Abstract
Background and Purpose
Although sleep disturbances are common and considered a major burden for patients with Alzheimer's disease (AD), the fundamental mechanisms underlying the development and maintenance of sleep disturbance in AD patients have yet to be elucidated. The aim of this study was to examine the correlation between regional cerebral blood flow (rCBF) and sleep disturbance in AD patients using technetium-99m hexamethylpropylene amine oxime single-photon emission computed tomography (SPECT).
Methods
A total of 140 AD patients were included in this cross-sectional study. Seventy patients were assigned to the AD with sleep loss (SL) group and the rest were assigned to the AD without SL group. SL was measured using the sleep subscale of the Neuropsychiatric Inventory. A whole-brain voxel-wise analysis of brain SPECT data was conducted to compare the rCBF between the two groups.
Results
The two groups did not differ in demographic characteristics, severity of dementia, general cognitive function, and neuropsychiatric symptoms, with the exception of sleep disturbances. The SPECT imaging analysis displayed decreased perfusion in the bilateral inferior frontal gyrus, bilateral temporal pole, and right precentral gyrus in the AD patients with SL group compared with the AD patients without SL group. It also revealed increased perfusion in the right precuneus, right occipital pole, and left middle occipital gyrus in the AD with SL group compared with the AD without SL group.
Conclusions
The AD patients who experienced sleep disturbance had notably decreased perfusion in the frontal and temporal lobes and increased rCBF in the parietal and occipital regions. The findings of this study suggest that functional alterations in these brain areas may be the underlying neural correlates of sleep disturbance in AD patients.
Sleep disturbances are common in both individuals who are aging normally and patients with Alzheimer's disease (AD). It has been reported that approximately 19–44% of AD patients are affected by sleep disturbances.123 Disrupted sleep in AD patients is a significant physical and psychological burden to not only patients but also caregivers and often cited as a major cause for institutionalizing dementia patients.456
While sleep-related problems such as daytime sleepiness and fragmented nighttime sleep occur with normal aging, these problems occur more frequently and tend to be more severe in patients with AD.7 Previous studies have demonstrated that sleep disturbances in AD patients are associated with changes in sleep architecture including sleep phase delay,8 increased nighttime awakenings,9 decreased slow-wave sleep and rapid eye movement sleep,910 and disrupted stage 2 sleep.11 Moreover, sleep disturbances were also associated with increased memory and functional impairment and more rapid cognitive decline in patients with AD.31213
The fundamental mechanisms underlying the development and maintenance of sleep disturbance in AD patients are complex and poorly understood. It may be attributed to AD-related damage in the brain regions or neuronal pathways that are involved in sleep regulation, thereby, exacerbating age-related sleep changes.7 In particular, it has been suggested that brainstem areas and pathways responsible for sleep-wake cycles and cortical areas that generate slow-wave activity during sleep are affected by degenerative changes associated with AD.14 Furthermore, these suspected mechanisms interact with and are influenced by a variety of physical, psychiatric, demographic, and environmental factors.7
While a large number of neuroimaging studies have been performed on AD patients, only a small number of studies have employed neuroimaging to investigate the neural mechanisms underlying sleep disturbance in AD patients. Notably, little attention has been devoted to the regional cerebral blood flow (rCBF), which is considered a measure of neuronal activity and cerebral metabolism. To the best of our knowledge, only one study with a modest sample size has examined rCBF changes in AD patients who experience sleep disturbance.15 Thus, the purpose of this study was to examine the correlation between regional cerebral perfusion and sleep loss (SL) in AD patients using single-photon emission computed tomography (SPECT).
One hundred forty patients with AD (70 patients with SL, 70 patients without SL) were recruited at Incheon St. Mary's Hospital (Incheon, Korea). Using the Neuropsychiatric Inventory (NPI),16 patients who scored 0 on the sleep disorders (nightmare behaviors) domain were assigned to the “AD without SL” group and those who scored 1 or above on the sleep disorders domain were assigned to the “AD with SL” group. The diagnosis for probable AD was made according to the criteria from the Diagnostic and Statistical Manual of Mental Disorders-IV17 and the National Institute of Neurological and Communicative Disorders and Stroke-AD Related Disorders Association.18 Patients with a history of head trauma, stroke, epilepsy, mixed or vascular dementia, radiological findings on magnetic resonance imaging, and other neurological or psychiatric disorders were excluded from the study.
This study was approved by the Institutional Review Board of Incheon St. Mary's Hospital, and all participants provided written informed consent.
Experienced neurologists conducted medical history and physical examinations. Global cognitive performance and neuropsychiatric symptoms were assessed with the Mini-Mental State Examination (MMSE)19 and NPI,16 respectively. Global severity of dementia was assessed using the Clinical Dementia Rating (CDR) and CDR-Sum of Boxes (CDR-SOB).2021
The SPECT images were acquired using a dual-head gamma camera (Discovery NM 640; GE Healthcare, Milwaukee, WI, USA). Patients were administered 1110 MBq of technetium-99m hexamethylpropylene amine oxime (99mTc-HMPAO) intravenously 40 minutes before the scan in a quiet, dimly lit room. Patients were in the supine resting state with their eyes open during the scan. The images were reconstructed as 128×128 matrices with a voxel size of 3.9×3.9×3.9 mm3 using a filtered back projection.
