Abstract
Fumigaclavine C (FC), an active indole alkaloid, is obtained from endophytic Aspergillus terreus (strain No. FC118) by the root of Rhizophora stylosa (Rhizophoraceae). This study is designed to evaluate whether FC has anti-adipogenic effects in 3T3-L1 adipocytes and whether it ameliorates lipid accumulation in high-fat diet (HFD)-induced obese mice. FC notably increased the levels of glycerol in the culture supernatants and markedly reduced lipid accumulation in 3T3-L1 adipocytes. FC differentially inhibited the expressions of adipogenesis-related genes, including the peroxisome proliferator-activated receptor proteins, CCAAT/enhancer-binding proteins, and sterol regulatory element-binding proteins. FC markedly reduced the expressions of lipid synthesis-related genes, such as the fatty acid binding protein, lipoprotein lipase, and fatty acid synthase. Furthermore, FC significantly increased the expressions of lipolysis-related genes, such as the hormone-sensitive lipase, Aquaporin-7, and adipose triglyceride lipase. In HFD-induced obese mice, intraperitoneal injections of FC decreased both the body weight and visceral adipose tissue weight. FC administration significantly reduced lipid accumulation. Moreover, FC could dose-dependently and differentially regulate the expressions of lipid metabolism-related transcription factors. All these data indicated that FC exhibited anti-obesity effects through modulating adipogenesis and lipolysis.
Obesity is a major risk of serious diet-related chronic diseases, such as hyperlipidemia, hypertension, type II diabetes, atherosclerosis, cardiovascular disease, non-alcoholic fatty liver disease, and metabolic syndrome [123]. These obesity-related syndromes are caused by triglyceride (TG) overload and fat deposition [45]. When TG levels are excessive, TG overload will cause lipid accumulation and interfere with the normal function of adipocytes. Excess fat deposition is causally linked to metabolic syndrome abnormalities [67]. Some studies have suggested that adipogenesis and lipolysis were considered as the complex processes involving several transcription factors, such as lipogenesis genes (the peroxisome proliferator-activated receptors [PPAR-α, PPAR-β, and PPAR-γ], CCAAT/enhancer-binding proteins [C/EBP-α and C/EBP-β], and sterol regulatory element-binding proteins [SREBP-1a, SREPB-1c, and SREBP-2]) [89], lipid synthesis enzymes (the fatty acid-binding protein [aP2], lipoprotein lipase [LPL], and fatty acid synthase [FAS]) [101112], and lipolysis genes (the hormone-sensitive lipase [HSL], aquaporin-7 [AQP-7], and adipose triglyceride lipase [ATGL]) [131415]. Hence, blocking the expressions of lipid metabolism-related transcription factors and their productions would be an impactful way to ameliorate lipid accumulation and improve obesity-related chronic diseases.
Long-term dietary supplementation intervention is important for humans at risk of developing adiposity [1617]. Teas have long been considered as a dietary component [18]. Rhizophora stylosa (Rhizophoraceae) is identified as a welcome edible plant whose leaves and roots are commonly used as an ingredient for herbal tea in Southern China [19]. As shown in Fig. 1A, fumigaclavine C (FC), an active indole alkaloid, is obtained from endophytic Aspergillus terreus (strain No. FC118) by the root of Rhizophora stylosa (Rhizophoraceae). FC possesses multiple health beneficial effects, including anti-inflammation [20], anti-atherosclerosis [21], anti-tumor [22], hepatoprotective activity [23], and immunosuppressive activity [24]. A previous study indicated that FC had a potential anti-atherosclerosis activity in apolipoprotein E-deficient mice via activating PPARs signaling pathway [25]. In high-fat diet (HFD)-induced obese animal model, the fat intake of obesity is accompanied with a low-grade inflammation characteristic [262728]. However, the anti-obesity effect of FC and the precise molecular mechanisms are incompletely delineated. Hence, this study is designed to evaluate whether FC has anti-adipogenic effect in adipocytes and whether it improves lipid accumulation in HFD-induced obese mice.
FC (purity is 99.5% by high performance liquid chromatography) is obtained from endophytic Aspergillus terreus (strain No. FC118) by the root of Rhizophora stylosa (Rhizophoraceae). The primary antibodies including PPAR-α, PPAR-β, PPAR-γ, C/EBP-α, C/EBP-β, SREBP-1c, aP2, LPL, FAS, HSL, AQP-7, ATGL, β-actin, and alkaline phosphatase labeled secondary antibody were purchased from Cell Signaling Technology (Danvers, MA, USA). Glycerol, TG, total cholesterol (TC), and Cell Counting Kit-8 (CCK-8) were evaluated via diagnostic assay kits from Nanjing Jiancheng Company (Nanjing, China). Simvastatin (Sim) was purchased from Sigma Aldrich Company (St. Louis, MO, USA).
