INTRODUCTION
The 2012 International Society of Urological Pathology (ISUP) consensus conference on renal neoplasia adopted the new terminology of multilocular cystic renal neoplasm of low malignant potential (MCRNLMP) owing to its similar genetic and histopathological characteristics to clear cell renal cell carcinoma (CRCC), but completely different prognostic feature of a benign nature without recurrence or progression [
12]. However, the main method for differential diagnosis is based on histopathological confirmation after radical tumor resection from the kidney using standard hematoxylin and eosin-based histologic evaluation, and there are no clear immunohistochemical markers [
1].
The main disease of consideration in the differential diagnosis of MCRNLMP is clear cell RCC with cystic change (MCRCC), which is characterized by clear tumor cells composed of numerous cystic tumors layers that are indistinguishable from grade-1 clear cell RCC but without formation of a malignant and expansive nodule [
12]. By contrast, MCRNLMP represents a tumor composed entirely of numerous cysts with the septa containing individual or groups of clear cells without expansile growth [
3].
Besides its rarity, with an overall prevalence of less than 2% among all forms of cystic RCC, and indolent nature without recurrence or metastasis, it has thus far remained a clinical challenge to pinpoint clear differential morphological and histological characteristics to distinguish MCRNLMP from MCRCC [
4]. Nevertheless, MCRNLMP is still considered a distinct subtype of RCC owing to its similar pathological and genetic features to other clear cell RCCs [
3]. Although the chromosome 3p deletion and mutation of the
VHL gene have been observed in approximately 74% and 25% of MCRNLMP cases, respectively [
56], no clear genetic characteristics that are specifically associated with this subtype have been identified to date.
Accordingly, we have been exploring the differential characteristics of MCRNLMP and MCRCC from the clinical, histopathological, and genetic spectra. We previously reported that TGase-2 and Ki-67 might serve as useful tissue immunohistochemistry or microarray markers to distinguish MCRNLMP from MCRCC [
3]. In the present study, we focused on the genetic spectrum to identify any genes with significantly different mutation profiles between resected tumor tissues of patients with MCRNLMP and MCRCC. To this end, we used a target gene sequencing approach of 88 custom panel genes selected from the kidney cancer gene panel of the National Cancer Center of Korea that are known to be involved in the pathogenesis and progression of RCC.
RESULTS
The clinicopathological characteristics of the 30 patients included in the study are summarized in
Table 1, as reported previously [
7]. No baseline characteristics were significantly different (p>0.05) between the two disease groups. After quality control of the 30 tissue specimens for targeted sequencing of the 88 custom panel genes, only 16 MCRCC and 5 MCRNLMP tissues were ultimately selected for further analysis.
Table 2 shows the comparative sequencing results of 88 potentially pathogenic genes between the MCRCC and MCRNLMP samples. Ultimately, six target genes emerged as showing significantly different mutation profiles between the two diseases:
GIGYF2,
FGFR3,
SETD2,
BCR,
KMT2C, and
TSC2 (
Table 2).
Table 2
Significant differential genes from the kidney cancer panel between 16 MCRCC and 5 MCRNLMP tissue samples
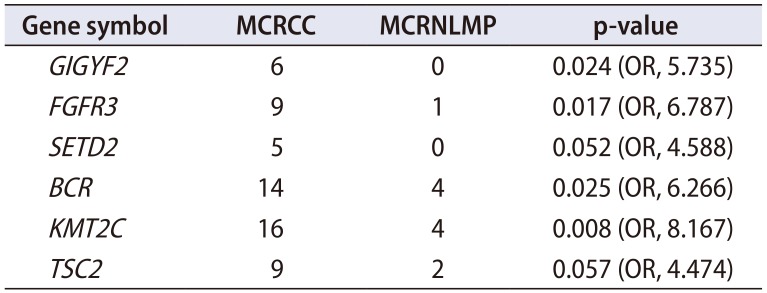
Gene symbol |
MCRCC |
MCRNLMP |
p-value |
GIGYF2
|
6 |
0 |
0.024 (OR, 5.735) |
FGFR3
|
9 |
1 |
0.017 (OR, 6.787) |
SETD2
|
5 |
0 |
0.052 (OR, 4.588) |
BCR
|
14 |
4 |
0.025 (OR, 6.266) |
KMT2C
|
16 |
4 |
0.008 (OR, 8.167) |
TSC2
|
9 |
2 |
0.057 (OR, 4.474) |

In addition, 36 of the 88 genes were not detected to be positive in any sample in both groups; BAP1, TCEB1, and TFEB appeared in one of the MCRNLMP cases; and the following 43 genes were only detected in MCRCC: AGTR1, MET, MTOR, PDGFRB, SETD2, FGFR4, FH, MYH11, SGOL2, SMAD4, ZNF765, AKT1, EGFR, FLCN, KIT, USP24, WWP2, PDGFRA, PNKD, RB1, AP5M1, AXL, BRAF, FLT3, KRAS, MLH1, PDXDC1, SDHD, SMARCA4, SMARCB1, STAG2, and TFE3. The three genes only detected in MCRNLMP and 46 genes only detected in MCRCC were only detected in some of the cases in each disease group, and no statistical significance was reached to indicate their differential mutation patterns (p>0.05).
