western blot assay, and the gray intensity was analyzed. (E) Cell migration was determined using transwell assay (0.5% crystal violet, ×100). (F and G) Expressions of α-SMA, desmin, COL1A1, and fibronectin were detected using Western blot assay, and the gray intensity was analyzed. All experiments were compared with cells transfected of anti-NC and performed in triplicate, *p<0.05. TGF-β1, transforming growth factor β1; α-SMA, α-smooth muscle actin; anti-miR-140-3p, miR-140-3p inhibitor; PCNA, proliferating cell nuclear antigen; COL1A1, collagen type 1A1.
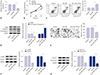
Fig. 3
PTEN is negatively regulated by miR-140-3p target binding in HSC-T6 cells. (A) Potential target sites (red) on PTEN 3′UTR were predicted on TargetScan software and mutated. (B) Luciferase reporter assay was conducted to identify the binding sites. Relative luciferase activity was significantly reduced in cells transfected with PTEN wild type (wt) 3′UTR and miR-140-3p mimic (miR-140-3p). (C) RIP were performed. Dramatically high expression of PTEN were obtained from Ago2, separately. (D) Effect of miR-140-3p on expression of PTEN in cells. Relative expression levels of PTEN were evaluated using Western blotting, and the gray intensity was analyzed. All experiments were performed in triplicate, *p<0.05. RIP, RNA immunoprecipitation; PTEN, phosphatase and tensin homolog deleted on chromosome.

Fig. 4
PTEN silencing blocks the effect of miR-140-3p knockdown in activated HSC-T6 cells. Silencing expression of PTEN was obtained after transfection with specific siRNA against PTEN (siPTEN) into TGF-β1-induced HSC-T6 cells. Effects of siPTEN on anti-miR-140-3p action were measured. (A) Expression levels of PTEN in cells transfected with anti-miR-140-3p and siPTEN or not. (B and C) Cell proliferation and apoptosis was analyzed in cells transfected with anti-miR-140-3p and siPTEN or not. (D) Expressions of PCNA, caspase 3, and cle-caspase 3 were analyzed in cells transfected with anti-miR-140-3p and siPTEN or not, and the gray intensity was calculated. (E) Cell migration was determined using transwell assay after transfection with anti-miR-140-3p and siPTEN or not. (F and G) Expressions of α-SMA, desmin, COL1A1, and fibronectin were analyzed in cells transfected with anti-miR-140-3p and siPTEN or not, and the gray intensity was calculated. All experiments were performed in triplicate, *p<0.05. TGF-β1, transforming growth factor β1; PTEN, phosphatase and tensin homolog deleted on chromosome 10; PCNA, proliferating cell nuclear antigen; α-SMA, α-smooth muscle actin; COL1A1, collagen type 1A1.

Fig. 5
miR-140-3p knockdown inactivates the AKT/mTOR signaling pathway, which is abolished by PTEN silencing. Effects of siPTEN on anti-miR-140-3p-inactivated signaling pathway were measured. Expressions of p-AKT and p-mTOR and PTEN were detected using Western blot assay, and the gray intensity was calculated. All experiments were performed in triplicate. *p<0.05. PTEN, phosphatase and tensin homolog deleted on chromosome.
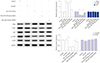
ACKNOWLEDGEMENTS
Notes
AUTHOR CONTRIBUTIONS
Conceptualization: Shi-Min Wu.
Data curation: Tian-Hong Li.
Formal analysis: Shi-Min Wu, Tian-Hong Li.
Funding acquisition: Hao Yun.
Investigation: Shi-Min Wu, Tian-Hong Li.
Methodology: Hao Yun.
Project administration: Shi-Min Wu.
Resources: Hao Yun.
Software: Hong-Wu Ai.
Supervision: Tian-Hong Li.
Validation: Hong-Wu Ai.
Writing—original draft: Ke-Hui Zhang.
Writing—review & editing: Shi-Min Wu, Tian-Hong Li.
References



