3T3-L1 pre-adipocyte cells were purchased from American Type Culture Collection (ATCC, Rockville, MD, USA). The cells were cultured in dulbelcos modified eagles medium (DMEM) containing heat-inactivated fetal calf serum (10%), streptomycin (100 µg/ml), and penicillin (100 U/ml) at 37℃ with 5% CO2. 3T3-L1 adipocyte cells viability was evaluated by CCK-8 diagnostic assay kit following the manufacture's specification. The cells were stimulated with 1-isobutyl-3-methylxanthine (0.5 mM), dexamethasone (1 µM), and insulin (10 µg/ml) in 6-well plates for 48 h. The medium was then replaced with DMEM containing insulin (10 µg/ml) for 48 h. These cells were incubated in DMEM without insulin every 48 h until 96 h. FC was dissolved in DMSO (final concentration ≤ 0.1%). The differentiated 3T3-L1 adipocyte cells were treated with various concentrations of FC for 24 h. Cell supernatants, proteins, and RNA extracts were stored in −80℃ refrigerator. Treatment with Sim (10 µM) was used as a positive control.
Four-week-old male C57BL/6 mice were purchased from SJA Laboratory Animal Co., Ltd. (Hunan, China), and were acclimatized to the experimental facility for one week. The mice were kept in a temperature and humidity controlled room with ad libitum access to water and mouse chow diet (CD, 10% fat, 14% protein, and 76% carbohydrates, 3.6 kcal/g) or high fat diet (HFD, 50% fat, 14% protein, and 36% carbohydrates, 5.1 kcal/g [Shanghai FBSH Biological Pharmaceutical Co., Ltd., Shanghai, China]) for sixteen weeks. All experimental procedures and care administered were approved by the Institutional Animal Care and Use Committee of Liuzhou General Hospital (Protocol No. 1019-3). Experiments with animals were performed following the animal ethics guidelines of the Institutional Animal Ethics Committee. All animals were randomly subdivided into five groups with ten mice in each group who were treated as follows for fifteen weeks. FC (10 and 20 mg/kg of body weight) and Sim (20 mg/kg of body weight) were dissolved in DMSO (final concentration ≤ 5%). Mice from the control group were given CD diet and injected intraperitoneally with DMSO. Mice from the HFD-induced group were fed HFD diet and injected intraperitoneally with DMSO. Mice from the FC group were fed HFD diet and injected intraperitoneally with FC (10 and 20 mg/kg of body weight). Mice from the positive control group were fed HFD diet and injected intraperitoneally with Sim (20 mg/kg of body weight). The intraperitoneal injections were performed three times a week for ten weeks (mouse age from eleven weeks to twenty weeks).
Body weight was recorded twice a week throughout the experiment. All animals were sacrificed by decapitation 6 h after fasting. Blood samples were collected and centrifuged at 4,000 rpm for 15 min at 4℃. Visceral adipocyte tissues (white fat tissues) were dissected immediately, rinsed with cold physiologic saline solution, weighed, frozen in liquid nitrogen and stored at −80℃ for further analysis. Glycerol, TG, and TC were respectively detected via diagnostic assay kits from Nanjing Jiancheng Company following the manufacture's protocol.
Differentiated 3T3-L1 adipocytes were treated with FC or Sim for 24 h and fixed with formalin as described [29]. Next, cells were stained with oil red O for 1 h. The cells were treated with isopropanol and lipid accumulation was determined by the absorbance at optical density 490 nm.
The cells and visceral adipocyte tissues were lysed with lysis buffer containing a mixture of protease and phosphatase inhibitors in ice condition for 30 min. The protein extracts were harvested by centrifugation at 13,000 rpm for 20 min at 4℃. Protein samples were quantified by using an Enhanced Bicinchoninic Acid Protein Assay Kit (Beyotime, Jiangsu, China). Fifty micrograms of protein was separated on 10% sodium dodecyl sulfatepolyacrylamide gel and electrotransferred to polyvinylidene fluoride membranes (Millipore, Bedford, MA, USA). The membranes were blocked with 5% non-fat milk in Tris-buffered saline, and then probed with primary antibodies (1:1,000) for PPAR-α, PPAR-β, PPAR-γ, C/EBP-α, C/EBP-β, SREBP-1c, aP2, LPL, FAS, HSL, AQP-7, ATGL, and β-actin at 4℃ overnight. β-actin was used as a loading control. After three washing with TBST, the membranes were incubated with alkaline phosphatase labeled secondary antibody. Membranes were stained via 5-bromo-4-chloro-3-indolyl phosphate/nitro blue tetrazolium at room temperature for 10 min. Finally, the relative band intensity was normalized by β-actin and determined by software Quantity One v4.62 (Bio-Rad, Inc., Berkeley, CA, USA).
Total RNA was extracted from differentiated 3T3-L1 adipocytes and visceral adipocyte tissue using TRIzol reagent according to the manufacture's protocol (Invitrogen, Carlsbad, CA, USA). First-strand cDNA was synthesized using the cDNA synthesis system (Invitrogen). Quantitative real-time PCR was performed using the Quanti Fast SYBR Green PCR Kit (Qiagen, Valencia, CA, USA). The PCR primers used were shown in Table 1. The threshold cycle (Ct) value for each gene was normalized by β-actin.