Data analyses were performed using Statistical Parametric Mapping 12 (SPM; Wellcome Department of Cognitive Neurology, Institute of Neurology, London, UK). All SPECT images were spatially normalized to the SPM SPECT template (Montreal Neurological Institute, McGill University, Montreal, Canada) and resliced with a voxel size of 2×2×2 mm3. The normalized images were smoothed with an isotropic Gaussian kernel (16 mm full-width at half-maximum).
A voxel-wise two-sample t-test analysis using SPM12 with age and sex as covariates was performed to examine regional differences in perfusion between the two groups. The perfusion values were scaled to those of the cerebellum using the Automated Anatomical Labeling atlas.222324 A voxel-level threshold of p less than 0.005 with a cluster size of 100 or more contiguous voxels was applied.
To compare the demographic and clinical data between the groups, independent t-tests and chi-square tests were used for continuous and categorical variables, respectively. All statistical analyses were conducted with Stata version 13.1 (StataCorp., College Station, TX, USA). A two-tailed p-value of less than 0.05 was considered statistically significant.
A total of 140 patients with AD were included in the study. The AD with SL group and the AD without SL group each consisted of 70 patients. Table 1 summarizes the demographic and clinical characteristics of the patients. The mean age, sex distribution, and education level did not differ significantly between the AD with SL group and the AD without SL group. Moreover, there were no significant differences between the two groups in the severity of the disease and cognitive function, which were assessed by the MMSE, CDR, and CDR-SOB. The neuropsychiatric problems assessed by the NPI also did not differ between the groups with the exception of the sleep disorders domain (p<0.001).
The SPECT imaging analysis exhibited decreased rCBF in the bilateral inferior frontal gyrus (p<0.001), bilateral temporal pole (p<0.001), and right precentral gyrus (p<0.001) in the AD patients with SL group compared with the AD patients without SL group (Table 2, Fig. 1). It also revealed increased rCBF in the right precuneus (p<0.001), right occipital pole (p=0.001), and left middle occipital gyrus (p=0.002) in the AD with SL group compared with the AD without SL group (Table 2, Fig. 1).
In this study, we investigated the changes in cerebral perfusion among AD patients who experience sleep disturbance using SPECT. The results of this study demonstrated that cerebral perfusion decreased in the temporal and frontal lobes and increased in the precuneus and occipital lobe in the AD patients who suffer from SL compared with AD patients who do not.
In line with the results of this study, functional deficits of the frontal and temporal cortices have been observed in previous neuroimaging studies of healthy individuals with compromised sleep quality. A study using positron emission tomography (PET) found decreases in metabolism in the frontal lobe including the inferior frontal gyrus and temporal regions after sleep deprivation.25 Similarly, other PET studies displayed a reduction of glucose uptake in the prefrontal cortex26 and changes in the rCBF pattern in the prefrontal and temporal areas during a verb generation task following sleep deprivation.27 Moreover, the electroencephalography (EEG) recordings of individuals with sleep deprivation exhibited increases in delta and theta activities in the frontal areas compared with the parietal and occipital areas, which indicated their vulnerability to the effects of sleep loss and their role in homeostatic function.28 Additionally, sleep problems were associated with impaired performance on tasks involving the frontal lobe including executive attention, working memory, and higher cognitive functions.29
Increased perfusion in the occipital areas in AD patients with SL was also found in this study. These findings are supported by previous PET studies that demonstrated increases in regional metabolism in the occipital cortex in individuals with sleep deprivation.2526 Since it has been suggested that the occipital areas are less susceptible to sleep loss than the frontal areas,30 decreased activity in the frontal lobe and increased activity in the occipital lobe may be due to the compensatory mechanisms of the occipital areas for frontal lobe deficits.25
On the other hand, our findings were not consistent with those from a previous SPECT study that demonstrated increased perfusion in the middle frontal gyrus in AD patients with SL.15 The inconsistencies may be explained in part by several differences between the studies including the study design, sample size, selection criteria, and patient samples.
The current study has limitations that merit mention. First, objective sleep measures such as a polysomnography were not used for group assignment in the study. Moreover, due to the cross-sectional design of the study, it is not possible to establish causal relationships.
In summary, the decreased perfusion in the frontal and temporal areas and increased rCBF in the parietal and occipital regions were evidenced in AD patients who suffer from sleep loss. The findings of this study suggest that functional alterations in these brain areas may be the underlying neural correlates of sleep disturbance in AD patients. Future studies with larger sample sizes and longitudinal designs are needed to confirm and further elucidate the effects of sleep disturbance on cerebral perfusion in AD patients.
Figures and Tables
Fig. 1
Differences in the regional cerebral blood flow between patients with AD with SL and without SL. At each voxel, increases or decreases in the regional brain perfusion in the AD with SL group appear in red-yellow or blue-green, respectively. The images are shown in a neurological convention and color bars represent voxel-level t-values. AD: Alzheimer's disease, SL: sleep loss.
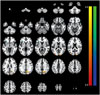
Acknowledgements
This research was supported by the Brain Research Program through the National Research Foundation of Korea (NRF) funded by the Ministry of Science and ICT (NRF-2015M3C7A1064832).
References
1. Carpenter BD, Strauss M, Patterson MB. Sleep disturbances in community-dwelling patients with Alzheimer's disease. Clinical Gerontologist. 1996; 16:35–49.


