Abstract
Pituitary tumors are usually benign but can occasionally exhibit hormonal and proliferative behaviors. Dysregulation of the G1/S restriction point largely contributes to the over-proliferation of pituitary tumor cells. F-box protein S-phase kinase-interacting protein-2 (SKP2) reportedly targets and inhibits the expression of p27Kip1, a well-known negative regulator of G1 cell cycle progression. In this study, SKP2 expression was found to be upregulated while p27Kip1 expression was determined to be downregulated in rat and human pituitary tumor cells. Furthermore, SKP2 knockdown induced upregulation of p27Kip1 and cell growth inhibition in rat and human pituitary tumor cells, while SKP2overexpression elicited opposite effects on p27Kip1 expression and cell growth. The expression of microRNA-186 (miR-186) was reported to be reduced in pituitary tumors. Online tools predicted SKP2 to be a direct downstream target of miR-186, which was further confirmed by luciferase reporter gene assays. Moreover, miR-186 could modulate the cell proliferation and p27Kip1-mediated cell cycle alternation of rat and human pituitary tumor cells through SKP2. As further confirmation of these findings, miR-186 and p27Kip1 expression were downregulated, while SKP2 expression was upregulated in human pituitary tumor tissue samples; thus, SKP2 expression negatively correlated with miR-186 and p27Kip1 expression. In contrast, miR-186 expression positively associated with p27Kip1 expression. Taken together, we discovered a novel mechanism by which miR-186/SKP2 axis modulates pituitary tumor cell proliferation through p27Kip1-mediated cell cycle alternation.
Pituitary tumors are usually benign neoplasms accounting for 10% to 15% of intracranial tumors [1]. Pituitary tumors occur generally in the sella turcica, and may occasionally be ectopic [2], exhibiting a wide range of hormonal and proliferative behavior [3]. Increased knowledge of the mechanisms that regulate pituitary tumor cell proliferation may be of great clinical value.
The cell cycle is the process by which cells grow, replicate their genome and divide. The cell cycle is controlled by a cyclically-operating biochemical system constructed from a set of interacting proteins that induce and coordinate proper progression through the cycle. Loss and/or deregulation of the cell cycle at the G1/S restriction point is considered a critical issue among virtually all types of human tumors, including pituitary tumors [3]. Furthermore, overproliferation induced by cell cycle dysfunction is regarded as a crucial contributor to pituitary tumor development [4].
According to previous studies, the tumor is highly susceptible to genetic alterations of specific cell cycle regulators such as the retinoblastoma protein and the cell cycle inhibitory protein p27Kip1 [45]. The p27 functions as a negative regulator of G1 cell cycle progression, and is commonly underexpressed in many tumor types, including pituitary tumors [6789]. Regarding the molecular mechanism, F-box protein S-phase kinase-interacting protein-2 (SKP2), a known oncogene, can inhibit p27Kip1 expression by directly targeting it for ubiquitin-dependent degradation [101112]. In pituitary adenomas, the expression of SKP2 negatively correlates with that of p27Kip1 [13], suggesting a significant role for SKP2/p27Kip1 in pituitary tumor development.
During the past decades, several studies have shown that miRNAs are involved in diverse processes, including differentiation metabolism cell growth and apoptosis [14], and have been implicated in the pathogenesis of several human cancers, including breast cancer [15] and colon cancer [16], as well as in pituitary tumors [17]. The dysregulation of microRNAs (miRNAs) has been shown to impact pituitary cell proliferation and cell cycle progression [18192021]. A total of 12 miRNAs, including miR-125b, miR-141, miR-144, miR-164, miR-145, miR-143, miR-15b, miR-16, miR-186, let-7b, let-7a3, and miR-128, have been found to be underexpressed in pituitary tumors [4]. Of these 12 miRNAs, the role of miR-186 has not been well established in pituitary tumors; however, online tools such as miRwalk (University of Heidelberg, Heidelberg, Germany) [22], miRanda (Memorial Sloan-Kettering Cancer Center, Newyork, NY, USA) [23] and TargetScan (Whitehead Institute, Cambrige, MA, USA) [24] predict that SKP2 might serve as a direct downstream target of miR-186.