The data were presented as means ± standard error of the mean of triplicate tests. All results were analyzed via one-way ANOVA followed by the Newman-Keuls test. Calculations were performed using IBM SPSS version 21.0 (IBM Co., Armonk, NY, USA). Differences of p < 0.05 were considered statistical significant.
As shown in Fig. 1B, the cytotoxic effect analysis indicated that FC at the tested concentrations showed no significant cytotoxicity in 3T3-L1 adipocytes. To evaluate whether FC had potential anti-adipogenic effect, we determined lipid accumulation by the absorbance method in differentiated 3T3-L1 adipocytes. As shown in Fig. 1C, FC could dose-dependently reduce lipid accumulation, and the maximum inhibition was at concentration of 10 µM (p < 0.01). Effect of FC (10 µM) could be comparable to positive reference Sim (10 µM). Glycerol was detected via diagnostic assay kit. As shown in Fig. 1D, FC notably increased the levels of glycerol in the culture supernatants. Effect of FC (10 µM) could be comparable to positive reference Sim (10 µM). These results indicated that FC showed no significant cytotoxicity and reduced lipid accumulation in differentiated 3T3-L1 adipocytes.
As shown in Fig. 2A, B, the tested concentrations of FC dose-dependently inhibited the protein expressions of PPAR-γ, but not of PPAR-α and PPAR-β. However, FC significantly suppressed the protein expressions of C/EBP-α, C/EBP-β, and SREBP-1c in differentiated 3T3-L1 adipocytes. The maximum inhibition was at concentration of 10 µM (p < 0.01). Effect of FC (10 µM) could be comparable to positive reference Sim (10 µM). Furthermore, as shown in Fig. 2C, FC treatment markedly down-regulated the mRNA expressions of PPAR-γ, C/EBP-α, C/EBP-β, and SREBP-1c, which showed a similar trend to the changes of the protein expression. Together these results suggested that FC differentially inhibited the expressions of adipogenesis-related factors in differentiated 3T3-L1 adipocytes.
As shown in Fig. 3A, B, the tested concentrations of FC dose-dependently suppressed the protein expressions of aP2, LPL, and FAS in differentiated 3T3-L1 adipocytes, and the maximum inhibition was at concentration of 10 µM (p < 0.01). Effect of FC (10 µM) could be comparable to positive reference Sim (10 µM). Furthermore, as shown in Fig. 3C, FC treatment markedly down-regulated the mRNA expressions of aP2, LPL, and FAS, which showed a similar trend to the changes of the protein expression. These data demonstrated that FC suppressed the expressions of lipid synthesis-related factors in differentiated 3T3-L1 adipocytes.
As shown in Fig. 4A, B, the tested concentrations of FC dose-dependently increased the protein expressions of HSL, AQP-7, and ATGL in differentiated 3T3-L1 adipocytes, and the maximum inhibition was at concentration of 10 µM (p < 0.01). Effect of FC (10 µM) could be comparable to positive reference Sim (10 µM). Furthermore, as shown in Fig. 4C, FC treatment markedly up-regulated the mRNA expressions of HSL, AQP-7, and ATGL, which showed a similar trend to the changes of the protein expression. These results demonstrated that FC increased the expressions of lipolysis-related factors in differentiated 3T3-L1 adipocytes.
Five-week-old male C57BL/6 mice were fed HFD diet, the lipid profiles including the body weight (Fig. 5A), visceral adipose tissue weight (Fig. 5B), TG (Fig. 5C), and TC (Fig. 5D) were significantly (p < 0.01) elevated. Then, these obese mice were treated with FC by intraperitoneal injection. As shown in Fig. 5A, B, FC administration markedly decreased both the body weight and visceral adipose tissue weight of HFD-induced obese mice. Meanwhile, the plasma levels of TG and TC were also markedly decreased in the FC group compared with the HFD-induced group (Fig. 5C, D). Effect of FC (20 mg/kg of body weight) could be comparable to positive reference Sim (20 mg/kg of body weight). These data indicated that FC improved lipid accumulation in HFD-induced obese mice.
As expected (Fig. 6A), the data of real-time PCR analysis showed that FC notably down-regulated the mRNA expressions of PPAR-γ, C/EBP-α, C/EBP-β, SREBP-1c, aP2, LPL, and FAS, and also markedly up-regulated the mRNA expressions of HSL, AQP-7, and ATGL in mouse visceral adipocyte tissue, compared with the HFD-induced group. Intriguingly, FC could not regulate the mRNA expressions of PPAR-α and PPAR-β in mouse visceral adipocyte tissue, which showed a similar trend to the changes of the mRNA expression in differentiated 3T3-L1 adipocytes. Furthermore, as shown in Fig. 6B, C, FC administration differentially affected these protein expressions, which showed a similar trend to the changes of the mRNA expression. Together these results demonstrated that FC could dose-dependently and differentially regulate the expressions of lipid metabolism-related factors in HFD-induced obese mice.
Obesity is a major risk of serious diet-related chronic diseases, such as hyperlipidemia, hypertension, type II diabetes, atherosclerosis, cardiovascular disease, non-alcoholic fatty liver disease, and metabolic syndrome [123]. In the present study, we indicated that FC could dose-dependently and differentially regulate the expressions of lipid metabolism-related factors in vitro and in vivo. Our results demonstrated that FC had anti-obesity effects via modulating adipogenesis and lipolysis.
