Abstract
PURPOSE
Limited data is available regarding the differences for possible microleakage problems and fitting accuracy of zirconia versus titanium abutments with various connection designs. The purpose of this in vitro study was to investigate the effect of connection design and abutment material on the sealing capability and fitting accuracy of abutments.
MATERIALS AND METHODS
A total of 42 abutments with different connection designs [internal conical (IC), internal tri-channel (IT), and external hexagonal (EH)] and abutment materials [titanium (Ti) and zirconia (Zr)] were evaluated. The inner parts of implants were inoculated with 0.7 µL of polymicrobial culture (P. gingivalis, T. forsythia, T. denticola and F. nucleatum) and connected with their respective abutments under sterile conditions. The penetration of bacteria into the surrounding media was assessed by the visual evaluation of turbidity at each time point and the number of colony forming units (CFUs) was counted. The marginal gap at the implant- abutment interface (IAI) was measured by scanning electron microscope. The data sets were statistically analyzed using Kruskal-Wallis followed by Mann-Whitney U tests with the Bonferroni-Holm correction (α=.05).
RESULTS
Statistically significant difference was found among the groups based on the results of leaked colonies (P<.05). The EH-Ti group characterized by an external hexagonal connection were less resistant to bacterial leakage than the groups EH-Zr, IT-Zr, IT-Ti, IC-Zr, and IC-Ti (P<.05). The marginal misfit (in µm) of the groups were in the range of 2.7–4.0 (IC-Zr), 1.8–5.3 (IC-Ti), 6.5–17.1 (IT-Zr), 5.4–12.0 (IT-Ti), 16.8–22.7 (EH-Zr), and 10.3–15.4 (EH-Ti).
Dental implant rehabilitation has been widely used in clinical practice to replace missing teeth with high success and survival rates.1234 An ideal relationship between an implant and the periimplant tissues is necessary to achieve functional and aesthetic restorations that can be accomplished with a careful balance of several parameters.25 Establishment of stable hard and soft tissues around the implant is related to some biological, technical, and prosthetic considerations such as the implant surface, surgical technique, and prosthetic design.145
Most dental implant systems are composed of two main components: the endosteal part (implant) and the transmucosal connection (abutment).3 The implant body and abutment connection could be designed as one-piece or two-piece.1 In one-piece dental implants, the standard abutment is fabricated with implant body, whereas two-piece dental implants have a standard or custom-made abutment fabricated separately.3 Two-piece dental implants are preferred due to the availability of custom-made or angled abutment options. One-piece implants are mainly indicated for specific cases with small diameters.2 Two-piece dental implants present microgaps at the implant-abutment interface (IAI) due to the precision limits of production stages.35 The microgap at the IAI is reported to limit the success of implant rehabilitation.167 The microgaps bare the potential for accumulation of bacteria and bacterial byproducts which may lead to periimplant inflammation and bone loss, eventually.8 Therefore, achieving perfect seal and accurate fit at the IAI is essential for long-term success of dental implants.
Since the introduction of dental implants, manufacturers have attempted to perfect the design of implant-abutment connection by developing different connection types. They can be generally classified into external and internal connections.123 Both types may be further subclassified according to the antirotational geometry of the contact area between implant and abutment.3 Some of these various antirotational features include hexagonal, octagonal, conical, tri-channel, spline, cam tube and the morse taper connection designs.236 A number of factors have been reported to affect the microgap and bacterial leakage at the IAI. Some of the factors include the antirotational geometry of connection, force used to tighten the abutment, and material used.56789 Dental implants and abutments are generally made of commercially pure titanium due to the high physical properties and biocompatibility of the material.23 However, titanium abutments might display an unnatural grayish discoloration through thin soft tissues, compromising optimal mucogingival esthetics.57 Alternative abutment materials have been sought to overcome such disadvantages. Zirconia abutments are frequently preferred for their esthetic properties and biocompatibility.9 Abutments with different connection designs or material types are placed on the implants with a torque value recommended by the manufacturer to ensure accurate fit and minimize microleakage at the IAI.37
