Abstract
The present study was conducted to investigate anti-nociceptive and anti-inflammatory effects of the leaves of Ilex latifolia Thunb (I. latifolia) in in vivo and in vitro. Writhing responses induced by acetic acid and formalin- and thermal stimuli (tail flick and hot plate tests)-induced pain responses for nociception were evaluated in mice. I. latifolia (50 – 200 mg/kg, p.o.) and ibuprofen (100 mg/kg, p.o.), a positive non-steroidal anti-inflammatory drug (NSAID), inhibited the acetic acid-induced writhing response and the second phase response (peripheral inflammatory response) in the formalin test, but did not protect against thermal nociception and the first phase response (central response) in the formalin test. These results show that I. latifolia has a significant anti-nociceptive effect that appears to be peripheral, but not central. Additionally, I. latifolia (50 and 100 µg/mL) and 3,5-di-caffeoyl quinic acid methyl ester (5 µM) isolated from I. latifolia as an active compound significantly inhibited LPS-induced NO production and mRNA expression of the pro-inflammatory mediators, iNOS and COX-2, and the pro-inflammatory cytokines, IL-6 and IL-1β, in RAW 264.7 macrophages. These results suggest that I. latifolia can produce antinociceptive effects peripherally, but not centrally, via anti-inflammatory activity and supports a possible use of I. latifolia to treat pain and inflammation.
Although inflammation is a host defense mechanism caused by infection or injury, the inflammation process causes increased vascular permeability, active migration of blood cells, and the passage of plasma constituents into injurious tissue, resulting in pain, swelling, and erythema.1 Various inflammatory mediators such as prostaglandins (PGs), leukotrienes, histamine, bradykinin, and platelet-activating factor are involved in initiating and sustaining pain and inflammatory cascades. Arachidonic acid, which is released from phospholipids of cellular membranes by phospholipase A2, is catalyzed to produce PGs by cyclooxygenase (COX) during inflammatory responses.2 Prostaglandins themselves does not produce pain, but strongly enhance the pain-producing effect of 5-hydroxytryptamine or bradykinin.3 Therefore, inflammatory process are controlled by the aspirin-like non-steroidal anti-inflammatory drugs (NSAIDs), which mainly inhibit COX and reduce synthesis of PGs, as well as by corticosteroids, which inhibit phospholipase A2 and further prevent PGs formation. Cyclooxygenase exists in two isoforms, COX-1 and COX-2.4 COX-1 is responsible for maintaining normal physiological functions, whereas COX-2 is induced by pro-inflammatory cytokines under inflammatory conditions and synthesized in macrophages in response to injury or bacterial infection.5
Nitric oxide (NO), which is also known as an inflammatory mediator,6 is synthesized by inducible nitric oxide synthase (iNOS) in tissue to regulate immunity and defend hosts against infectious pathogens.7 However, excessive synthesis of NO results in destruction of tissue homeostasis and mediation of inflammation.8
Macrophages play key roles in inflammation by inducing inflammatory mediators, including NO, PGE2 and pro-inflammatory cytokines such as tumor necrosis factor alpha (TNF-α), interleukin (IL)-6, and IL-1β.9 Cytokines released from activated macrophages increase the expression of inflammatory mediators including iNOS and COX-2.10 The inhibition of pro-inflammatory cytokines and mediators is regarded as a primary target of anti-inflammatory agents.11
Ilex latifolia (I. latifolia) is a bitter tea of Chinese origin that has received a great deal of attention because of its many beneficial functions, which include cardiovascular, antioxidant, hepatoprotective, neuroprotective and anti-inflammatory effects.121314 Previous phytochemical investigations have shown that I. latifolia contains a large quantity of caffeoylquinic acids (CQAs) such as 3,5-dicaffeoylquinic acid (diCQA), 3,4-diCQA, 3-CQA, 5-CQA, and triterpenoid saponins, which may contribute to the beneficial effects of this plant.1516 Ethanol extract of I. latifolia leaves showed neuroprotective effects through antioxidative and anti-inflammatory action in stroke and AD models in previous studies.131417 Moreover, it has been hypothesized that I. latifolia may exert anti-inflammatory effects, which might be related to its neuroprotection on ischemic brain injury and memory impairment. Therefore, the present study was conducted to investigate anti-nociceptive properties of an ethanol extract of I. latifolia after applying different pain stimuli to mice. In addition, we investigated the anti-inflammatory effects of I. latifolia in LPS-induced RAW 264.7 macrophages to elucidate the underlying mechanism. Finally, it was suggested that 3,5-dicaffeoylquinic acid methyl ester (3,5-diCQA methyl ester) might be an active component of I. latifolia contributing to the anti-inflammatory effect.