In the present study, the expression and function of SKP2 and p27Kip1 were evaluated in a rat pituitary tumor cell line and primary human growth hormone (GH)-secreting pituitary adenoma (PA) cells. Next, the direct binding and regulatory relationship between miR-186 and SKP2 were examined. After confirming that miR-186 directly targets SKP2, the combined effects of miR-186 and SKP2 on pituitary tumor cell cycle and proliferation were evaluated. Finally, the expression levels of and correlations among miR-186, SKP2, and p27Kip1 in human pituitary tumor tissue samples were determined to further confirm the findings in this study. In summary, we have discovered a novel mechanism of the miR-186/SKP2/p27Kip1 axis modulating pituitary tumor cell cycle and proliferation.
A total of 20 human pituitary tumor tissues were collected from patients who had undergone surgery. In additon, 5 normal pituitary tissues were obtained from autopsies in which the deceased had no history of any endocrine abnormalities. Tissues were collected under research protocol approved by Sichuan Provincial People's Hospital of University of Electronic of Science and Technology of China. Informed consent was obtained from all patients or their families. Tissue samples were fixed in 10% formalin or immediately frozen in liquid nitrogen and stored at −70℃ until further use.
The fixed tissue samples were embedded in paraffin and then cut into 4-µm-thick tissue sections. After removing the wax, the sections were incubated in 10% normal goat serum (Beyotime, Haimen, China) for 30 min at 37℃ and then with the anti-p27Kip1 antibody (ab32034; Abcam, Cambridge, MA, USA), overnight at 4℃. The sections were incubated with a secondary antibody linked with biotin and then with HRP-conjugated anti-biotin (Beyotime) for 30 min at 37℃. Subsequently, the sections were incubated in freshly prepared DAB reagent and then counterstained with hematoxylin (Beyotime). The sections were visualized by light microscopy (Olympus, Tokyo, Japan).
The rat pituitary tumor cell line GH3 and the human embryonic kidney epithelial cell line HEK293 were obtained from ATCC (American Type Culture Collection, Manassas, VA, USA). HEK293 cells were cultured in Eagle's minimum essential medium (MEM) supplemented with 10% fetal bovine serum (FBS). GH3 cells were cultured in Ham's F12K medium enriched with 15% horse serum and 2.5% FBS, as previously described [25]. All cells were cultured at 37℃ with 5% CO2.
Human primary GH-secreting PA cells were isolated from adenoma tissues as previously described [26]. Adenoma tissues were minced with a scalpel suspended in MEM and treated with collagenase I at a final concentration of 1 mg/ml for 2 h at 37℃. Undissociated tissue was removed using a 200-µm filter. Cells were collected by centrifugation, washed twice and then resuspended in MEM supplemented with 10% FBS.
The expression of p27Kip1 and SKP2 was regulated by transfection with either small interfering RNA for p27 or SKP2 (si-p27/si-SKP2) or p27/SKP2 overexpressing vectors (GeneCopoecia, Guangzhou, China). The expression of miR-186 was controlled by transfection with miR-186 mimics or inhibitors (GenePharma, Shanghai, China). Cell transfection was performed using Lipofectamine 2000 (Invitrogen, Waltham, MA, USA).
Total RNA was extracted using the TRIzol reagent (Invitrogen) following the manufacturer's protocol. SYBR green PCR Master Mix (Qiagen, Hilden, Germany) was used to detect messenger RNA (mRNA) expression following the manufacturer's protocol. GAPDH expression was used as an endogenous control. miRNA expression was determined using a Hairpin-it TM miRNAs qPCR kit (Genepharma) with RNU6B serving as an endogenous control. The 2−ΔΔCT method was used to analyze the relative fold changes.
