Abstract
Figures and Tables
Fig. 2
Isolation scheme of compounds 1 – 6 from the aqueous extract left after partitioning of the total MeOH extract using pet.ether, CH2Cl2 and EtOAc, respectively.
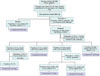
Fig. 4
Impact of treatments with EtOAc extracts and compounds (1, 6, 7), isolated from Shinus terbenthifolius leaves, on egg hatchability of root-knot nematode Meloidogyne incognita under laboratory conditions.
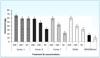
Fig. 5
Impact of treatments with EtOAc extracts and compounds (1, 6, 7), isolated from Shinus terbenthifolius leaves, against larvae of root-knot nematode Meloidogyne incognita under laboratory conditions.
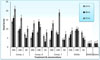
Fig. 6
Impact of compounds 1, isolated from Shinus terbenthifolius leaves, on plant growth response of tomato var. 162 infected with Meloidogyne incognita under greenhouse conditions (27 ± 3℃).
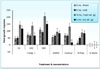
Fig. 7
Impact of compound 1 isolated from Schinus terbenthifolius leaves, on the reproduction of Meloidogyne incognita infecting tomato var. 162 grown under greenhouse conditions at 27 ± 3℃. Reproduction factor (RF), Root gall index (RGI) or egg-masses index (EI) were determined according to the scale given by Taylor and Sasser (1978).
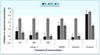
Table 1
Impact of treatments with n-hexane, EtOAc extracts and compounds (1 – 9), isolated from Shinus terbenthifolius leaves, against eggs and larvae of root-knot nematode Meloidogyne incognita under laboratory conditions.
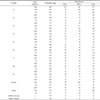
Table 2
Impact of treatment with n-hexane, EtOAc extracts and compounds 1, 6 and 7, isolated from Shinus terbenthifolius leaves, on plant growth response of tomato var. 162 infected with Meloidogyne incognita under greenhouse conditions (27 ± 3 ℃).
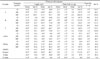
Table 3
Impact of treatments with n-hexane, EtOAc extracts and compounds 1, 6 and 7, isolated from Schinus terbenthifolius leaves, on the development and reproduction of Meloidogyne incognita infecting tomato var. 162 grown under greenhouse conditions at 27 ± 3℃.
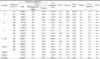
*Each value presented the mean of three replicates.
N = M. incognita (1000 larva/plant).
Means in each column followed by the same letter (s) did not differ at P ≤ 0.05 according to Duncan's multiple range test.
** Reproduction factor (RF) = Nematode population in soil + No. of developmental stages + No. of females + No. of eggmasses.
No. of eggs inocula, * ** Root gall index (RGI) or egg-masses index (EI) was determined according to the scale given by Taylor and Sasser (1978) as follows : 0 = no galls or eggmasses, 1 = 1–2 galls or eggmasses , 2 = 3–10 galls eggmasses, 3 = 11–30 galls or eggmasses, 4 = 31–100 galls or eggmasses and 5 = more than 100 galls or eggmas
Table 4
Impact of treatment with n-hexane, EtOAc extracts and compounds 1, 6 and 7, isolated from Schinus terbinthifolius leaves, on the biochemical activities of the leaves of tomato var. 162 infected with Meloidogyne incognita and grown under greenhouse conditions at 27 ± 3℃.
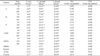
*Each value presented the mean of three replicates. T. carb; total carbohydrates, C. proteins; crude proteins, T. phenols; total phenols, PO; peroxidase, PPO; polyphenol oxidase. N = M. incognita (1000 larvae / plant). N-free: Plants free of M. incognita. Means are followed by the same letter (s) did not differ at P ≤ 0.05 according to Duncan's multiple range test.