Dried I. latifolia leaves were purchased from an herbal market in Hong Kong by Han Kook Shin Yak Co., Ltd. (Chungnam, South Korea) and identified by Dr. Bang Yeon Hwang (Chungbuk National University, Cheongju, South Korea). A voucher specimen (CBNU006) was deposited in the herbarium at the College of Veterinary Medicine, Chungbuk National University. The I. latifolia leaves (1 kg) were subjected to extraction with 5 L of 75% ethanol at room temperature for 2 h. The extract was then filtered through Whatman NO. 1 filter paper, after which the filtrate was concentrated using a rotary vacuum evaporator to yield an ethanol extract (220 g) that was stored at room temperature until required. 3,5-DiCQA methyl ester, which purity was more than 95% in HPLC (210 nm) (Fig. 1), was isolated as previously reported.14 Ibuprofen, Dulbecco's modified Eagle's medium (DMEM) and lipopolysaccharide (LPS, Escherichia coli 055:B5) were purchased from Sigma Chemical Co. (St. Louis, MO, USA) and morphine was acquired from Myungmoon Pharm. Co. (Gyunggi-Do, Korea). Fetal bovine serum (FBS) was purchased from JRS Biosciences (Lenexa, KS, USA).
Male ICR mice (5 weeks old) were acquired from Samtako, Inc. (Gyunggi-Do, Korea) and housed in an environmentally controlled room at 22 ± 2 ℃ under a relative humidity of 55 ± 5% and a 12 h light/dark cycle while provided with food and water ad libitum. The procedures involving experimental animals complied with the Animal Care Guidelines of the National Institutes of Health and the Animal Ethics Committee of Chungbuk National University.
Acetic acid (2%) was intraperitoneally injected into mice 30 min after the administration of vehicle (normal saline, p.o.), I. latifolia (50, 100 and 200 mg/kg, p.o.) or ibuprofen (100 mg/kg, p.o.). Each mouse was then placed in an individual clear plastic observation chamber, and the total number of writhes made by each mouse was counted between 5 and 25 min after acetic acid administration. For scoring purposes, a writhe was indicated by stretching of the abdomen with simultaneous stretching of at least one hind limb.18
Formalin-induced nociception was measured as previously described, with slight modification.19 Briefly, mice received 20 µL of 5% formalin in saline via an intraplantar injection of the right hind paw 30 min after the administration of vehicle (normal saline, p.o.), I. latifolia (50, 100 and 200 mg/kg, p.o.) or ibuprofen (100 mg/kg, p.o.). Following formalin injection, mice were placed in a transparent acrylic cage and the time in seconds spent licking the injected paw was recorded with a chronometer for both the early neurogenic phase (0 – 5 min) and late inflammatory phase (15 – 30 min) of this model. These behaviors were considered nociceptive responses.
