Abstract
Purpose
Materials and Methods
Figures and Tables
Fig. 1
The expression of C-type natriuretic peptide (CNP)/natriuretic peptide receptor 2 (NPR2) at different postnatal stages of testes. (A) The expression of CNP/NPR2 mRNA in testes and Leydig cells. (B, C) The location by immunohistochemistry (×200) and expression by Western blot of NPR2 in testes and Leydig cells. (D) The expression of CNP at mouse different postnatal stages of testes. (E) The expression of NPR2 at mouse different postnatal stages of testes. The β-actin was used as internal control. The data are presented as the mean±standard error of mean of three independent experiments. Bars with different letters are significantly different (p<0.05).
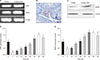
Fig. 2
Effects of C-type natriuretic peptide (CNP) on cyclic guanosine monophosphate (cGMP) production, natriuretic peptide receptor 2 (NPR2) expression, cell proliferation, testosterone secretion in mouse Leydig cells. (A) The cGMP production in mouse Leydig cells. (B) The NPR2 expression in mouse Leydig cells. (C) The cell activity in mouse Leydig cells. (D) The testosterone secretion in mouse Leydig cells. The β-actin was used as internal control. The data are presented as the mean±standard error of mean of three independent experiments. Bars with different letters are significantly different (p<0.05).
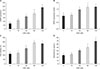
Fig. 3
Effects of natriuretic peptide receptor 2 (NPR2) knockdown on cyclic guanosine monophosphate (cGMP) production and cell proliferation in mouse Leydig cells. (A and B) The NPR2 expression in mRNA and Western blot levels in mouse Leydig transducted with shRNA-NPR2 lentivirus. (C) The cGMP production in mouse Leydig cells transducted with shRNA-NPR2 lentivirus. (D) The cell activity in mouse Leydig cells transducted with shRNA-NPR2 lentivirus. (E) The mRNA expression of cyclin A1, cyclin B1, and cyclin D2 in mouse Leydig cells transducted with shRNA-NPR2 lentivirus. The β-actin was used as internal control. The protein of NPR2 were normalized to that of β-actin. The results are presented as the mean±standard error of mean and are representative of three independent experiments. *p<0.05 and **p<0.01 compared with each corresponding group in the control.
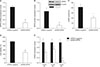
Fig. 4
Effects of natriuretic peptide receptor 2 (NPR2) knockdown on cells apoptosis in mouse Leydig cells. (A) Relative mRNA expression of cell apoptosis related genes (Bcl-2, Bax, p53, and Caspase-3) in mouse Leydig cells transducted with shRNA-NPR2 lentivirus. (B) The phosphorylation of AKT in mouse Leydig cells transducted with shRNA-NPR2 lentivirus. (C) Caspase-3 activity in mouse Leydig cells transducted with shRNA-NPR2 lentivirus. (D) Relative protein expression of Bcl-2 and Bax in mouse Leydig cells transducted with shRNA-NPR2 lentivirus. The β-actin was used as internal control. The phosphorylation of AKT were normalized to that of total AKT. The results are presented as the mean±standard error of mean and are representative of three independent experiments. *p<0.05 and **p<0.01 compared with each corresponding group in the control.
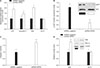
Fig. 5
Effect of natriuretic peptide receptor 2 (NPR2) knockdown on testosterone secretion in mouse Leydig cells. (A) The effects C-type natriuretic peptide (CNP) and human chorionic gonadotropin (hCG) on CNP/NPR2 expression. (B) Concentration of testosterone secretion in the culture medium transducted with shRNA-NPR2 lentivirus. (C) Relative mRNA expression of genes (Star, Cyp11a1, 3β-hydroxysteroid dehydrogenase [3β-HSD], insulin-like factor 3 [Insl3], and luteinizing hormone receptor [LHR]) in mouse Leydig cells transducted with shRNA-NPR2 lentivirus. (D) Relative protein expression of Star and Cyp11a1 in mouse Leydig cells transducted with shRNA-NPR2 lentivirus. The β-actin was used as internal control. The results are presented as the mean±standard error of mean and are representative of three independent experiments. Bars with different letters are significantly different (p<0.05).
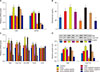
Fig. 6
The mechanism of C-type natriuretic peptide (CNP), testosterone, and estradiol in promoting natriuretic peptide receptor 2 (NPR2) expression in mouse Leydig cells. (A) The expression of NPR2 after stimulation with CNP, testosterone and estradiol with or without flutamide and ICI182780 in mouse Leydig cells. (B) The expression of Cyp19a1 after stimulation with CNP, testosterone and estradiol with or without flutamide and ICI182780 in mouse Leydig cells. The Σ-actin was used as internal control. The data are presented as the mean±standard error of mean of three independent experiments. Bars with different letters are significantly different (p<0.05).
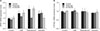
Table 1
Cell cycle value comparison between shRNA-negative and shRNA-natriuretic peptide receptor 2

Group | G1-phase (%) | S-phase (%) | G2-phase (%) |
---|---|---|---|
shRNA-negative | 63.37±2.35 | 15.43±1.83 | 21.23±2.49 |
shRNA-NPR2 | 57.33±1.49* | 23.18±2.28* | 19.67±1.34 |
ACKNOWLEDGEMENTS
Notes
Author Contribution
Conceptualization: Yang L.
Data curation: Huang S.
Formal analysis: Yang L.
Funding acquisition: Yang L, Huang S.
Investigation: Lei L, Zhao Q.
Methodology: Yang L, Gong Y, Guan G.
Project administration: Huang S.
Resources: Huang S.
Software: Lei L, Guan G.
Supervision: Gong Y.
Validation: Yang L.
Visualization: Yang L, Zhao Q.
Writing-original draft: Lei L.
Writing-review & editing: Yang L.
References




















