Obesity-related syndromes are caused by TG overload and fat deposition [45]. When TG levels are excessive, TG overload will cause lipid accumulation and interfere with the normal function of adipocytes. Excess fat deposition is causally linked to metabolic syndrome abnormalities [67]. On one hand, FC could dose-dependently reduce lipid accumulation in differentiated 3T3-L1 adipocytes. On the other hand, FC could notably increase the levels of glycerol in the culture supernatants. These findings suggested that FC exhibited anti-obesity effects via suppressing lipid accumulation.
Adipogenesis is considered as the complex processes that preadipocytes changed into differentiated mature adipocytes [30]. Pre-adipocytes require the sequential action of adipogenesis-related genes to induce their differentiation into mature adipocytes [29]. The PPARs affect adipocytes differentiation and regulate adipogenesis. PPAR-α and PPAR-β affect the early stage of adipocytes differentiation, while PPAR-γ is involved in the later stage [8]. In the present study, the tested concentrations of FC dose-dependently inhibited PPAR-γ gene and protein productions in differentiated 3T3-L1 adipocytes, but not of PPAR-α and PPAR-β. Furthermore, FC could not regulate the mRNA expressions of PPAR-α and PPAR-β in mouse visceral adipocyte tissue, which showed a similar trend to the changes of the mRNA expression in differentiated 3T3-L1 adipocytes. It is possible that the mechanism of anti-adipogenic effect of FC is distinct from statins. FC could not hydrolyze to the β-hydroxy acid form [3132333435]. Therefore, we evaluated the other transcription factors in the early stage of adipocytes differentiation. C/EBPs and SREBPs might be related to the early stage of adipocytes differentiation process [9]. FC treatment markedly decreased the levels of C/EBP-α, C/EBP-β, and SREBP-1c both in vitro and in vivo. These data demonstrated that FC differentially inhibited the expressions of adipogenesis-related factors.
Emerging studies have suggested that major lipid synthesis enzymes were aP2, LPL, FAS, and so on [101112]. aP2 regulates lipid and glucose metabolism in mature adipose tissue [10]. LPL hydrolyzes lipoproteins from chylomicrons to free TG in serum [11]. FAS catalyzes and synthesizes long-chain fatty acids [12]. FC treatment markedly decreased the levels of aP2, LPL, and FAS both in vitro and in vivo, compared with the other lipid metabolism-related genes. These results indicated that FC suppressed the expressions of lipid synthesis-related factors.
Lipolysis, a process of hydrolysis of TG and TC ester, is mainly measured by HSL, ATGL, AQP-7 and so on [13]. HSL and ATGL break down TG to reduce the visceral adipose tissue weight [14]. AQP-7 is an adipocyte membrane channel protein, and it affects the release of glycerol from adipocytes into serum [15]. In the present study, FC administration markedly decreased both the body weight and visceral adipose tissue weight of HFD-induced obese mice. Meanwhile, the levels of TG and TC were also markedly decreased. FC also notably increased the levels of HSL, AQP-7, and ATGL both in vitro and in vivo. These data demonstrated that FC could decrease TG and reduce the weight of HFD-induced obese mice.
In summary, the present study elucidated that FC exhibited anti-obesity effects via modulating adipogenesis and lipolysis in differentiated adipocytes and HFD-induced obese mice. The mechanisms were involved in regulating the expressions of lipid metabolism-related factors, attenuating adipogenesis, and reducing lipid accumulation.
Figures and Tables
Fig. 1
Effects of fumigaclavine C (FC) on adipogenesis in differentiated 3T3-L1 adipocytes.
(A) The chemical structure of FC. (B) Effects of FC on 3T3-L1 adipocytes viability. (C) Effects of FC on lipid accumulation in differentiated 3T3-L1 adipocytes. Cells were treated with isopropanol and lipid accumulation was determined by the absorbance at optical density 490 nm. (D) Effects of FC on levels of glycerol in differentiated 3T3-L1 adipocytes. Simvastatin (Sim, 10 µM) acted as the positive control group. Each value represents as means ± standard error of the mean of triplicate experiments. *p < 0.05 and **p < 0.01 as compared with the control group.
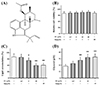
Fig. 2
Fumigaclavine C (FC) differentially regulates the expressions of adipogenesis-related genes and proteins.
