Abstract
Objective
The purpose of this study was to investigate the relationship between the facial skeletal patterns and the shape of the mandibular symphysis in adults with malocclusion by using a structural equation model (SEM).
Methods
Ninety adults who had malocclusion and had records of facial skeletal measurements performed using cone-beam computed tomography were selected for this study. The skeletal measurements were classified into three groups (vertical, anteroposterior, and transverse). Cross-sectional images of the mandibular symphysis were analyzed using generalized Procrustes and principal component (PC) analyses. A SEM was constructed after the factors were extracted via factor analysis.
Results
Two factors were extracted from the transverse, vertical, and anteroposterior skeletal measurements. Latent variables were extracted for each factor. PC1, PC2, and PC3 were selected to analyze the variations of the mandibular symphyseal shape. The SEM was constructed using the skeletal variables, PCs, and latent variables. The SEM showed that the vertical latent variable exerted the most influence on the mandibular symphyseal shape.
The mandibular symphysis is located at the midline of the frontal part of the mandible, and constitutes the chin and frontal part of the lower face.1 Several factors affect the growth and shape of the mandibular symphysis, such as neuroskeletal balance,2 masseter muscle thickness,3 mandibular plane angle,4 overbite,256 lower incisor angle,7 occlusal function,8 and inheritance.9 The shape of the mandibular symphysis plays a significant role in aligning the mandibular incisors during orthognathic surgery and orthodontic treatment. Evaluation of the shape of the mandibular symphysis before orthodontic treatment is important to prevent possible iatrogenic damage.1011
Aki et al.12 reported that patients with a large symphysis could be treated using increased protrusion of the anterior teeth with a higher probability of nonextraction treatment. However, patients who had a long and narrow symphysis were more likely to be treated using orthodontic extraction treatment to compensate for their arch length discrepancy. Clinicians classify the anterior and posterior growth patterns of the mandible on the basis of the size and shape of the mandibular symphysis.121314 Compared to the treatment of the posterior growth pattern, which is related to mandibular retraction, the treatment of the anterior growth pattern tends to require orthodontic treatment and orthognathic surgery. Thus, the shape of the suture affects both the overall treatment plan and the classification of the facial skeletal morphology.
By using two-dimensional (2D) cephalometric analysis, many studies showed that the shape of the mandibular symphysis correlates with the facial skeletal pattern.115 Retrospective cephalometric analysis showed that individuals with brachyfacial patterns had more hard tissue on their chins, and individuals with a larger mandibular plane angle showed higher growth rate of the lower anterior facial height.1
Many studies have investigated the relationship between the mandibular symphysis morphology and the 2D facial skeletal pattern. However, these studies had some limitations because they did not consider the three-dimensional (3D) facial skeletal pattern. These studies evaluated the facial skeletal pattern for each cross-sectional dimension. However, a statistical model that demonstrates the relationship between the form of the mandibular symphysis and the 3D facial skeletal pattern is needed for extensive understanding of the maxillofacial complex morphology.
Cone-beam computed tomography (CBCT) imaging allows for 3D analysis of the facial skeletal pattern, which cannot be performed using lateral or posteroanterior cephalography. CBCT reconstructs the 3D facial skeletal pattern and presents coordinate points in three dimensions. Identifying the measurement points on the different planes could reduce image distortion or errors that occur in cephalometric radiographs. It is also possible to reconstruct a cross-section of the jaw that is based on a reference plane and established on 3D measurements. Moreover, CBCT allows for both precise measurement and acquisition of reliable data by using the same landmarks.
Data analysis of multivariate variables, however, is complicated. Therefore, a statistical method is required to simplify the relationship among the variables. A structural equation model (SEM) allows for simultaneous investigation and estimation of multiple interdependent relationships. It also allows for analyses that consider the measurement error related to the observed variables, and conceptually expresses the relationship between the variables by classifying the latent variables. These models demonstrate the relationship between the variables clearly and express the regression weights of individual relationships as structural coefficients.
Therefore, this study aimed to visualize the relationship between the vertical, horizontal, and transverse facial skeletal patterns, delineate the shape of the mandibular symphysis via a SEM, and analyze the relationship between the facial skeletal pattern and the cross-sectional morphology of the mandibular symphysis. Specifically, the goals were to 1) obtain the mandibular symphysis morphology via geometric morphometrics and perform a principal component (PC) analysis via cross-sectional CBCT imaging of patients with skeletal malocclusion; 2) extract the facial skeletal factors as latent variables by using factor analysis; and 3) analyze the relationship between the facial skeletal pattern and the mandibular symphysis morphology via a SEM.