Fig. 1 provides an overall comparative view of the prevalence status of the 88 candidate genes in the two diseases in view of the different types of mutations detected (e.g., insertion, deletion, point mutation, mis/non-sense mutation, splicing mutation, multi-hit point mutation).
DISCUSSION
MCRNLMP and MCRCC have several similarities, allowing for classification of MCRNLMP as a subtype of RCC despite its lack of progression and favorable prognosis [
21]; indeed, it has been suggested that MCRNLMP may represent the earliest stage of clear cell RCC. Histopathologically, MCRNLMP shows a clear cell type lining, and approximately 25% of cases show a
VHL mutation and 74% of cases are associated with a chromosome 3p deletion [
56]. Less than 10% of patients have concurrent MCRNLMP and MCRCC in the same ipsilateral kidney, indicating that a trigger point might exist to induce the malignant transformation from MCRNLMP originating from the same event involving similar developmental processes owing to a mutation of the
VHL gene [
22].
Similar to this study, Raspollini et al. [
23] conducted a genetic mutational analysis between clear cell RCC and MCRNLMP and found no significant genetic differences between the groups, except that a
KRAS mutation could distinguish between the two subtypes in spite of their histologic similarities. In the present study,
KRAS mutation was not detected in any of the five MCRNLMP cases but was detected in one MCRCC case, which might support these previous results. Importantly, we identified six novel genetic alterations (
GIGYF2,
FGFR3,
SETD2,
BCR,
KMT2C, and
TSC2) that could be potential candidate genes for differentiating between MCRNLMP and MCRCC.
Although we were not able to compare the complete gene profiles owing to a lack of statistical power because of the small sample size, interpretation of each individual gene and its suggestion of a genetic role can offer a preliminary start toward gaining an understanding of the differential pathogenesis of MCRNLMP from MCRCC for elucidating the mechanism contributing to the aggressive nature of RCC. Besides the six genes mentioned above, the 36 genes that were expressed in all cases in one group or in no cases in the other group might also be relevant to the pathogenesis of cystic RCC, particularly with regard to considering MCRNLMP as the earliest stage of RCC with same developmental route.
SET domain-containing 2 (
SETD2) is chromatin-remodeling tumor suppressor gene that is frequently found mutated in clear cell RCC [
2124], and alterations of this gene were significantly more frequent in MCRCC than in MCRNLMP in the present study.
SETD2 is located in chromosome 3p21, along with other genes involved in chromatin remodeling such as polybromo 1 (
PBRM1) and BRCA-1-associated protein-1 (
BAP1), which have all been shown to influence tumor biology and predict survival [
24]. Inactivation of the
SET2D gene enables renal primary tubular epithelial cells to bypass the senescence barrier, facilitating a malignant transformation toward clear cell RCC. Therefore, SET2D might be the most important key gene for transforming benign MCRNLMP to malignant MCRCC given that
SET2D expression was not detected in any of the five MCRNLMP samples.
The lysine methyltransferase 2C (
KMT2C; odds ratio [OR], 8.167), also known as MLL3/KMT2C in humans is associated as a tumor suppressor with leukemia and other solid tumors and encodes a nuclear protein with multiple domains, including a SET domain and post-SET domain. It possesses histone methylation activity for transcriptional coactivation [
321]. A recent mutation hotspot analysis showed that
KMT2C was one of the stronger mutation hotspots with a higher mutation allele frequency than non-hotspots, which can help to prioritize cancer drivers as potential targets [
23]. The knockdown model of MLL3/KMT2C produced aggressive features of acute myeloid lymphoma in mouse models, and the knockdown of MLL3 protein in normal hematopoiesis results in impaired differentiation and increased hematopoietic stem/multipotent progenitor cell numbers relating to increased oxidative stress [
25]. This study also showed that all cases of MCRCC had alterations of
KMT2C, and most cases of MCRNLMP were also positive for this gene. A study of unclassified RCC samples showed a frequency of 10%
KMT2C mutation, indicating its role as an epigenetic regulator in RCC [
26].