Target cells were lysed using RIPA buffer supplemented with 1% phenylmethylsulfonyl fluoride. The proteins were extracted and analyzed to determine their concentration using the bicinchoninic acid protein assay kit (Beyotime Institute of Biotechnology, China). Proteins were then loaded onto a SDS-PAGE minigel and transferred onto a PVDF membrane. Afterward, the membrane was probed with the following antibodies at 4℃ overnight: anti-p27Kip1 (ab32034), anti-SKP2 (ab68455, Abcam) and anti-tubulin (ab6160, Abcam). Next, the blots were incubated with the horseradish peroxidase-conjugated secondary antibody immunoglobulin G (cat. P0448; Dako, Milano, Italy). Signal visualization was conducted using ECL Substrates (Millipore, Billerica, MA, USA) with tubulin serving as an endogenous protein for normalization. Gray intensity analysis was performed using ImageJ software (National Institutes of Health, Bethesda, MD, USA).
A Cell Counting Kit-8 (CCK-8; Beyotime) was used to determine the cell proliferation rate. Transfected cells were seeded in 96-well culture plates at a density of 0.5 × 104 cells/well, and the plates were incubated for 24, 48, 72 h. 1 h before the endpoint, 10 µl CCK-8 reagents was added into each well. The valus of optical density at 490 nm were measured using a microplate reader (Bio-Rad, Hercules, CA, USA).
The DNA synthesis in proliferating cells was determined by measuring 5-bromo-2′-deoxyuridine (BrdU) incorporation. BrdU assays were conducted at 24 and 48 h after transfection. Cells were seeded in 96-well culture plates at a density of 2 × 103 cells/well, cultured for 24 or 48 h, and then incubated with a 10 µM solution of BrdU (BD Pharmingen; San Diego, CA, USA) for 2 h. After the incubation, the medium was removed, and the cells were fixed for 30 min at room temperature, incubated with peroxidase-coupled anti-BrdU antibody (Sigma-Aldrich, St. Louis, MO, USA) for 60 min at room temperature, washed three times with phosphate-buffered saline (PBS), incubated with peroxidase substrate (tetramethylbenzidine) for 30 min, and then the 450 nm absorbance values were measured for each well. Background BrdU immunofluorescence was determined in cells not exposed to BrdU but stained with the BrdU antibody.
For the cell cycle analysis, cells were washed two times with ice-cold PBS, resuspended in 1 ml of ice-cold 70% ethanol with gentle vortexing and incubated at 4℃ overnight. The following day, the cells were washed with 1 × PBS and resuspended in buffer containing 10 µg/ml of propidium iodide, 10 µg/ml of RNase A and 0.1% Triton X-100. After incubating for 30 min at room temperature in the dark, the DNA content in the cells was measured using a flow cytometer (Guava easyCyte 8HT), and the data were analyzed using InCyte software.
Data were processed using SPSS ver. 17.0 statistical software (SPSS Inc., Chicago, IL, USA) and presented as the mean ± standard deviation of results from at least three independent experiments. Student's t-test was used for statistical comparison between means where applicable. Differences among more than two groups in the above assays were estimated using one-way ANOVA. A p-value less than 0.05 was considered statistically significant.
To assess the role of SKP2 and p27Kip1, we achieved SKP2 expressionby transfection with either si-SKP2 or SKP2 overexpressing vectors, as confirmed by immunoblotting (Fig. 1A, B). In rat GH3 and human GH-secreting PA cells, si-SKP2 increased p27Kip1 protein levels, while p27Kip1 protein levels were reduced by SKP2 overexpression (Fig. 1A, B).
The two cell lines transfected with either si-SKP2 or SKP2-overexpressing vectors were then examined for their DNA synthesis capacity and cell proliferation. As shown in Fig. 1C–F, SKP2 overexpression significantly promoted DNA synthesis and cell proliferation in both cell lines, while SKP2 knockdown resulted in opposite effects. Cell cycle alternation was examined. As shown in Fig. 1E, SKP knockdown induced cell cycle arrest in the G0/G1 phase, while SKP2 overexpression resulted in opposite effects. Importantly, the effects of SKP2 knockdown could be partially attenuated by the p27Kip1 knockdown. The above findings indicate that SKP2 modulates the proliferation and cell cycle of rat and human pituitary tumor cells via through p27Kip1.