This study used CBCT data of adult patients who visited the Pusan National University Dental Hospital between January 2010 and December 2017, and whose chief complaints were skeletal malocclusion. In total, 90 patients (39 male and 51 female) were included, and their average age was 22.96 ± 4.5 years. Those who had systemic diseases, trauma, surgical history, or maxillofacial malformations were excluded. This study was reviewed and approved by the institutional review board of the Pusan National University Dental Hospital (PNUDH-2016-025).
CBCT (Pax-Zenith3D; Vatech Co., Ltd., Hwaseong, Korea) was performed for all patients under the same conditions: 90 kVp, 10 mA, scan time of 24 seconds, voxel size of 0.3 mm, and FOV of 20 × 19 cm. The maxillofacial complex morphology was analyzed and measured using a 3D analysis software (InVivo6; Anatomage Inc., San Jose, CA, USA).
The CBCT images were reoriented on two 3D reference planes (sagittal and horizontal), considering the nasion as the origin point (0, 0, 0). The horizontal reference plane was the Frankfort plane passing through the left and right porion and the right orbitale, while the sagittal reference plane crossed the Frankfort plane perpendicularly and passed through the origin point and the sella.
The definitions of the facial skeletal measurements, which were obtained using CBCT, are presented in Table 1. After the CBCT images were reoriented in uniform positions, the measurements were obtained and confirmed in three planes (transverse, sagittal, and frontal planes).
The measurements were used as observed variables to analyze the facial skeletal morphology and were classified as three factors: horizontal, vertical, and anteroposterior factors. The definitions of the factors are presented in Table 2.
The horizontal factor included the distance measurements of the orbit, zygoma, maxilla, and mandible. The upper and lower parts of the orbit were measured as the distances between the left and right frontozygomatic point and orbitale, respectively. For the zygoma, the distance between the left and right zygomatic arch was measured. For the maxilla, the distance between the left and right jugal point was measured. For the mandible, the distances of the condyle and mandibular body were taken as follows: for the condyle, the distance between the left and right condylion, and for the mandibular body, the distance between the left and right gonion, located at the intersection of the ramus and mandibular body, or the left and right antegonion.
The vertical factor included the distance measurements of the anterior facial height, posterior facial height, and ramus length. The total anterior facial height was the distance between the nasion and menton; superior anterior facial height was the distance between the nasion and anterior nasal spine; and posterior facial height was the distance between the sella and gonion. The vertical height of the ramus was measured from the condylion to gonion.
The anteroposterior factor included the distance measurements of the cranial base, maxilla, and mandible. The length of the cranial base consisted of the anterior cranial base, which was the distance from the sella to nasion, and the total cranial base, which was the distance from the Ba to nasion. The length of the maxilla was the distance from the anterior nasal spine, which was the most anterior point of the maxilla to the posterior nasal spine, which was the most posterior point of the maxilla. The length of the mandible was taken from the menton to gonion.
The 3D mandibular plane consisted of the left and right gonion and menton, and was set as the reference plane for repositioning the CBCT images. Cross-sectional images were acquired from the section that crossed the mandibular symphysis perpendicularly, and the center of the left and right mandibular central incisors. All images were arranged such that the labial area was located on the right side and the lingual area was on the left.
The morphology of the external and internal cortices was acquired, and each bone had 2 landmarks and 21 semi-landmarks (Figure 1). Landmarks 1 and 2 were located on the uppermost part of the mandibular labial and lingual cortices. The outermost part of the cortical bone was defined as the external cortical bone, and the border between the internal cancellous bone and the cortical bone was defined as the internal cortical bone. The 21 semi-landmarks were positioned to obtain the morphology of the internal and external cortices. The semi-landmarks were located on the outline of each cortical bone and were realigned to be within the same distances.
Facial skeletal measurements of the 90 patients were recorded as observed variables and were categorized into three groups: vertical, horizontal, and anteroposterior. Before verifying the effect of the facial skeletal shape on the mandibular symphysis morphology, the factors were extracted using factor analysis. The factors were used as latent variables to establish the SEM.
Stastical analyses were performed using Amos ver. 24.0 (IBM, Armonk, NY, USA).