Differentiated 3T3-L1 adipocytes were treated with FC or Simvastatin (Sim) for 24 h. These adipogenesis-related genes were evaluated via real-time polymerase chain reaction and Western blot technique. β-actin was used as an internal control. (A, B) Effects of FC on protein productions of adipogenesis-related genes in differentiated 3T3-L1 adipocytes. (C) Effects of FC on mRNA expressions of adipogenesis-related genes in differentiated 3T3-L1 adipocytes. Sim (10 µM) acted as the positive control group. Each value represents as means ± standard error of the mean of triplicate experiments. PPAR, peroxisome proliferator-activated receptors; C/EBP, CCAAT/enhancer-binding proteins; SREBP, sterol regulatory element-binding proteins. *p < 0.05 and **p < 0.01 as compared with the control group.
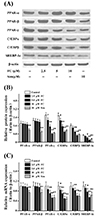
Fig. 3
Effects of fumigaclavine C (FC) on the expressions of lipid synthesis-related genes and proteins.
Differentiated 3T3-L1 adipocytes were treated with FC or Simvastatin (Sim) for 24 h. These lipid synthesis-related genes were evaluated via real-time polymerase chain reaction and Western blot technique. β-actin was used as an internal control. (A, B) Effects of FC on protein productions of lipid synthesis-related genes in differentiated 3T3-L1 adipocytes. (C) Effects of FC on mRNA expressions of lipid synthesis-related genes in differentiated 3T3-L1 adipocytes. Sim (10 µM) acted as the positive control group. Each value represents as means ± standard error of the mean of triplicate experiments. aP2, fatty acid-binding protein; LPL, lipoprotein lipase; FAS, fatty acid synthase. *p < 0.05 and **p < 0.01 as compared with the control group.
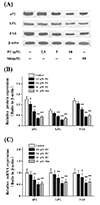
Fig. 4
Effects of fumigaclavine C (FC) on the expressions of lipolysis-related genes and proteins.
Differentiated 3T3-L1 adipocytes were treated with FC or Simvastatin (Sim) for 24 h. These lipolysis-related genes were evaluated via real-time polymerase chain reaction and Western blot technique. β-actin was used as an internal control. (A, B) Effects of FC on protein productions of lipolysis-related genes in differentiated 3T3-L1 adipocytes. (C) Effects of FC on mRNA expressions of lipolysis-related genes in differentiated 3T3-L1 adipocytes. Sim (10 µM) acted as the positive control group. Each value represents as means ± standard error of the mean of triplicate experiments. HSL, hormone-sensitive lipase; AQP-7, aquaporin-7; ATGL, adipose triglyceride lipase. *p < 0.05 and **p < 0.01 as compared with the control group.
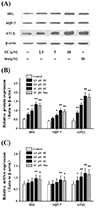
Fig. 5
Effects of fumigaclavine C (FC) administration on the body weight, visceral adipocyte tissue weight, and plasma levels of in high-fat diet (HFD)-induced obese mice.
Inhibitory effects of FC administration on the body weight (A) and visceral adipocyte tissue weight (B). Diagnostic kits were used to measure the plasma levels of triglyceride (TG) (C) and total cholesterol (TC) (D) in HFD-induced obese mice. Simvastatin (Sim) (20 mg/kg) acted as the positive control group. Each value represents as means ± standard error of the mean of 10 mice. #p < 0.01 as compared with the control group. *p < 0.05 and **p < 0.01 as compared with the HFD-induced group.
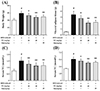
Fig. 6
Effects of fumigaclavine C (FC) administration on the lipid metabolism-related factors expressions in the visceral adipocyte tissue of high-fat diet (HFD)-induced obese mice.
Real-time polymerase chain reaction (A) and Western blotting (B, C) were used to measure the expressions of PPAR-α, PPAR-β, PPAR-γ, C/EBP-α, C/EBP-β, SREBP-1c, aP2, LPL, FAS, HSL, AQP-7, and ATGL in the visceral adipocyte tissue. Simvastatin (Sim) (20 mg/kg) acted as the positive control group. Each value represents as means ± standard error of the mean of 10 mice. PPAR, peroxisome proliferator-activated receptors; C/EBP, CCAAT/enhancer-binding proteins; SREBP, sterol regulatory element-binding proteins; aP2, fatty acid-binding protein; LPL, lipoprotein lipase; FAS, fatty acid synthase; HSL, hormone-sensitive lipase; AQP-7, aquaporin-7; ATGL, adipose triglyceride lipase. #p < 0.01 as compared with the control group. *p < 0.05 and **p < 0.01 as compared with the HFD-induced group.
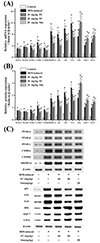
Table 1
Sequences of primers used for the real-time polymerase chain reaction analysis
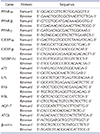
PPAR, peroxisome proliferator-activated receptors; C/EBP, CCAAT/enhancer-binding proteins; SREBP, sterol regulatory element-binding proteins; aP2, fatty acid-binding protein; LPL, lipoprotein lipase; FAS, fatty acid synthase; HSL, hormone-sensitive lipase; AQP-7, aquaporin-7; ATGL, adipose triglyceride lipase.
ACKNOWLEDGEMENTS
This study was supported by the Guangxi Natural Science Foundation (2016GXNSFBA380075 and 2017JJA120670y), the Open Fund of Key Laboratory for Processing of Sugar Resources of Guangxi Higher Education Institutes (2016TZYKF09 and GXTZY201706), the Special Fund for Guangxi University of Science and Technology (No. 16Z06), and the Young and Middle-aged Teachers Ability Promotion Project of Guangxi Autonomous Region (KY2016YB250).
Notes
References
1. Gangadaran S, Cheema SK. A high fat diet enriched with sea cucumber gut powder provides cardio-protective and anti-obesity effects in C57BL/6 mice. Food Res Int. 2017; 99:799–806.