The test was conducted using the Hot Plate Analgesia Meter (IITC Life Science, Woodland Hills, CA, USA). The hot plate temperature was kept constant at 55 ± 1 ℃. Mice were gently placed on the hot plate and jumping with all four paws was considered nociception. Only mice showing a pretreatment reaction time ≤3 s were selected. Additionally, mice were only kept on the hot surface for 10 s (cut-off time) to avoid any thermal injury. The reaction time of jumping was measured before and repeatedly at different times (15, 30, 45, 60 and 90 min) after administration of vehicle (normal saline, p.o.), I. latifolia (50, 100, and 200 mg/kg, p.o.), or morphine (5 mg/kg, s.c.).20
To measure the tail-flick latency, mice were gently held with one hand with the tail positioned in the Tail-Flick Analgesia Meter (IITC Inc. Life Science, Woodland Hills, CA, USA) and the tail-flick response was elicited by applying radiant heat to the dorsal surface of the tail.21 The intensity of radiant heat was adjusted so that the animal flicked its tail within 3 to 5 s and only mice showing a pretreatment reaction time ≤3 s were selected. The cut-off time for the tail flick test was 10 s to minimize any thermal injury. Immediately after basal latency assessment, vehicle (normal saline, p.o.), I. latifolia (50, 100, and 200 mg/kg, p.o.), or morphine (5 mg/kg, s.c.) was administered and the reaction time was again measured at 15, 30, 45, 60, 90, and 120 min after the administration.
The RAW 264.7 murine macrophage cell line was obtained from the Korean Cell Line Bank. Cells were grown at 37 ℃ in DMEM supplemented with 10% FBS, penicillin (100 units/mL) and streptomycin sulfate (100 µg/mL) in a 5% CO2 incubator.22 Cells were incubated with I. latifolia (10, 50 and 100 µg/mL) and 3,5-diCQA methyl ester (0.1, 1 and 5 µM) 1 h before treatment with 1 µg/mL LPS, which was solubilized and diluted fresh with serum-free growth medium for every experiment. I. latifolia and 3,5-diCQA methyl ester were dissolved in DMSO, diluted in serum-free DMEM and added to the medium in serial dilutions (the final DMSO concentration in all assays did not exceed 0.1%).
The nitrite accumulated in the culture medium was measured as an indicator of NO production based on the Griess reaction. Briefly, the supernatant (50 µL) of culture media was harvested 24 h after treatment with LPS, mixed with an equal volume of Griess reagent (0.1% (w/v) N-naphthylethylenediamine-dihydrochloride and 1% (w/v) sulfanilamide in 5% (v/v) phosphoric acid) in a 96-well plate and incubated at room temperature for 15 min. The absorbance at 540 nm was then measured using a microplate reader (ELX808; Bio-Tek, Winooski, VT, USA). Serum-free culture medium was used as the blank in all experiments. The amount of nitrite in the samples was obtained by comparison to a NaNO2 serial dilution standard curve and the nitrite production was measured.23
After 12 h of treatment with LPS, total cellular RNA was isolated using a RNA isolation kit (Zymo Research, Irvine, CA, USA). Reverse transcription was then performed with a Reverse Transcription System (Promega, Madison, WI, USA). Briefly, total RNA (1 µg) was reverse-transcribed into cDNA using commercially available cDNA synthesis kits (Promega, WI, USA), 20 µM oligo dT primer, 1 mM deoxyribonucleotide triphosphates (dNTP), and 1 U RNAse inhibitor. After this reaction cocktail was incubated at 70 ℃ for 5 min, 25 ℃ for 5 min, and 37 ℃ for 60 min in series, reverse transcriptase was inactivated by heating at 70 ℃ for 10 min. Samples were then stored at −70 ℃ until used. Polymerase chain reaction (PCR) was performed in reaction buffer containing cDNA, 1 U Taq DNA polymerase (Promega, WI, USA), 3′- and 5′-primer (50 µM each), 10× PCR buffer, and 100 µM dNTP in TBE buffer (8.9 mM Tris-base, 8.9 mM boric acid and 50 mM EDTA, pH 8.2).22 The PCR primers used to detect TNF-α, IL-1β, IL-6, iNOS, and COX-2 (using GAPDH as an internal standard) were purchased from Macrogen Inc. (Seoul, Korea). Reaction conditions consisted of initial denaturation at 94 ℃ for 5 min, followed by and 30 cycles of 30 s at 94 ℃, 30 s at 58 ℃ – 60 ℃ (58 ℃ for IL-6, iNOS and COX-2; 60 ℃ for TNF-α and IL-1β), and 30 s at 72 ℃, and then 10 min at 72 ℃ for final extension before holding at 4 ℃. Each sample was analyzed by electrophoresis in 1.5% agarose gel in the presence of 5 ng/mL ethidium bromide and visualized under ultraviolet light.