The 23 points (2 landmarks and 21 semi-landmarks) acquired from the external and internal cortices of the mandibular symphysis were superimposed based on generalized Procrustes analysis. The mean shape of the symphysis was drawn, excluding the effects of position, size, and direction.
Variations in the cross-sectional shape of the mandibular symphysis were analyzed using PC analysis. The PCs were extracted to have 70% cumulative proportion, and the dimension was reduced during the process of extraction.
A SEM was used to analyze the relationship between the facial skeletal factors and the morphologic variation of the symphysis. The relationships between the variables, including the latent and observed variables, could be analyzed using the SEM. Factor relationships were analyzed using the SEM based on the latent variables, which were extracted from the facial skeletal patterns and PC analysis of the symphysis morphology. The reliability of the constructed model was evaluated using the goodness-of-fit index. The SEM is presented as a path diagram.
The observed variables of the facial skeletal pattern were analyzed using confirmatory factor analysis to extract the latent variables of the transverse, vertical, and anteroposterior factors. The relationship of the extracted latent variables was derived from the SEM. The model was considered statistically significant at a significance level of 5%. Relationships between the latent variables are presented in Tables 3 and 4. The intraexaminer reliabilities of the measurements were very high according to the intraclass correlation coefficient (mean of 0.964).
PC analysis was performed on the 23 landmarks of the external and internal cortices of the 90 mandibular symphyses. PC1, PC2, and PC3 were used to obtain 70% cumulative proportion. The relative proportions of PCs 1–3 of the internal cortices were 50.18%, 15.19%, and 12.00%, respectively. The relative proportions of PCs 1–3 of the external cortices were 52.38%, 14.21%, and 12.66%, respectively. The cumulative proportions of PCs 1–3 of the internal and external cortices were 77.37% and 79.26%, respectively.
The PCs of morphologic variances on the external and internal cortices are presented in Figures 2 and 3. The mean shape of the internal cortical bone narrowed from the upper third to the middle third and then widened. The width increased slightly as it progressed from the top to the bottom, and demonstrated a generally curved outline. For the morphologic variation of the internal cortical bone in PC1, when observed in the range of 3 times its standard deviation (3SD), the width from the middle third to the lower part was twice the mean morphology at +3SD, but narrowed at −3SD with the middle third being the narrowest part. For the morphologic variation of the internal cortical bone in PC2, the width of the lower third of the lingual part increased and projected lingually; thus, the outline was mostly curved at +3SD, while the width of the upper third decreased slightly and the lingual part was mostly straight at −3SD. For the morphologic variation of the internal cortical bone in PC3, the width of the lower third decreased with intensified lingual curve angularity. Subsequently, the overall outline was curved with a generally uniform width at +3SD, while the width of the lower third was wider than the mean shape and with an uneven straight line on the lingual part at −3SD; thus, the overall outline was straight with a wide lower part.
The mean shape of the external cortical bone widened downward and curved toward the lingual part. For the morphologic variation of the external cortical bone in PC1, the overall width increased from the middle third to the lower third with the middle third being twice as wide at +3SD. However, the width of the middle third was half as wide as the mean morphology with a tendency of decreasing as it proceeded downwards to the lower third at −3SD. For the morphologic variation of the external cortical bone in PC2, the lingual width of the lower third decreased; thus, the lingual curve angularity increased at +3SD. However, the lingual width of the lower third increased; thus, the outline was almost linear at −3SD. For the morphologic variation of the external cortical bone in PC3, the width of the part between the middle third and the lower third increased; thus, the lingual part folded at +3SD. However, the width was narrower than the mean morphology; thus, the overall outline showed an S-shaped curve that gently projected toward the labial part at −3SD.
The relationship between the facial skeletal factors of the vertical, transverse, and anteroposterior latent variables, as delineated using confirmatory factor analysis, is shown in Table 4 and Figure 4. Dimension reduction was attempted by extracting the factors from the PCs of the internal and external cortices of the mandibular symphysis; however, the factors could not be extracted from PC1 of the internal and external cortices. The internal and external cortices of PC1 were, therefore, treated as independent factors. Variation 2 was extracted from the external cortical bone of PC2 and the internal cortical bone of PC3, and variation 3 was extracted from the internal cortical bone of PC2 and the external cortical bone of PC3. Confirmatory factor analysis showed significance at the 5% level. The extracted factors, as well as variations 2 and 3, showed a weak correlation (correlation coefficient = 0.195).