Rat GH3 and human GH-secreting PA cells were transfected with miR-186 mimics or inhibitors to achieve miR-186 expression, as confirmed using real-time PCR (Fig. 2A). The mRNA expression and protein levels of SKP2 were significantly upregulated by miR-186 inhibition and downregulated by miR-186 overexpression in both cell lines (Fig. 2B, C).
To confirm direct binding of miR-186 to the 3′-untranslated region (UTR) of SKP2, two different SKP2 3′-UTR luciferase reporter gene vectors were constructed: wt-SKP2 3′-UTR containing the wild-type SKP2 3′-UTR with the predicted miR-186 binding site and mut-SKP2 3′-UTR containing a mutation in the predicted miR-186 binding site within the SKP2 3′-UTR (Fig. 2D). HEK293 cells were cotransfected with these two vectors and either miR-186 mimics or miR-186 inhibitors and then examined for luciferase activity. As shown in Fig. 2D, the luciferase activity of the wt-SKP2 3′-UTR was significantly suppressed by miR-186 mimics and while enhanced by miR-186 inhibitors. Importantly, mutation of the putative miR-186 binding site eliminated these effects on the luciferase activity.
After confirming that SKP2 is a direct downstream target of miR-186, functional experiments were performed. Rat GH3 and human GH-secreting PA cells were transfected with either miR-186 mimics or inhibitors, and then DNA synthesis capacity, cell proliferation, and cell cycle analyses were performed. The DNA synthesis capacity and proliferation of the two pituitary tumor cell lines were significantly suppressed by miR-186 overexpression and promoted by miR-186 inhibition (Fig. 3A, B). Overexpression miR-186 consistently induced cell cycle arrest of both two cell lines at G0/G1 phase, while miR-186 inhibition resulted in opposite effect (Fig. 3C).
To gain insight into the molecular mechanism behind these effects, we investigated the role of SKP2 in miR-186 function in pituitary tumor cell lines. Rat GH3 and human GH-secreting PA cells were cotransfected with SKP2 vector and miR-186 mimics, and then, the protein levels of SKP2 and p27Kip1 and the DNA synthesis capacity were examined. MiR-186 overexpression remarkably reduced the SKP2 protein level and increased the p27Kip1 protein level, while SKP2 overexpression resulted in opposite effects. Importantly, the effect of miR-186 overexpression could be partially attenuated by SKP2 overexpression (Fig. 3D). Consistently, the DNA synthesis capacity and proliferation of rat GH3 and human GH-secreting PA cells was suppressed by miR-186 overexpression and enhanced by SKP2 overexpression; the effects of miR-186 overexpression could be partially reversed by SKP2 overexpression (Fig. 3F, G), suggesting that miR-186 modulates the proliferation of pituitary tumor cell lines via SKP2 and downstream p27Kip1.
To further confirm the above findings, the expression of miR-186, SKP2 and p27Kip1 were examined in 5 normal pituitary tissues and 20 GH-producing pituitary tumor tissues. As shown in Fig. 4A–C, miR-186 and p27Kip1 mRNA expression were significantly downregulated while SKP2 expression was upregulated in tumor tissues. Moreover, immunoblotting and IHC results showed that the p27Kip1 protein levels were also lower in pituitary tumor tissues (Fig. 4D–E). Spearman's rank correlation analysis showed that SKP2 expression was negatively correlated with miR-186 and p27Kip1 expression and that miR-186 expression was positively correlated with p27Kip1 expression (Fig. 4F–H).
In the present study, the mRNA expression of SKP2 was found to be significantly upregulated while that of p27Kip1 was downregulated in rat and human pituitary tumor cell lines. SKP2 knockdown induced cell cycle arrest at the G0/G1 phase while p27Kip1 knockdown promoted the release of rehabilitated cells back into the proliferating pool. Through direct binding to the 3′-UTR of SKP2, miR-186 negatively regulates SKP2 expression and thus modulates pituitary tumor cell proliferation and cell the cycle through SKP2 and its downstream target p27Kip1.