Currently, there is very limited data comparing possible microleakage and marginal fit of zirconia versus titanium abutments with different connection designs. Thus, the purpose of this in vitro study was to investigate the effects of various connection designs and type of abutment material on the sealing capability and marginal fit of abutments. The null hypothesis was that the material type or connection design would not affect the microleakage and marginal fit at the IAI.
Three groups of implants with different IAI geometries [internal conical (IC), internal tri-channel (IT), and external hexagonal (EH)] and abutment materials [titanium (Ti) and zirconia (Zr)] were used in the present study (Table 1). A total of 42 sterile implants [14 with an internal conical connection (Nobel Parallel CC RP 4.3 × 10 mm, Nobel Biocare, Göteborg, Sweden), 14 with a tri-channel connection (Replace Select Straight TiU RP 4.3 × 10 mm, Nobel Biocare, Göteborg, Sweden) and 14 with an external hexagon (NOBELSPEEDY Groovy RP 4.0 × 10 mm, Nobel Biocare, Göteborg, Sweden)] were divided into 2 subgroups (n = 7) according to the abutment material used (Table 2) (Fig. 1).
A polymicrobial culture was prepared with the main bacterial strains involved in periimplantitis and alveolar bone resorption: P. gingivalis (ATCC 33277), T. forsythia (ATCC 43037), T. denticola (ATCC 35404) and F. nucleatum (ATCC 10935). P. gingivalis was grown in tryptic soy broth supplemented with hemin, yeast extract, and vitamin K. T. forsythia was grown in liquid tryptic soy agar broth supplemented with yeast extract, sheep blood, and N-acetylmuramic acid (NAM). T. denticola was grown in GM-1 serum-containing broth and F. nucleatum was grown on blood agar plates. All bacteria were grown under anaerobic conditions (85% N2-10% H2-5% CO2) in an anaerobic chamber at 37℃. A Petroff-Hausser counting chamber was used to determine the amount of each bacterial strain. The culture purities were determined by plating the diluted bacteria on blood agar for P. gingivalis, by culturing on NAM plates for T. forsythia, by examining with phase-contrast microscopy for T. denticola, and by Gram staining for F. nucleatum. A polymicrobial culture was prepared by mixing equal volumes [1 mL (108 cells per mL)] of each bacterial strain (P. Gingivalis, T. denticola, T. forsythia and F. nucleatum, respectively) in Todd-Hewitt broth (Sigma-Aldrich; Merck KGaA, Darmstadt, Germany) supplemented with hemin (10 mL/L) and menadione (2 mL/L) under anaerobic conditions. The growth phase of the bacterial culture, the counts and viability of each bacterial strain were all standardized. The inner parts of the implants kept in an upright position were inoculated with 0.7 µL of the polymicrobial culture (2.5 × 105 cells of each bacterium per mL) by a calibrated micropipette (Pipetman Ultra, Gilson, Middleton, WI, USA) in a sterile hood. Then, the abutments were screwed onto the implants with a torque of 35 Ncm according to the manufacturer's instructions (torque wrench, Nobel Biocare, Göteborg, Sweden) without contaminating the external surfaces of implant-abutment assemblies. Each sample was then immersed into the test tubes containing 1 mL of Todd-Hewitt broth supplemented with hemin and menadione, which was sufficient enough to cover the IAI while preventing leakage through the screw access channel. One negative control test tube was assessed for each subgroup (n = 6) with submerging the implant-abutment assembly into the same broth without bacterial inoculation. Additionally, one positive control for each subgroup with bacterial inoculation was prepared with abutments tightened with minimal torque (10 Ncm) and immersed into the same nutritious conditions to confirm the viability of bacteria throughout the experiment. The penetration of bacteria into the surrounding media was assessed by the visual evaluation of turbidity at each time point for up to 7 days. After the observation of turbidity, 60 µL of Todd-Hewitt broth was removed from each test tube and spread-plated on blood agar or evaluated by phase-contrast microscopy and Gram staining for quantification of colony-forming units (CFUs).