Table 5 presents the regression weights of the latent variables in the SEM. The anteroposterior latent factor was the most influential factor, because the factor not only affected PC1 of the internal and external cortices but also variations 2 and 3. The vertical latent variable was the second most influential factor as it affected PC1 of the internal and external cortices. The transverse latent variable affected variations 2 and 3.
The total effects of the SEM are presented in Tables 6 and 7. The total effects of PC1 of the internal cortical bone, from the vertical and anteroposterior latent variables were 0.142 and 0.142, respectively. However, the total effects of PC1 of the external cortical bone from the vertical and anteroposterior latent variables and variation 2 were 0.167, 0.158, and 0.226, respectively. The total effects of variation 2 were 0.038 and −0.047 from the transverse and anteroposterior latent variables, respectively. The total effects of variation 3 were 0.027 and −0.029 from the horizontal and anteroposterior latent variables, respectively. The direct effects were the same as the total effects, with the exception of the direct effects of the external cortical bone of PC1 from the anteroposterior latent variable, which was 0.168. The indirect effect on the external cortical bone of PC1 from the anteroposterior latent variable was −0.011.
The mandibular symphysis morphology is important in orthodontic treatment planning for adult patients who have stopped growing. The cortical bone of the symphysis is thinner than the other mandibular parts, and this becomes an anatomically limiting factor for tooth movement. Patients with narrow mandibular symphyses have a higher probability of iatrogenic injuries, such as gingival recession or dehiscence; therefore, tooth movement should be cautiously performed during orthodontic treatment procedures. Subsequently, it is important to establish an appropriate treatment plan for individual patients based on an evaluation of the morphology of their mandibular symphyses.
In this study, the relationship between the shape of the mandibular symphysis and the facial skeletal pattern was investigated using a SEM with 3D analysis. The facial skeletal pattern was analyzed in three dimensions by using the vertical, transverse, and anteroposterior factors. These factors were extracted through observed variables that were categorized according to the factors, and the latent variables were then extracted and analyzed.
The latent variables of the facial skeletal patterns had a relationship with each other because the maxillofacial skeletal morphology was not limited to a specific direction but was associated with different parts.1617 This relationship between the latent variables was presented in the SEM, but it exerted minimal influence on the entire model analysis. The final model showed that the relationship between the latent variables was correlational rather than causal.
Analysis of the shape of the mandibular symphysis was based on Procrustes superimposition and principal component analyses. PCs 1, 2, and 3 of the external and internal cortices were extracted from the PC analysis and > 70% of the cumulative proportion was acquired. Dimension reduction was attempted by extracting the latent variables through correlation analysis. According to the relationships, the latent variables were extracted from PCs 2 and 3. Variation 2 was extracted from PC3 of the internal cortical bone and PC2 of the external cortical bone; variation 3 was extracted from PC2 of the internal cortical bone and PC3 of the external cortical bone. The relationship between PC1 of the internal and external cortices, however, was minimal; therefore, it was treated as an independent factor. PC1 of the internal and external cortices lacked morphological similarity, and it was assumed that it was difficult to form a single factor by extracting the latent variables. Therefore, after modifying the study model, the final model analyzed the relationships between the facial skeletal pattern and the mandibular symphysis, which included PC1 of the internal and external cortices as observed variables, and PCs 2 and 3 as the latent variables.
The SEM was constructed with three facial skeletal factors and symphyseal PCs. In the SEM, the influence on the relationships was shown in the total effects, which was the sum of the direct and indirect effects. Most of the total effects were identical to the direct effects, with the exception of the relationship between the anteroposterior factor and PC1 of the external cortical bone, which showed decreased total effects when compared to the direct effects because of the existence of the indirect effects.
The effects of variation 2 on PC1 of the external cortical bone were the largest among the total effects of the SEM. It implies that PCs 2 and 1 of the external cortical bone had a partially positive relationship. Both PCs were of the external cortical bone, and had significant similarities in the lingual cortical bone morphologies, and differences in the labial cortical bone morphologies. PC3 of the internal cortical bone was similar in morphology to that of the external cortical bone, showing a curved outline with a uniform thickness at +3SD and a straight outline with a wider lower part at −3SD.
Among the effects of the skeletal factors on the cortical bone, the vertical factor effect on PC1 of the external cortical bone was the largest, with a value of 0.167. It implies that the overall thickness, including the middle third and lower third, can increase when the vertical factor increases. This also implies that the width of the external cortical bone of the mandibular symphysis could be wider in patients with a strong vertical pattern.