The expression of the p27Kip1 protein is higher in quiescent cells but decreases when cells begin to proliferate. p27Kip1 is well known for its primary function in maintaining the inactive state in mammalian cells [2728]. The effects of p27Kip1 on cell cycle progression are mainly due to the binding and inactivation of cyclin E-CDK2 complexes at the G1/S transition [29]. Overexpression of p27Kip1 not only induces not only cell cycle arrest and loss of cyclin-CDK activity but also triggers apoptosis in human breast cancer cells and several other cancer cell lines [3031]. However, the p27Kip1 expression is inhibited in cancer by SKP2 through direct targeting [101112]. In the present study, the mRNA expression of SKP2 was significantly upregulated while that of p27Kip1 was downregulated in rat and human pituitary tumor cell lines. Consistent with previous studies, p27Kip1 mRNA expression was negatively correlated with that of SKP2 in pituitary tissues. Inhibiting SKP2 expression to rescue p27kip1 expression might eliminate the overproliferation and cell cycle dysregulation of pituitary tumor cells.
As discussed above, miRNAs play a vital role in the initiation and development of pituitary tumors by modulating tumor cell proliferation, apoptosis and the cell cycle [192021]. They target mRNA in a sequence-specific manner, inducing translational repression or mRNA degradation, depending on the degree of complementarity between the miRNA and the 3′-UTR region of its target [32]. A series of miRNAs, including miR-329, miR-300, miR-381 and miR-655, were reported to target the pituitary tumor transforming gene (PTTG1), a newly discovered oncogene, to inhibit its expression and thereby inhibit pituitary tumor cell tumorigenesis [33]. Similarly, miR-132, miR-15a, and miR-16 target SOX5 to inhibit pituitary tumor cell proliferation, invasion, and migration [34]. In the present study, miR-186, which has been reported to be downregulated in pituitary tumor tissues [4], was predicted by online tools to bind the SKP2 3′-UTR directly. In rat and human pituitary tumor cell lines, miR-186 was found to regulate SKP2 expression negatively. Furthermore, the direct binding of miR-186 to the SKP2 3′-UTR was also verified.
The tumor-suppressive role of miR-186 has been widely reported. In lung adenocarcinoma, miR-186 downregulation correlated with poor survival due to dysregulation of the cell cycle [35]. In bladder cancer, miR-186 downregulated the protein phosphatase PPM1B and mediated the G1-S phase transition [36]. In prostate cancer, miR-186 blocked the G1/S transition and inhibited cell proliferation by targeting GOLPH3 [37]. Herein, the function of miR-186 in pituitary tumor cell proliferation was assessed. Consistent with its role in other cancers, miR-186 inhibition promoted cell proliferation and the G1-S phase transition in rat pituitary tumor cells, whereas miR-186 overexpression resulted in the opposite effects. More importantly, the levels of the SKP2 downstream protein p27Kip1 were increased by miR-186 overexpression. The suppressive effects of miR-186 overexpression on p27Kip1 protein levels and the proliferation of rat pituitary tumor cell could be partially attenuated by SKP2 overexpression, indicating that miR-186 may modulate tumor cell proliferation and the cell cycle via SKP2/p27Kip1.
As further confirmation of the above findings, miR-186 and p27Kip1 expression were downregulated while SKP2 expression was upregulated in human pituitary tumor tissues. Thus, SKP2 expression negatively correlated with that of miR-186 and p27Kip1 while miR-186 expression positively associated with that of p27Kip1 in tissue samples. In summary, the miR-186/SKP2 axis modulates pituitary tumor cell proliferation and p27-mediated cell cycle regulation. These findings should be further explored by in vivo experiments and studies with a larger sample size in the future.
Figures and Tables
Fig. 1
The expression and combined function of SKP2 and p27Kip1 in pituitary tumor cell lines.