2. Imai N, Suzuki M, Ishizu Y, Kuzuya T, Honda T, Hayashi K, Ishigami M, Hirooka Y, Ishikawa T, Goto H, Fujimoto T. Hepatocytespecific depletion of ubiquitin regulatory X domain containing protein 8 accelerates fibrosis in a mouse non-alcoholic steatohepatitis model. Histochem Cell Biol. 2017; 148:219–227.


3. Yu WG, He H, Yao JY, Zhu YX, Lu YH. Dimethyl cardamonin exhibits anti-inflammatory Effects via interfering with the PI3K-PDK1-PKCα signaling pathway. Biomol Ther (Seoul). 2015; 23:549–556.



4. Chandrashekaran V, Seth RK, Dattaroy D, Alhasson F, Ziolenka J, Carson J, Berger FG, Kalyanaraman B, Diehl AM, Chatterjee S. HMGB1-RAGE pathway drives peroxynitrite signaling-induced IBD-like inflammation in murine nonalcoholic fatty liver disease. Redox Biol. 2017; 13:8–19.



5. Tian F, Wu CL, Yu BL, Liu L, Hu JR. Apolipoprotein O expression in mouse liver enhances hepatic lipid accumulation by impairing mitochondrial function. Biochem Biophys Res Commun. 2017; 491:8–14.