Anti-nociceptive activity was evaluated by an acetic acid writhing test, formalin test, and thermal stimuli (hot plate and tail-flick) tests. The effect of an ethanol extract of I. latifolia on acetic acid-induced writhing responses in mice is shown in Fig. 2. The writhing number of the vehicle treated group was 116.5 ± 4.8 after injection of 2% acetic acid (i.p.); however, pretreatment of I. latifolia (50, 100 and 200 mg/kg, p.o.) significantly inhibited acetic acid-induced writhing to 86.7 ± 6.6, 65.5 ± 4.5, and 59.9 ± 3.6 occurrences, respectively. Ibuprofen (100 mg/kg) also induced an inhibitory effect on acetic acid-induced writhing to only 13.2 ± 2.6 instances (Fig. 2).
I. latifolia (50, 100, and 200 mg/kg) induced significant inhibition of the second inflammatory phase (15 – 30 min) of formalin-induced licking, resulting in licking times of 305.8 ± 8.2, 222.0 ± 16.5, and 178.9 ± 10.7 s, respectively, whereas that of the vehicle-control was 400.5 ± 14.7 s. A similar inhibitory effect toward inflammatory nociception was induced by ibuprofen (100 mg/kg). However, neither I. latifolia nor ibuprofen inhibited the first phase (0 – 5 min) of neurogenic nociception (Fig. 3).
Measurement of escape latency was repeated at the indicated times after treatment with drugs in the hot plate and tail flick tests (Fig. 4). I. latifolia (50, 100, and 200 mg/kg) did not induce an inhibitory effect on thermal stimuli-induced nociceptive responses in the hot plate test (Fig. 4A) or the tail flick test (Fig. 4B). In contrast, morphine (5 mg/kg, s.c.) remarkably inhibited thermally induced nociception.
When RAW 264.7 cells were exposed to 1 µg/mL LPS for 24 h, NO production increased to 72.2 ± 0.6 µM, while it was only 0.5 ± 0.1 µM in the untreated control. However, I. latifolia (10, 50 and 100 µg/mL) significantly blocked the LPS-induced increase in NO production (16.1 ± 2.6 µM for 100 µg/mL) (Fig. 5). Among the seven isolated CQAs, only 3,5-diCQA methyl ester (1 and 5 µM) showed a significant inhibitory effect against LPS-induced NO production (14.9 ± 1.5 µM for 5 µM) (Fig. 5).
Treatment with 1 µg/mL LPS for 12 h significantly increased the mRNA expression level of iNOS and COX-2. However, pretreatment with I. latifolia (50 and 100 µg/mL) and 3,5-diCQA methyl ester (1 and 5 µM) significantly reduced the expression levels of iNOS and COX-2 mRNA that were induced by LPS (Fig. 6 A and B).
Treatment with 1 µg/mL LPS for 12 h significantly increased the mRNA expression level of cytokines, including TNF-α, IL-1β and IL-6. Pretreatment with I. latifolia (50 and 100 µg/mL) and 3,5-diCQA methyl ester (1 and 5 µM) significantly reduced the expression of IL-1β, and IL-6 mRNA; however, LPS-induced increase in TNF-α mRNA expression was not inhibited by treatment with I. latifolia or 3,5-diCQA methyl ester (Fig. 7A and B).