(A, B) SKP2 expression in rat GH3 and human GH-secreting PA cells was regulated by transfection with si-SKP2 or SKP2 overexpressing vectors, as confirmed by immunoblot assays. Negative control small RNA interference (si-NC) or an empty vector (NC) served as a control. (C, D) Rat GH3 and human GH-secreting PA cells were transfected with si-SKP2 or SKP2 overexpressing vectors, and then DNA synthesis was examined using BrdU assays. (E, F) Cell proliferation was determined using CCK-8 assays. (G) Rat GH3 and human GH-secreting PA cells were cotransfected with si-SKP2 and si-p27Kip1, and then cell cycle analysis was performed using flow cytometer assays. The data are presented as the mean ± standard deviation of three independent experiments. *p < 0.05 and **p < 0.01, compared to the si-NC or SKP2 overexpression vector group. si, small interfering; NC, negative control; GH, growth hormone; PA, pituitary adenoma; BrdU, 5-bromo-2′-deoxyuridine; OD, optical density.
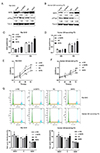
Fig. 2
SKP2 is a direct target of miR-186, which negatively regulates SKP2 expression.
(A) Rat GH3 and human GH-secreting PA cells were transfected with miR-186 mimics or inhibitors to regulate miR-186 expression, as confirmed using real-time PCR. (B) SKP2 mRNA expression in response to miR-186 overexpression or inhibition in rat GH3 and human GH-secreting PA cells was examined using real-time PCR. (C) SKP2 protein levels under the same conditions were examined using immunoblot assays. (D) Luciferase reporter gene assays were performed using wt-SKP2 3′-UTR or mut-SKP2 3′-UTR vectors to verify the direct binding between miR-186 and SKP2 3′-UTR. The data are presented as mean ± standard deviation of three independent experiments. *p < 0.05, **p < 0.01. miR, microRNA; mRNA, messenger RNA; GH, growth hormone; PA, pituitary adenoma; PCR, polymerase chain reaction; UTR, untranslated region; NC, negative control.
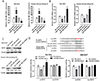
Fig. 3
The miR-186 modulates pituitary tumor cell proliferation and the cell cycle through SKP2.
(A) Rat GH3 and human GH-secreting PA cells were transfected with miR-186 mimics or inhibitors, and then, DNA synthesis was examined using BrdU assays. (B) Cell proliferation using CCK-8 assays. (C) Cell cycle analysis using flow cytometer assays. (D, E) Rat GH3 and human GH-secreting PA cells were cotransfected with miR-186 mimics and SKP2 overexpressing vectors, and then examined for the protein levels of SKP2 and p27Kip1 were examined using Immunoblot assays. (F) DNA synthesis was examined using BrdU assays. (G) Cell proliferation was examined using CCK-8 assays. The data are presented as the mean ± standard deviation of three independent experiments. *p < 0.05, **p < 0.01, compared to the control group; ##p < 0.01, compared to the SKP2 group. miR, microRNA; GH, growth hormone; PA, pituitary adenoma; BrdU, 5-bromo-2′-deoxyuridine; UTR, untranslated region; NC, negative control; OD, optical density.
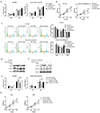
Fig. 4
The expression of and correlations among miR-186, SKP2 and p27Kip1 in human tissue samples.
(A–C) The mRNA expression of miR-186, SKP2 and p27kip1 in 5 normal human pituitary tissues and 20 human GH-producing pituitary tumor tissues was examined using real-time PCR. The data are presented as the mean ± standard deviation of three independent experiments. **p < 0.01. The protein levels of p27kip1 in normal pituitary tissues and pituitary tumor tissues were determined using immunoblot assays (D) and immunohistochemistry staining (E), respectively. The red arrows indicated p27kip1 positive cells. (F–H) The correlations among miR-186, SKP2 and p27Kip1 in tissue samples were analyzed using Spearman's rank correlation. mRNA, messenger RNA; GH, growth hormone; PCR, polymerase chain reaction.
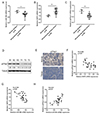
ACKNOWLEDGEMENTS
This study is supported by the grant from Department of Science and Technology of Sichuan Province, China (No. 2016FZ0092).
Notes
References
1. Shimon I, Melmed S. Genetic basis of endocrine disease: pituitary tumor pathogenesis. J Clin Endocrinol Metab. 1997; 82:1675–1681.