After the microbiological tests, implant-abutment assemblies were cleaned with phosphate buffered saline (PBS) and evaluated by scanning electron microscopy (SEM) (EVO LS 10, Zeiss, Oberkochen, Germany) for marginal fit at the IAI. Each implant-abutment assembly was placed on a custom-made specimen holder to ensure accurate positioning for SEM images. Four aspects (mesial, distal, buccal, and palatal) of each sample along the IAI was imaged at a magnification of × 2000. The arithmetic means of 85 measuring points of each aspect were recorded with the aid of an analyzer function of SEM apparatus.
Data were analyzed using statistical software (IBM SPSS Version 20, IBM, New York, NY, USA). The normality of data was tested using Shapiro-Wilk test, and nonparametric multivariate Kruskal-Wallis was used to test the omnibus hypothesis. The Kruskal-Wallis test followed by Mann-Whitney U tests with the Bonferroni-Holm correction was used to analyze differences among the groups. The level of significance was set at P < .05.
The multivariate Kruskal-Wallis test revealed significant differences among the groups (IC-Ti, IC-Zr, IT-Ti, IT-Zr, EH-Ti, and EH-Zr) for the tested parameters (bacterial leakage and marginal fit) (P < .05).
The results of bacterial leakage are presented in Table 3. Statistically significant difference was found among the groups based on the results of leaked colonies (P < .05) (Table 3). The implants in group EH-Ti characterized by an external hexagonal connection were less resistant to bacterial leakage than the other tested groups (EH-Zr, IT-Zr, IT-Ti, IC-Zr, and IC-Ti). No significant difference was found among the groups EH-Zr, IT-Zr, IT-Ti, and IC-Ti for the leaked colonies (P > .05). The lowest bacterial leakage was found in IC-Zr group characterized by an internal conical connection and Zr abutment.
The results of marginal misfit at the IAI are presented in Table 4. The representative SEM images of the IAI of each group (EH-Zr, IT-Zr, IT-Ti, IC-Zr, and IC-Ti) used for marginal misfit measurements are shown in Figure 2. The marginal misfit values (in µm) of the groups were in the range of 2.7–4.0 (IC-Zr), 1.8–5.3 (IC-Ti), 6.5–17.1 (IT-Zr), 5.4–12.0 (IT-Ti), 16.8–22.7 (EH-Zr) and 10.3–15.4 (EH-Ti). The highest mean marginal misfit was obtained in EH-Zr group, which was significantly higher than IC-Zr, IC-Ti, and IT-Ti (P < .05) (Table 4). The IC-Ti group revealed the lowest marginal misfit, which was significantly lower than IT-Zr, IT-Ti, EH-Zr, and EH-Ti (P < .05).
The null hypothesis was rejected as significant differences were found among the groups based on the results of polymicrobial sealing capability and marginal fit of tested abutments.
Inflammation of the periimplant tissues is considered as one of the primary causes leading to implant failures.678 The long-term success of dental implants is affected by several factors related to the implant-abutment connection including microbiological, mechanical, and technical aspects.245 In the two-piece dental implants, the internal cavity of implant and the microgap at the IAI may act as a reservoir for microorganisms.78 Thus, the microbial leakage through the microgap between implant and abutment can cause inflammation at the periimplant tissues, bone loss, and eventually implant failures.6789 Assessment of the sealing capability of implant systems with different connection designs and abutment materials is of primary importance for proper abutment selection in clinical practice.7810 Up to now, the leakage at the IAI was evaluated by several techniques including microorganisms, color tracing, bacterial byproducts, or gas infiltration method.101112 In order to maintain clinical relevance, periimplantitis associated anaerobic bacteria, which is known as ‘red complex’, were used in the present study. However, the level of leakage could be affected by the methodology and the volume of bacterial concentration used to inoculate the inner parts of implants.1012 In the present study, a volume of 0.7 µL bacterial suspension was used to inoculate the implants, which is determined after several laboratory trials to be sufficient to avoid false positive or false negative results. Different microbiological cultures with varying inoculation volumes were used for microleakage tests in previous studies that reported bacterial leakage at the IAI, similar to the present study.121314 However, the polymicrobial combination used in the present study has not been used in any other study. In order to increase clinical relevance, a polymicrobial culture responsible for periimplant diseases that is consisted of P. gingivalis, T. forsythia, T. denticola, and