Acetic acid-induced abdominal constriction response has been described as a typical experimental model for the study of inflammatory pain and used to evaluate analgesics or anti-inflammatory drugs.24 The local irritation provoked by intraperitoneal injection of chemical substances, such as phenyl quinone or acetic acid, triggers liberation of a variety of mediators including bradykinin, substance P and PGs and cytokines such as TNF-α, IL-1β, and IL-8.25 These mediators activate chemosensitive nociceptors that contribute to the development of inflammatory pain, which is known to be sensitive to NSAIDs like ibuprofen or indomethacin. In this study, I. latifolia as well as ibuprofen used as a reference NSAID significantly inhibited acetic acid-induced writhes in mice, suggesting a mechanism involved in anti-nociceptive effect by the inhibition of mediator release during the inflammatory process.
The formalin-induced algesia test is a well-established and frequently used model to study mechanisms of pain and evaluate the analgesic action of various endogenous and exogenous substances. Pain produced by the hind paw injection of formalin results from persistent tissue damage and therefore more closely resembles clinical pain.26 Intraplantar injection of formalin produces a reproducible syndrome of nociceptive behaviors, which appear in two distinct phases. The first phase begins at the time of injection and lasts for about 10 min. The subsequent (second) phase starts at 10 min post-injection and lasts for about 50 min. The first phase is thought to result from a direct chemical activation of nociceptive afferent fibers, while the second is believed to be mediated by inflammatory pain triggered by a combination of stimuli, including inflammation of the peripheral tissues and mechanisms of central sensitization.27 Notably, the first phase nociception produced by formalin is quite resistant to the NSAIDs, such as ibuprofen (results presented here), acetyl salicylic acid, and indomethacin. However, these drugs can attenuate the second phase of formalin-induced licking.28 Moreover, morphine is able to inhibit both phases of the formalin test.29 Intraplantar injection of formalin generated a characteristic biphasic licking response in the present study. While single administration of I. latifolia was not able to decrease the licking time on the first phase, the second phase was significantly inhibited. Ibuprofen also showed an analgesic effect only during the second phase. These results suggest that I. latifolia may act via inhibition of inflammation.
To further elucidate a possible central involvement in the anti-nociceptive effect of I. latifolia, tail flick and hot plate tests were conducted. These tests using thermal stimuli are commonly employed to investigate the anti-nociceptive activity mediated by agents targeting central mechanisms, such as morphine, but peripheral compounds are inactive on this kind of painful stimulus.30 In the present study, morphine increased the latencies of the tail flick and hot plate responses, but I. latifolia failed to induce an anti-nociceptive effect in either test. These results provide evidence that the anti-nociceptive action of I. latifolia is more like that of NSAIDs, occurring via peripheral anti-nociceptive action, than that of morphine, which exerts a central anti-nociceptive effect. Taken together, these findings suggest that I. latifolia could inhibit inflammatory pain, possibly through the inhibition of PGs synthesis by COX, but not neurogenic pain.
To elucidate the anti-inflammatory mechanism, the protective properties of I. latifolia against LPS-induced inflammatory damage were investigated in the RAW 264.7 cell line, a mouse leukemic monocyte macrophage cell line. Inflammatory stimulation of RAW 264.7 cells with LPS produces a large amount of inflammatory mediators, reactive oxygen species and reactive nitrogen species.31 Thus, the RAW 264.7 cell line provides an excellent model for investigating anti-inflammatory mechanisms and screening anti-inflammatory drugs. Treatment of RAW 264.7 cells with LPS induced release of NO in the current study. Nitric oxide, which is derived from L-arginine by iNOS.32 is a free radical that plays a key role in cell death and inflammatory cascades.33 Increases in the expression of iNOS can trigger overproduction of NO and high levels of released NO have a cytotoxic effect in many types of inflammation.34 Expression of iNOS mRNA by LPS in RAW 264.7 cells was also increased in this study. I. latifolia significantly inhibited NO production and iNOS mRNA expression in LPS-treated cells, suggesting that I. latifolia could inhibit NO production by suppressing iNOS expression. In a previous report, we isolated seven CQAs from I. latifolia and demonstrated that three CQAs, 3,4-diCQA, 3,5-diCQA and 3,5-diCQA methyl ester, showed neuroprotective effects against hypoxic and excitotoxic neuronal cell death.14 However, only 3,5-diCQA methyl ester significantly inhibited iNOS mRNA expression and NO production in the current study.