2. Coire CI, Horvath E, Kovacs K, Smyth HS, Ezzat S. Cushing's syndrome from an ectopic pituitary adenoma with peliosis: a histological, immunohistochemical, and ultrastructural study and review of the literature. Endocr Pathol. 1997; 8:65–74.


3. Asa SL, Ezzat S. The cytogenesis and pathogenesis of pituitary adenomas. Endocr Rev. 1998; 19:798–827.


4. Palumbo T, Faucz FR, Azevedo M, Xekouki P, Iliopoulos D, Stratakis CA. Functional screen analysis reveals miR-26b and miR-128 as central regulators of pituitary somatomammotrophic tumor growth through activation of the PTEN-AKT pathway. Oncogene. 2013; 32:1651–1659.


5. Tang FY, Shih CJ, Cheng LH, Ho HJ, Chen HJ. Lycopene inhibits growth of human colon cancer cells via suppression of the Akt signaling pathway. Mol Nutr Food Res. 2008; 52:646–654.

6. Li W, Zhang G, Wang HL, Wang L. Analysis of expression of cyclin E, p27kip1 and Ki67 protein in colorectal cancer tissues and its value for diagnosis, treatment and prognosis of disease. Eur Rev Med Pharmacol Sci. 2016; 20:4874–4879.

7. Berton S, Cusan M, Segatto I, Citron F, D'Andrea S, Benevol S, Avanzo M, Dall'Acqua A, Schiappacassi M, Bristow RG, Belletti B, Baldassarre G. Loss of p27kip1 increases genomic instability and induces radio-resistance in luminal breast cancer cells. Sci Rep. 2017; 7:595.



8. Kim GJ, Kim DH, Min KW, Kim YH, Oh YH. Loss of p27kip1 expression is associated with poor prognosis in patients with taxane-treated breast cancer. Pathol Res Pract. 2018; 214:565–571.
9. Haddad NF, Teodoro AJ, Leite de Oliveira F, Soares N, de Mattos RM, Hecht F, Dezonne RS, Vairo L, Goldenberg RC, Gomes FC, de Carvalho DP, Gadelha MR, Nasciutti LE, Miranda-Alves L. Lycopene and beta-carotene induce growth inhibition and proapoptotic effects on ACTH-secreting pituitary adenoma cells. PLoS One. 2013; 8:e62773.


10. Kulinski M, Achkar IW, Haris M, Dermime S, Mohammad RM, Uddin S. Dysregulated expression of SKP2 and its role in hematological malignancies. Leuk Lymphoma. 2018; 59:1051–1063.


11. Ma Y, Yan M, Huang H, Zhang L, Wang Q, Zhao Y, Zhao J. Associations and prognostic significance of p27Kip1, Jab1 and Skp2 in non-Hodgkin lymphoma. Mol Clin Oncol. 2016; 5:357–364.


12. Borriello A, Naviglio S, Bencivenga D, Caldarelli I, Tramontano A, Speranza MC, Stampone E, Sapio L, Negri A, Oliva A, Sinisi AA, Spina A, Della Ragione F. Histone deacetylase inhibitors increase p27(Kip1) by affecting its ubiquitin-dependent degradation through Skp2 downregulation. Oxid Med Cell Longev. 2016; 2016:2481865.
13. Frescas D, Pagano M. Deregulated proteolysis by the F-box proteins SKP2 and beta-TrCP: tipping the scales of cancer. Nat Rev Cancer. 2008; 8:438–449.


15. Iorio MV, Ferracin M, Liu CG, Veronese A, Spizzo R, Sabbioni S, Magri E, Pedriali M, Fabbri M, Campiglio M, Ménard S, Palazzo JP, Rosenberg A, Musiani P, Volinia S, Nenci I, Calin GA, Querzoli P, Negrini M, Croce CM. MicroRNA gene expression deregulation in human breast cancer. Cancer Res. 2005; 65:7065–7070.


16. Bandres E, Bitarte N, Arias F, Agorreta J, Fortes P, Agirre X, Zarate R, Diaz-Gonzalez JA, Ramirez N, Sola JJ, Jimenez P, Rodriguez J, Garcia-Foncillas J. microRNA-451 regulates macrophage migration inhibitory factor production and proliferation of gastrointestinal cancer cells. Clin Cancer Res. 2009; 15:2281–2290.