Tuberous sclerosis complex 2 (
TSC2; OR, 4.474) is a well-known tumor suppressor gene along with
TSC1 and shows a clear association with autosomal dominant hereditary kidney cancer of the tuberous sclerosis complex, as well as angiomyolipoma, clear cell RCC, and other types of tumors [
27]. The
TSC2 gene encodes tuberin protein, which is an essential part of the LKB1/AMPK/TSC/mTOR nutrient and energy sensing pathway [
27]. The levels of active TSC protein complex directly control the activity of the mTOR pathway, which is itself controlled by AMPK and AKT in opposing manners.
TSC2 loss has been shown to result in the accumulation of HIF1α and increased expression of HIF-responsive genes, including vascular endothelial growth factor (VEGF). We also found a greater number of MCRCC tissues with a missense mutation in
TSC2, and a previous study demonstrated increased expression of VEGF1-3 tissue markers using immunohistochemistry [
7].
The BCR, RhoGEF, and GTPase activating protein (
BCR; OR, 6.266) gene does not have a clearly identified function in cancer, including RCC. However,
BCR associated with
ABL as a fusion gene is known as a driver oncogene in chronic myeloid leukemia and acute lymphoblastic leukemia and is closely connected with the immune system [
28]. ABL1 kinase inhibitors (for example, imatinib) have been introduced to markedly improve the survival of leukemia patients with the
ABL-BCR combination in spite of acquired drug resistance via the BCR-ABL1 kinase domain gatekeeper mutation Thr315Ile (T315I). Axitinib is a well-known multi-targeting agent in metastatic RCC with a good therapeutic prognosis and potently inhibits BCR-ABL1(T315I) at both the biochemical and cellular levels by binding to the active form of ABL1(T315I) in a mutation-selective binding mode.
The GRB10 interacting GYF protein 2 (
GIGYF2; OR, 5.735) gene is not well known to play a role in RCC but is well known to be associated with breast cancer and may be involved in the regulation of tyrosine kinase receptor signaling, especially for Akt activity regulation in the EGFR-signaling pathway, including Akt, EGFR, GIGYF1, GIGYF2, and GRB10 [
29]. The present study also showed that
EGFR was mutated in four MCRCC cases and
GIGYF2 was mutated in six cases, whereas none of the MCRNLMP cases was positive for
EGFR or
GIGYF2 mutation (p=0.113). Therefore, further studies should investigate the clinical implication of
GIGYF2 in combination with
EGFR.
A recent study investigated the point mutations in the fibroblast growth factor receptor 3 (
FGFR3) gene in RCC, and no mutations were detected in any renal tumor type examined, with all cases showing the wild-type sequence [
30]. However, another recent study described a patient with cystic renal dysplasia (thanatophoric dysplasia type 1) due to a germline
FGFR3 mutation, indicating potential involvement of mutational
FGFR3 activation in renal cyst formation [
2]. Indeed, the proximal tubule expresses
FGFR3 but not
FGFR1,
FGFR2, or
FGFR4 [
10], and several studies also showed that FGFs and FGFRs are expressed in the developing rat metanephros and thus likely play important roles in metanephric development and maturation [
14]. In the present study,
FGFR3 gene alteration was more highly involved in MCRCC than in MCRNLMP.
One of the main limitations of this study was the small number of samples so that the statistical power was low for detecting the association of the majority of the target genes in spite of their potential differentiation between the two diseases. Another limitation was that many of the MCRNLMP tissues failed to pass their quality control test because of the small proportion of presenting tumor cells in each case. Such cases tended to have single-layered and scant clear cells lining the multiseptated renal cysts. However, the most important limitation was the interpretation and statistical comparison of the 88 gene sequencing results. The interpretation of the results was based only on the significant differences in genetic alterations between the two disease groups, regardless of a single gene sequence interpretation, which should be further analyzed in future studies. Finally, more comprehensive and multifactorial interpretational approaches in consideration of clinicopathological and immunohistochemical findings should be further considered in future investigations, and multi-combined gene linkage analyses should be conducted given that many diverse cancer pathways are collectively involved in RCC.
Despite these limitations, this comparative gene study provided preliminary information on the differential genetic pathogenesis of these two similar diseases. Further studies with larger numbers of patients are needed to validate the six significant genes found in this study, providing more clinically significant information for a better understanding of the pathogenetic changes from benign potential to invasive malignancy in RCC.