The involvement of COX-2, which catalyzes PGs production, in the progression and manifestation of inflammatory conditions is well established.35 In the present study, LPS treatment of RAW 264.7 cells increased mRNA expression of COX-2, while I. latifolia and 3,5-diCQA methyl ester inhibited this COX-2 mRNA expression. The inhibition of COX-2 expression by I. latifolia might suppress the production of PG, which would contribute to the anti-nociceptive effect of this plant against inflammatory pain induced by chemical stimuli.
During inflammatory responses, activated macrophages cause tissue damage via excessive release of proinflammatory cytokines, such as TNF-α, IL-1β, and IL-6, which is responsible for enhancing COX-2 and iNOS expression.36 IL-1β is synthesized in activated macrophages and triggers cell apoptosis,37 while IL-6 is a secondary cytokine stimulated by primary cytokines including TNF-α and IL-1. In the present study, LPS significantly increased mRNA expression of TNF-α, IL-1β, and IL-6 in RAW 264.7 cells, while I. latifolia and 3,5-diCQA methyl ester significantly inhibited expression of IL-1β and IL-6 mRNA. The inhibition of IL-1β may result in the inhibition of the secondary cytokine, IL-6. However, I. latifolia and 3,5-diCQA methyl ester failed to reduce mRNA expression of TNF-α, suggesting that the inhibition of iNOS or COX-2 expression by I. latifolia and 3,5-diCQA methyl ester is associated with suppression of the release of IL-1β and IL-6 release, and less mediated by TNF-α formation. Further study is needed to elucidate the underlying mechanisms responsible for these effects.
In the present study, I. latifolia showed an anti-nociceptive effect against chemical stimuli-induced pain in mice. Additionally, LPS-induced NO production and increases in mRNA expression of the inflammatory mediators, IL-1β, IL-6, iNOS, and COX-2, were significantly inhibited by I. latifolia and 3,5-diCQA methyl ester in RAW 264.7 cells. These results suggest that I. latifolia exerts anti-nociceptive action against inflammation-mediated pain by inhibiting NO and PG production through inhibition of the expression of iNOS and COX-2. Inhibition of IL-1β and IL-6 may also result in inhibition of iNOS and COX-2 expression. The anti-nociceptive and anti-inflammatory effects of I. latifolia could be, at least in part, attributable to its active compound, 3,5-diCQA methyl ester. Studies have demonstrated that I. latifolia exerts anti-inflammatory effects through in vitro experiments.16 However, the present study demonstrated for the first time that I. latifolia induces significant peripheral anti-nociceptive effects in inflammation-mediated nociception models using experimental animals and demonstrated the mechanism of anti-inflammation using macrophage cell lines. This study is the first to demonstrate that 3,5-DiCQA methyl ester exerts anti-inflammatory activity via the inhibition of cytokines and COX-2 and iNOS. We previously reported neuroprotective effects of I. latifolia on ischemic brain injury and memory impairment,1317 which might be attributable to its anti-inflammatory activity. In summary, the current study provides a quantitative basis for explaining the effects of the traditional folk-medicine I. latifolia when used to treat pain and inflammation.
Figures and Tables
Fig. 1
HPLC chromatogram of 3,5-diCQA methyl ester. HPLC analysis was performed on an Waters system (2695 Separation module with a 2996 photodiode array detector, detecting wavelength = 210 nm) and a YMC J'sphere ODS-H80 column (4 µm, 150 × 4.6 mm I.D.), using the mixed solvent system MeCN-H2O (20:80 to 30:70, 0–25 min) at a flow rate of 1.0 mL/min.
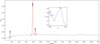
Fig. 2
Inhibitory effect of I. latifolia against acetic acid-induced writhing response in mice. The total number of writhes of each mouse was counted for 20 min, between 5 and 25 min after acetic acid injection. Stretching of the abdomen with simultaneous stretching of at least one hind limb was considered a writhe. Values are expressed as the means ± S.E.M. (n=10). ** P<0.01 vs. vehicle.