These results are slightly different from those of previous studies. Some studies reported that patients with class III malocclusion with long faces had long and narrow mandibular symphyses.1118 This difference could be attributed to the fact that the previous studies analyzed the influences observed on the facial profiles in a fragmented manner. Another possible reason could be that the previous studies analyzed malocclusion independent of the skeletal pattern, while the present study included the entire facial skeletal pattern.
The total effects of the anteroposterior factor on PC1 of the external cortical bone were also relatively high, with a value of 0.158. The direct effect was 0.168, which was higher than that of the vertical factor. The indirect effects of variation 2 on PC1 of the external cortical bone, which showed a negative value, are considered to decrease the total effects. The influence of variation 2 was necessary to analyze the mandibular symphysis morphology. However, it was not easy to assess the effect from the facial skeletal pattern to the mandibular symphysis morphology, including the effects between the mandibular shapes. Considering the direct effect alone, the width of the external cortical bone of the mandibular symphysis was considered wider as the lateral facial skeleton was wider.
Previous studies categorized malocclusion according to the characteristics of the skeletal patterns, and then analyzed the relationships between the facial skeletal pattern and the mandibular symphysis. Al-Khateeb et al.19 studied the difference in the mandibular symphysis morphology on the basis of the classification of malocclusion. The mandibular symphysis dimensions in class III malocclusion were considered different from those of class I or II malocclusion. The morphology of the alveolar bone of the mandibular symphysis in compensated class III malocclusion resulted from the position of the maxilla and mandible. The size of the mandibular symphysis had a strong correlation with the anterior facial height. Swasty et al.20 studied the relationship between the vertical maxillofacial skeletal morphology and the cross-section of the mandible. The height of the mandibular symphysis was high in the dolichofacial pattern and low in the brachyfacial pattern. The width of the mandibular symphysis was wide in the brachyfacial pattern but narrow in the dolichofacial pattern. A comparison of the lower part with the upper part of the symphysis showed that the lower part was wider than the upper part in the brachyfacial pattern.
Previous studies on 2D maxillofacial skeletal patterns showed a correlation with the cross-section of the mandibular symphysis. However, it was difficult to comprehensively understand the morphology of the facial skeletal patterns that extended in three dimensions. The factors considered in the analysis of 3D skeletal morphology were multivariate variables, which also made it difficult to analyze the effect of individual factors through general statistical techniques.
This study used the SEM not only to understand the tendency of the mandibular symphyseal morphology according to the vertical, transverse, and anteroposterior factors, but also to analyze the influence of these factors. A SEM can simplify the complex relationships between the individual multivariate variables and suggest an intuitively understandable result. It can also extract latent variables, by considering the measurement errors in observed variables, and therefore allow for the analysis of the pure concept of factors. It was useful to discover and analyze the relationship between the concepts by using the constructed model.
The analytical limitation of the SEM was that when extracting the latent variables of the mandibular symphysis, some PCs were extracted as latent variables, while others were suggested as observed variables. Because the extraction of the latent variables was based on the correlation of the observed variables, some factors were considered individual factors with independence. The relationships of the factors were complicated, and although the relationship was simplified through the SEM, there were still difficulties in its clinical interpretation. Additionally, there were relationships between the symphyseal factors. It was necessary to consider the total-both direct and indirect effects in the analysis. The indirect effects between the morphologies of the mandibular symphyses are assumed to decrease the total effects.
Nonetheless, this study was meaningful in its consideration of the overall 3D skeletal morphology and the cross-sectional morphology of the mandibular symphysis. The facial skeletal pattern should be considered in the 3D skeletal morphology, and its influence on morphologic variations of the maxilla and mandible should be analyzed in future studies.
Facial skeletal patterns with skeletal malocclusions were categorized into three factors: vertical, transverse, and anteroposterior. The mandibular symphysis morphology was analyzed using geometric morphometrics and PC analysis. A SEM based on confirmatory factor analysis was used to analyze the relationship between the facial skeletal pattern and the shape of the mandibular symphysis. The results of the SEM demonstrated that the vertical skeletal factor had a higher influence on the morphologic variation of the mandibular symphysis than other factors.
Figures and Tables
Figure 1
Obtaining the landmarks for the mandibular symphysis cross-sectional images. The outline of the mandibular symphysis is considered for the external (A) and internal (B) cortices. Two landmarks (yellow) and 21 semi-landmarks (red) are positioned along the outline of the mandibular cortex.