6. Hirotani Y, Fukamachi J, Ueyama R, Urashima Y, Ikeda K. Effects of capsaicin coadministered with eicosapentaenoic acid on obesity-related dysregulation in high-fat-fed mice. Biol Pharm Bull. 2017; 40:1581–1585.


7. Kang HH, Kim IK, Lee HI, Joo H, Lim JU, Lee J, Lee SH, Moon HS. Chronic intermittent hypoxia induces liver fibrosis in mice with diet-induced obesity via TLR4/MyD88/MAPK/NF-κB signaling pathways. Biochem Biophys Res Commun. 2017; 490:349–355.

8. Pražienková V, Holubová M, Pelantová H, Bugáňová M, Pirník Z, Mikulášková B, Popelová A, Blechová M, Haluzík M, Železná B, Kuzma M, Kuneš J, Maletínská L. Impact of novel palmitoylated prolactin-releasing peptide analogs on metabolic changes in mice with diet-induced obesity. PLoS One. 2017; 12:e0183449.


9. Lee JH, Kang HS, Park HY, Moon YA, Kang YN, Oh BC, Song DK, Bae JH, Im SS. PPARα-dependent Insig2a overexpression inhibits SREBP-1c processing during fasting. Sci Rep. 2017; 7:9958.


10. Wang Y, Viscarra J, Kim SJ, Sul HS. Transcriptional regulation of hepatic lipogenesis. Nat Rev Mol Cell Biol. 2015; 16:678–689.



11. Mota de Sá P, Richard AJ, Hang H, Stephens JM. Transcriptional regulation of adipogenesis. Compr Physiol. 2017; 7:635–674.

12. Tang Q, Jiang S, Jia W, Shen D, Qiu Y, Zhao Y, Xue B, Li C. Zoledronic acid, an FPPS inhibitor, ameliorates liver steatosis through inhibiting hepatic de novo lipogenesis. Eur J Pharmacol. 2017; 814:169–177.


13. Hsiao PJ, Chiou HC, Jiang HJ, Lee MY, Hsieh TJ, Kuo KK. Pioglitazone enhances cytosolic lipolysis, β-oxidation and autophagy to ameliorate hepatic steatosis. Sci Rep. 2017; 7:9030.



14. Lass A, Zimmermann R, Oberer M, Zechner R. Lipolysis - a highly regulated multi-enzyme complex mediates the catabolism of cellular fat stores. Prog Lipid Res. 2011; 50:14–27.



15. Lebeck J. Metabolic impact of the glycerol channels AQP7 and AQP9 in adipose tissue and liver. J Mol Endocrinol. 2014; 52:R165–R178.


16. Yu WG, He H, Qian J, Lu YH. Dual role of 2′,4′-dihydroxy-6′-methoxy-3′,5′-dimethylchalcone in inhibiting high-mobility group box 1 secretion and blocking its pro-inflammatory activity in hepatic inflammation. J Agric Food Chem. 2014; 62:11949–11956.


17. Ding S, Jiang J, Zhang G, Bu Y, Zhang G, Zhao X. Resveratrol and caloric restriction prevent hepatic steatosis by regulating SIRT1-autophagy pathway and alleviating endoplasmic reticulum stress in high-fat diet-fed rats. PLoS One. 2017; 12:e0183541.


18. Wang S, Huang Y, Xu H, Zhu Q, Lu H, Zhang M, Hao S, Fang C, Zhang D, Wu X, Wang X, Sheng J. Oxidized tea polyphenols prevent lipid accumulation in liver and visceral white adipose tissue in rats. Eur J Nutr. 2017; 56:2037–2048.