17. Stilling G, Sun Z, Zhang S, Jin L, Righi A, Kovācs G, Korbonits M, Scheithauer BW, Kovacs K, Lloyd RV. MicroRNA expression in ACTH-producing pituitary tumors: up-regulation of microRNA-122 and -493 in pituitary carcinomas. Endocrine. 2010; 38:67–75.


18. Bottoni A, Piccin D, Tagliati F, Luchin A, Zatelli MC, degli Uberti EC. miR-15a and miR-16-1 down-regulation in pituitary adenomas. J Cell Physiol. 2005; 204:280–285.


19. Wierinckx A, Roche M, Legras-Lachuer C, Trouillas J, Raverot G, Lachuer J. MicroRNAs in pituitary tumors. Mol Cell Endocrinol. 2017; 456:51–61.


20. Zhang QJ, Xu C. The role of microRNAs in the pathogenesis of pituitary tumors. Front Biosci (Landmark Ed). 2016; 21:1–7.


21. Sapochnik M, Nieto LE, Fuertes M, Arzt E. Molecular mechanisms underlying pituitary pathogenesis. Biochem Genet. 2016; 54:107–119.


22. Dweep H, Gretz N, Sticht C. miRWalk database for miRNA-target interactions. Methods Mol Biol. 2014; 1182:289–305.


23. John B, Enright AJ, Aravin A, Tuschl T, Sander C, Marks DS. Human MicroRNA targets. PLoS Biol. 2004; 2:e363.


24. Agarwal V, Bell GW, Nam JW, Bartel DP. Predicting effective microRNA target sites in mammalian mRNAs. Elife. 2015; 4:e05005.


25. Molatore S, Marinoni I, Lee M, Pulz E, Ambrosio MR, degli Uberti EC, Zatelli MC, Pellegata NS. A novel germline CDKN1B mutation causing multiple endocrine tumors: clinical, genetic and functional characterization. Hum Mutat. 2010; 31:E1825–E1835.


26. An J, Pei X, Zang Z, Zhou Z, Hu J, Zheng X, Zhang Y, He J, Duan L, Shen R, Zhang W, Zhu F, Li S, Yang H. Metformin inhibits proliferation and growth hormone secretion of GH3 pituitary adenoma cells. Oncotarget. 2017; 8:37538–37549.



27. Sherr CJ, Roberts JM. Inhibitors of mammalian G1 cyclin-dependent kinases. Genes Dev. 1995; 9:1149–1163.


28. Sherr CJ, Roberts JM. CDK inhibitors: positive and negative regulators of G1-phase progression. Genes Dev. 1999; 13:1501–1512.


29. Muşat M, Vax VV, Borboli N, Gueorguiev M, Bonner S, Korbonits M, Grossman AB. Cell cycle dysregulation in pituitary oncogenesis. Front Horm Res. 2004; 32:34–62.

30. Craig C, Wersto R, Kim M, Ohri E, Li Z, Katayose D, Lee SJ, Trepel J, Cowan K, Seth P. A recombinant adenovirus expressing p27Kip1 induces cell cycle arrest and loss of cyclin-Cdk activity in human breast cancer cells. Oncogene. 1997; 14:2283–2289.


31. Katayose Y, Kim M, Rakkar AN, Li Z, Cowan KH, Seth P. Promoting apoptosis: a novel activity associated with the cyclin-dependent kinase inhibitor p27. Cancer Res. 1997; 57:5441–5445.

33. Liang HQ, Wang RJ, Diao CF, Li JW, Su JL, Zhang S. The PTTG1-targeting miRNAs miR-329, miR-300, miR-381, and miR-655 inhibit pituitary tumor cell tumorigenesis and are involved in a p53/PTTG1 regulation feedback loop. Oncotarget. 2015; 6:29413–29427.



34. Renjie W, Haiqian L. MiR-132, miR-15a and miR-16 synergistically inhibit pituitary tumor cell proliferation, invasion and migration by targeting Sox5. Cancer Lett. 2015; 356:568–578.


35. Cai J, Wu J, Zhang H, Fang L, Huang Y, Yang Y, Zhu X, Li R, Li M. miR-186 downregulation correlates with poor survival in lung adenocarcinoma, where it interferes with cell-cycle regulation. Cancer Res. 2013; 73:756–766.