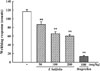
Fig. 3
Inhibitory effect of I. latifolia against formalin-induced paw licking in mice. Licking time of the injected paw at 0 – 5min (phase 1) and 15 – 30min (phase 2) after formalin injection was measured in seconds. Values are expressed as the means ± S.E.M. (n=10). ** P<0.01 vs. vehicle.
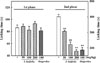
Fig. 4
Inhibitory effect of I. latifolia against thermal pain in mice subjected to a hot plate test (A) and tail flick test (B). Pre-values were determined before administration of vehicle, I. latifolia or morphine and post latencies were obtained at 15, 30, 45, 60, 90, and 120 min after administration. Values are expressed as the means ± S.E.M. (n=10). ** P<0.01 vs. vehicle.
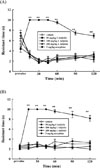
Fig. 5
Inhibitory effects of I. latifolia and 3,5-diCQA methyl ester against LPS-induced NO production in RAW 264.7 cell line. The concentration of in culture media was measured 24 h after treatment with LPS. Values are expressed as the means ± S.E.M. of data obtained from at least ten independent experiments. ## P<0.01 vs. control, ** P<0.01 vs. 1 µg/mL LPS.
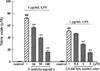
Fig. 6
Inhibitory effects of I. latifolia and 3,5-diCQA methyl ester against LPS-induced increase in mRNA expression level of iNOS and COX-2 in the RAW 264.7 cell line. (A) Representative RT-PCR analysis of mRNA. (B) Bar graphs of relative ratio of iNOS and COX-2 mRNA compared to GAPDH. Values are expressed as the means ± S.E.M. of data obtained from at least five independent experiments. * P<0.05 and ** P<0.01 vs. 1 µg/mL LPS.
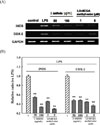
Fig. 7
Inhibitory effects of I. latifolia and 3,5-diCQA methyl ester against LPS-induced increase in mRNA expression level of cytokines in the RAW 264.7 cell line. (A) Representative RT-PCR analysis of mRNA. (B) Bar graphs of relative ratio of cytokines mRNA compared to GAPDH. Values are expressed as the means ± S.E.M. of data obtained from at least five independent experiments. * P<0.05 and ** P<0.01 vs. 1 µg/mL LPS.
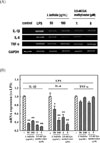
References
5. Crofford LJ, Lipsky PE, Brooks P, Abramson SB, Simon LS, van de Putte LB. Arthritis Rheum. 2000; 43:4–13.

7. Szabó C, Thiemermann C, Wu CC, Perretti M, Vane JR. Proc Natl Acad Sci U S A. 1994; 91:271–275.
16. Negishi O, Negishi Y, Yamaguchi F, Sugahara T. J Agric Food Chem. 2004; 52:5513–5518.
20. Matheus ME, Berrondo LF, Vieitas EC, Menezes FS, Fernandes PD. J Ethnopharmacol. 2005; 102:377–381.

23. D'Amour FE, Smith DL. J Pharmacol Exp Ther. 1941; 72:74–79.
26. Vinegar R, Truax JF, Selph JL, Johnston PR. Vane JR, Ferreira SH, editors. In Anti-inflammatory Drugs. Germany: Springer;1979. p. 208–222.
27. de Oliveira AM, de Araújo AF, Lyra Lemos RP, Conserva LM, de Souza Ferro JN, Barreto E. J Nat Med. 2015; 69:232–240.

34. Sweet MJ, Hume DA. J Leukoc Biol. 1996; 60:8–26.
36. Saravanan R, Viswanathan P, Pugalendi KV. Life Sci. 2006; 78:713–718.
39. Anderson GD, Hauser SD, McGarity KL, Bremer ME, Isakson PC, Gregory SA. J Clin Invest. 1996; 97:2672–2679.