F. nucleatum were prepared. Stastically significant differences were found among the groups based on the results of leaked CFUs. The highest mean value was obtained in the EH-Ti group followed by the groups EH-Zr, IT-Zr, IC-Ti, IT-Ti, and IC-Zr. The Zr abutments with an internal conical (IC-Zr) connection were more resistant to bacterial leakage than the Ti abutments with internal conical connection and Ti or Zr abutments with external hexagonal or tri-channel connection. In accordance with the present study, the conical connections had been reported to be more resistant to bacterial leakage than the other connection designs.1013 Nevertheless, increased leakage for Zr abutments compared to Ti abutments was reported, possibly due to the lower recommended torque values used to tighten the Zr abutments.15 In the present study, the same torque value of 35 Ncm was used to tighten all the abutments following the manufacturer's instructions. Zr abutments in the IC and EH groups were more resistant to bacterial leakage than the Ti abutments in the same groups. However, greater bacterial adhesion to titanium surfaces when compared to zirconia had been reported by Nascimento et al.16 Different findings among the studies could be explained with the differences in the methodology such as bacteria used, sampling techniques, closing screw torque values, and characteristics of implant systems. Additionally, no significant difference was observed between Zr and Ti abutments in the IT group. This finding of our study could be attributed to the presence of a secondary metallic insert. The IT-Zr abutment has a titanium insert that attaches the implant body and creates a titanium-titanium interface rather than a zirconia-titanium interface, which might have an impact on the leakage values obtained.
The inevitable microgap at the IAI in two-piece implants is considered to be responsible for some biological and mechanical problems such as bacterial leakage and screw loosening.67 Several modifications to the design of implant systems were made to minimize the gap at the IAI, including location of the IAI, connection geometry, taper degree, abutment screw shape, length, and recommended screw torque value.1234 The size of the microgap at the IAI could be investigated by different techniques such as SEM, micro-CT, and synchrotron-based radiography.717 The observation technique used to detect the microgap was SEM in the present study. The observational technique and the reference points determined to measure the microgap were reported to be effective on the results obtained.161718 A gap size ranging from 0.1 µm to 50 µm at the IAI was reported in different studies.716171819 Statistically significant differences were found among the groups concerning the results of marginal fit in the present study. The lowest mean microgap was 1.8 ± 0.3 µm observed in IC-Ti group and the highest mean microgap was 22.7 ± 9.1 µm observed in EH-Zr group. The obtained mean microgap values in IC-Zr, IC-Ti, IT-Zr, and IT-Ti groups were in line with the results of some previous studies.1617 There are also studies reporting higher or lower microgap values for the same abutments in previous studies. Baixe et al.20 reported the size of the microgap in the range of 0.25 to 18.93 µm for Zr Nobel Replace abutments with tri-channel connection. In another study by Hamilton et al.,21 the mean microgap size was reported to be 47.7 µm for Ti Nobel Replace abutments with tri-channel connection and 22.3 µm for Ti Nobel Bränemark System abutments with external hexagonal connection. The differences among the studies could be explained with different technical conditions such as reference points, sample size, and deformation due to the cross-sectioning.1617181920
Further in vitro and in vivo studies are necessary to determine the effects of connection design and abutment material on the microleakage and marginal fit by simulating the loading conditions of intraoral environment and different closing screw torque values.
Within the limitations of the present study, it may be concluded that the connection design and type of abutment material affect the microbiological sealing capability and marginal fit of abutments. The zirconia abutments with internal conical connection seem to be more resistant to bacterial leakage.
References
1. Gracis S, Michalakis K, Vigolo P, Vult von Steyern P, Zwahlen M, Sailer I. Internal vs external connections for abutments/reconstructions: a systematic review. Clin Oral Implants Res. 2012; 23:202–216. PMID: 23062143.