Figure 2
Principal component (PC) analysis of the internal cortices of the mandibular symphysis.
M, Mean; SD, standard deviation.
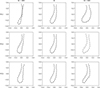
Figure 3
Principal component (PC) analysis of the external cortices of the mandibular symphysis.
M, Mean; SD, standard deviation.
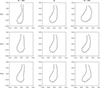
Figure 4
Structural equation model of the skeletal factors and mandibular cortices of the symphysis. The double and dotted arrows show the relationship of the vertical, horizontal, and anteroposterior latent variables.
PC, Principal component.
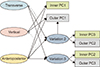
Table 2
Definition of the measurements obtained in this study
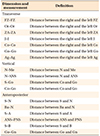
See Table 1 for the definition of each landmark.
ACKNOWLEDGEMENTS
This study was supported by the National Research Foundation of Korea (NRF) grant funded by the Korean government (2018R1D1A1B07042098).
Notes
References
1. Khan MY, Kishore MS, Bukhari SA, Rachala MR, Sashidhar NR. Alveolar and skeletal chin dimensions associated with lower facial height among different divergent patterns. J Clin Diagn Res. 2016; 10:ZC75–ZC80.


2. Haskell BS. The human chin and its relationship to mandibular morphology. Angle Orthod. 1979; 49:153–166.
3. Kubota M, Nakano H, Sanjo I, Satoh K, Sanjo T, Kamegai T, et al. Maxillofacial morphology and masseter muscle thickness in adults. Eur J Orthod. 1998; 20:535–542.


4. Eröz UB, Ceylan I, Aydemir S. An investigation of mandibular morphology in subjects with different vertical facial growth patterns. Aust Orthod J. 2000; 16:16–22.
5. Ceylan I, Eröz UB. The effects of overbite on the maxillary and mandibular morphology. Angle Orthod. 2001; 71:110–115.
6. Beckmann SH, Kuitert RB, Prahl-Andersen B, Segner D, The RP, Tuinzing DB. Alveolar and skeletal dimensions associated with overbite. Am J Orthod Dentofacial Orthop. 1998; 113:443–452.
7. Nojima K, Nakakawaji K, Sakamoto T, Isshiki Y. Relationships between mandibular symphysis morphology and lower incisor inclination in skeletal class III malocclusion requiring orthognathic surgery. Bull Tokyo Dent Coll. 1998; 39:175–181.
8. Shimomoto Y, Chung CJ, Iwasaki-Hayashi Y, Muramoto T, Soma K. Effects of occlusal stimuli on alveolar/jaw bone formation. J Dent Res. 2007; 86:47–51.


9. Garn SM, Lewis AB, Vicinus JH. The inheritance of symphyseal size during growth. Angle Orthod. 1963; 33:222–231.
10. Diedrich P. [Problems and risks in the movement of the mandibular anterior teeth]. Fortschr Kieferorthop. 1995; 56:148–156. German.
11. Handelman CS. The anterior alveolus: its importance in limiting orthodontic treatment and its influence on the occurrence of iatrogenic sequelae. Angle Orthod. 1996; 66:95–109. discussion 109-10.
12. Aki T, Nanda RS, Currier GF, Nanda SK. Assessment of symphysis morphology as a predictor of the direction of mandibular growth. Am J Orthod Dentofacial Orthop. 1994; 106:60–69.


15. Esenlik E, Sabuncuoglu FA. Alveolar and symphysis regions of patients with skeletal class II division 1 anomalies with different vertical growth patterns. Eur J Dent. 2012; 6:123–132.


16. Wang MF, Otsuka T, Akimoto S, Sato S. Vertical facial height and its correlation with facial width and depth: three dimensional cone beam computed tomography evaluation based on dry skulls. Int J Stomatol Occlusion Med. 2013; 6:120–129.
17. Isaacson JR, Isaacson RJ, Speidel TM, Worms FW. Extreme variation in vertical facial growth and associated variation in skeletal and dental relations. Angle Orthod. 1971; 41:219–229.
18. Molina-Berlanga N, Llopis-Perez J, Flores-Mir C, Puigdollers A. Lower incisor dentoalveolar compensation and symphysis dimensions among Class I and III malocclusion patients with different facial vertical skeletal patterns. Angle Orthod. 2013; 83:948–955.