19. Wang Y, Zhu H, Tam NFY. Polyphenols, tannins and antioxidant activities of eight true mangrove plant species in South China. Plant Soil. 2014; 374:549–563.


20. Tan Y, Wu X, Sun J, Guo W, Gong F, Shao F, Tan T, Cao Y, Zheng B, Gu Y, Sun Y, Xu Q. A fumigaclavine C isostere alleviates Th1-mediated experimental colitis via competing with IFN-γ for binding to IFN-γ receptor 1. Biochem Pharmacol. 2017; 123:63–72.


21. Zhao Y, Liu J, Wang J, Wang L, Yin H, Tan R, Xu Q. Fumigaclavine C improves concanavalin A-induced liver injury in mice mainly via inhibiting TNF-α production and lymphocyte adhesion to extracellular matrices. J Pharm Pharmacol. 2004; 56:775–782.


22. Guo W, Hu S, Elgehama A, Shao F, Ren R, Liu W, Zhang W, Wang X, Tan R, Xu Q, Sun Y, Jiao R. Fumigaclavine C ameliorates dextran sulfate sodium-induced murine experimental colitis via NLRP3 inflammasome inhibition. J Pharmacol Sci. 2015; 129:101–106.


23. Yu W, Pan Z, Zhu Y, An F, Lu Y. Fumigaclavine C exhibits anti-inflammatory effects by suppressing high mobility group box protein 1 relocation and release. Eur J Pharmacol. 2017; 812:234–242.


24. Du RH, Li EG, Cao Y, Song YC, Tan RX. Fumigaclavine C inhibits tumor necrosis factor α production via suppression of toll-like receptor 4 and nuclear factor κB activation in macrophages. Life Sci. 2011; 89:235–240.


25. Du RH, Qin SY, Shi LS, Zhou ZQ, Zhu XY, Liu J, Tan RX, Cao W. Fumigaclavine C activates PPARγ pathway and attenuates atherogenesis in ApoE-deficient mice. Atherosclerosis. 2014; 234:120–128.


26. Fenni S, Hammou H, Astier J, Bonnet L, Karkeni E, Couturier C, Tourniaire F, Landrier JF. Lycopene and tomato powder supplementation similarly inhibit high-fat diet induced obesity, inflammatory response, and associated metabolic disorders. Mol Nutr Food Res. 2017; 61:1601083.


27. Yu WG, Qian J, Lu YH. Hepatoprotective effects of 2′,4′-dihydroxy-6′-methoxy-3′,5′-dimethylchalcone on CCl4-induced acute liver injury in mice. J Agric Food Chem. 2011; 59:12821–12829.


28. Veiga FMS, Graus-Nunes F, Rachid TL, Barreto AB, Mandarim-de-Lacerda CA, Souza-Mello V. Anti-obesogenic effects of WY14643 (PPAR-alpha agonist): hepatic mitochondrial enhancement and suppressed lipogenic pathway in diet-induced obese mice. Biochimie. 2017; 140:106–116.

29. Liou CJ, Wu SJ, Chen LC, Yeh KW, Chen CY, Huang WC. Acacetin from traditionally used saussurea involucrata kar. et Kir. Suppressed adipogenesis in 3T3-L1 adipocytes and attenuated lipid accumulation in obese mice. Front Pharmacol. 2017; 8:589.



30. Hu YC, Zhang Z, Shi WG, Mi TY, Zhou LX, Huang N, Hoptroff M, Lu YH. 2′,4′-Dihydroxy-6′-methoxy-3′,5′-dimethylchalcone promoted glucose uptake and imposed a paradoxical effect on adipocyte differentiation in 3T3-L1 cells. J Agric Food Chem. 2014; 62:1898–1904.


31. Singh SP, Sashidhara KV. Lipid lowering agents of natural origin: an account of some promising chemotypes. Eur J Med Chem. 2017; 140:331–348.


32. Panahi Y, Ahmadi Y, Teymouri M, Johnston TP, Sahebkar A. Curcumin as a potential candidate for treating hyperlipidemia: a review of cellular and metabolic mechanisms. J Cell Physiol. 2018; 233:141–152.


33. Xiao S, Yu R, Ai N, Fan X. Rapid screening natural-origin lipase inhibitors from hypolipidemic decoctions by ultrafiltration combined with liquid chromatography-mass spectrometry. J Pharm Biomed Anal. 2015; 104:67–74.