2. Shim HW, Yang BE. Long-term cumulative survival and mechanical complications of single-tooth Ankylos Implants: focus on the abutment neck fractures. J Adv Prosthodont. 2015; 7:423–430. PMID: 26813443.


3. Binon PP. Implants and components: entering the new millennium. Int J Oral Maxillofac Implants. 2000; 15:76–94. PMID: 10697942.
4. Koutouzis T, Neiva R, Nonhoff J, Lundgren T. Placement of implants with platform-switched Morse taper connections with the implant-abutment interface at different levels in relation to the alveolar crest: a short-term (1-year) randomized prospective controlled clinical trial. Int J Oral Maxillofac Implants. 2013; 28:1553–1563. PMID: 24278924.


5. Siadat H, Beyabanaki E, Mousavi N, Alikhasi M. Comparison of fit accuracy and torque maintenance of zirconia and titanium abutments for internal tri-channel and external-hex implant connections. J Adv Prosthodont. 2017; 9:271–277. PMID: 28874994.


6. Moon SJ, Kim HJ, Son MK, Chung CH. Sinking and fit of abutment of locking taper implant system. J Adv Prosthodont. 2009; 1:97–101. PMID: 21165262.


7. Lee JH, Kim DG, Park CJ, Cho LR. Axial displacements in external and internal implant-abutment connection. Clin Oral Implants Res. 2014; 25:e83–e89. PMID: 23088616.


8. Goodacre CJ, Kan JY, Rungcharassaeng K. Clinical complications of osseointegrated implants. J Prosthet Dent. 1999; 81:537–552. PMID: 10220658.


9. Nakamura K, Kanno T, Milleding P, Ortengren U. Zirconia as a dental implant abutment material: a systematic review. Int J Prosthodont. 2010; 23:299–309. PMID: 20617217.
10. Teixeira W, Ribeiro RF, Sato S, Pedrazzi V. Microleakage into and from two-stage implants: an in vitro comparative study. Int J Oral Maxillofac Implants. 2011; 26:56–62. PMID: 21365038.
11. Mishra SK, Chowdhary R, Kumari S. Microleakage at the different implant abutment interface: A systematic review. J Clin Diagn Res. 2017; 11:ZE10–ZE15. PMID: 28764310.


12. Al-Jadaa A, Attin T, Peltomäki T, Schmidlin PR. Comparison of three in vitro implant leakage testing methods. Clin Oral Implants Res. 2015; 26:e1–e7.
13. Harder S, Dimaczek B, Açil Y, Terheyden H, Freitag-Wolf S, Kern M. Molecular leakage at implant-abutment connection--in vitro investigation of tightness of internal conical implant-abutment connections against endotoxin penetration. Clin Oral Investig. 2010; 14:427–432.


14. Nascimento C, Ikeda LN, Pita MS, Pedroso e Silva RC, Pedrazzi V, Albuquerque RF, Ribeiro RF. Marginal fit and microbial leakage along the implant-abutment interface of fixed partial prostheses: An in vitro analysis using Checkerboard DNA-DNA hybridization. J Prosthet Dent. 2015; 114:831–838. PMID: 26359546.
15. Abdelhamed MI, Galley JD, Bailey MT, Johnston WM, Holloway J, McGlumphy E, Leblebicioglu B. A Comparison of Zirconia and Titanium Abutments for Microleakage. Clin Implant Dent Relat Res. 2015; 17:e643–e651. PMID: 25726941.


16. Nascimento CD, Pita MS, Fernandes FHNC, Pedrazzi V, de Albuquerque RF Junior, Ribeiro RF. Bacterial adhesion on the titanium and zirconia abutment surfaces. Clin Oral Implants Res. 2014; 25:337–343. PMID: 23316996.


17. Nascimento CD, Pita MS, Fernandes FHNC, Pedrazzi V, de Albuquerque RF Junior, Ribeiro RF. Bacterial adhesion on the titanium and zirconia abutment surfaces. Clin Oral Implants Res. 2014; 25:337–343. PMID: 23316996.


18. Smith NA, Turkyilmaz I. Evaluation of the sealing capability of implants to titanium and zirconia abutments against Porphyromonas gingivalis, Prevotella intermedia, and Fusobacterium nucleatum under different screw torque values. J Prosthet Dent. 2014; 112:561–567. PMID: 24656409.


19. Black DL, Turkyilmaz I, Lien W, Chong CH. Evaluation of the sealing capability of the internal conical connections of implants with titanium and zirconia abutments. J Contemp Dent Pract. 2017; 18:915–922. PMID: 28989130.
20. Baixe S, Fauxpoint G, Arntz Y, Etienne O. Microgap between zirconia abutments and titanium implants. Int J Oral Maxillofac Implants. 2010; 25:455–460. PMID: 20556243.
21. Hamilton A, Judge RB, Palamara JE, Evans C. Evaluation of the fit of CAD/CAM abutments. Int J Prosthodont. 2013; 26:370–380. PMID: 23837169.


Fig. 2
Scanning electron micrographs of marginal misfit at implant-abutment interface (original magnification × 2000).
(A) IC-Ti; (B) IC-Zr; (C) IT-Ti; (D) IT-Zr; (E) EH-Ti; (F) EH-Zr and (original magnification × 100).
(a) IC-Ti; (b) IC-Zr; (c) IT-Ti; (d) IT-Zr; (e) EH-Ti, and (f) EH-Zr.
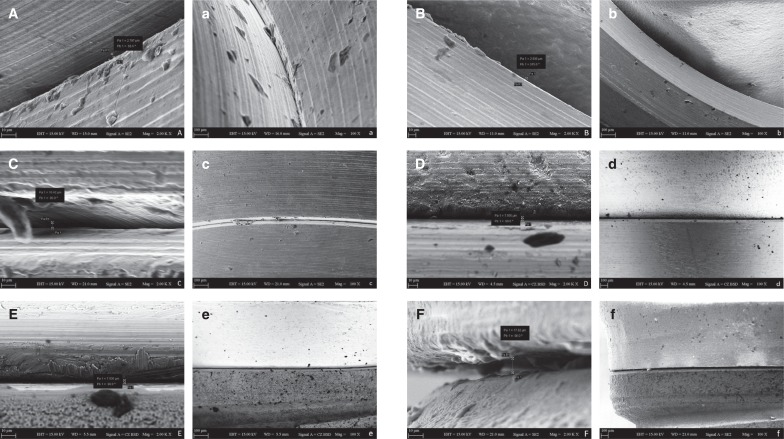
Table 1
Characteristics of the implants and abutments used
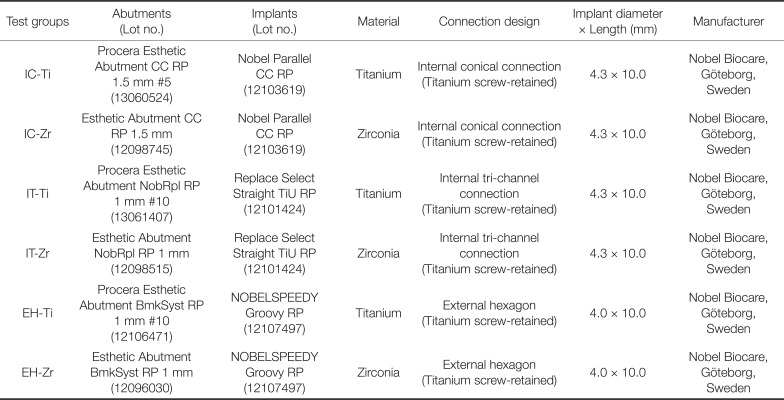
Table 3
Mean ± SD values of leaked colonies
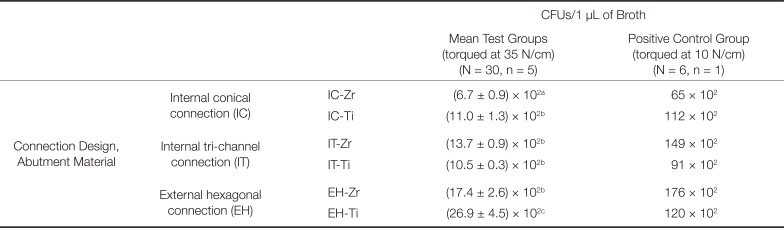
Table 4
Mean ± SD values of marginal misfit at the IAI
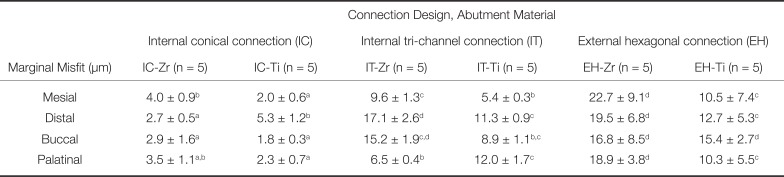