2. Ritchie K. Behavioral disturbances of dementia in ambulatory care settings. Int Psychogeriatr. 1996; 8:Suppl 3. 439–442.


3. McCurry SM, Logsdon RG, Teri L, Gibbons LE, Kukull WA, Bowen JD, et al. Characteristics of sleep disturbance in community-dwelling Alzheimer's disease patients. J Geriatr Psychiatry Neurol. 1999; 12:53–59.


4. Pollak CP, Perlick D. Sleep problems and institutionalization of the elderly. J Geriatr Psychiatry Neurol. 1991; 4:204–210.


5. Hope T, Keene J, Gedling K, Fairburn CG, Jacoby R. Predictors of institutionalization for people with dementia living at home with a carer. Int J Geriatr Psychiatry. 1998; 13:682–690.


6. Chenier MC. Review and analysis of caregiver burden and nursing home placement. Geriatr Nurs. 1997; 18:121–126.


7. McCurry SM, Reynolds CF, Ancoli-Israel S, Teri L, Vitiello MV. Treatment of sleep disturbance in Alzheimer's disease. Sleep Med Rev. 2000; 4:603–628.


8. Harper DG, Volicer L, Stopa EG, McKee AC, Nitta M, Satlin A. Disturbance of endogenous circadian rhythm in aging and Alzheimer disease. Am J Geriatr Psychiatry. 2005; 13:359–368.


9. Vitiello MV, Prinz PN, Williams DE, Frommlet MS, Ries RK. Sleep disturbances in patients with mild-stage Alzheimer's disease. J Gerontol. 1990; 45:M131–M138.


10. Prinz PN, Peskind ER, Vitaliano PP, Raskind MA, Eisdorfer C, Zemcuznikov N, et al. Changes in the sleep and waking EEGs of nondemented and demented elderly subjects. J Am Geriatr Soc. 1982; 30:86–93.


11. Allen SR, Stähelin HB, Seiler WO, Spiegel R. EEG and sleep in aged hospitalized patients with senile dementia: 24-h recordings. Experientia. 1983; 39:249–255.


12. Moe KE, Vitiello MV, Larsen LH, Prinz PN. Sleep/wake patterns in Alzheimer's disease: relationships with cognition and function. J Sleep Res. 1995; 4:15–20.


13. Mortimer JA, Ebbitt B, Jun SP, Finch MD. Predictors of cognitive and functional progression in patients with probable Alzheimer's disease. Neurology. 1992; 42:1689–1696.


14. Vitiello M, Prinz P. Sleep disturbances in the elderly. In : Albert ML, Knoefel JE, editors. Clinical Neurology of Aging. 2nd ed. New York: Oxford University Press, Inc.;1994. p. 637–650.
15. Ismail Z, Herrmann N, Francis PL, Rothenburg LS, Lobaugh NJ, Leibovitch FS, et al. A SPECT study of sleep disturbance and Alzheimer's disease. Dement Geriatr Cogn Disord. 2009; 27:254–259.


16. Choi SH, Na DL, Kwon HM, Yoon SJ, Jeong JH, Ha CK. The Korean version of the neuropsychiatric inventory: a scoring tool for neuropsychiatric disturbance in dementia patients. J Korean Med Sci. 2000; 15:609–615.


17. American Psychiatric Association. Task Force on DSM-IV. Diagnostic and Statistical Manual of Mental Disorders: DSM-IV. 4th ed. Washington, DC: American Psychiatric Association;1994.
18. McKhann G, Drachman D, Folstein M, Katzman R, Price D, Stadlan EM. Clinical diagnosis of Alzheimer's disease: report of the NINCDSADRDA Work Group under the auspices of Department of Health and Human Services Task Force on Alzheimer's Disease. Neurology. 1984; 34:939–944.


19. Kang Y, Na DL, Hahn SH. A validity study on the Korean mini-mental state examination (K-MMSE) in dementia patients. J Korean Neurol Assoc. 1997; 15:300–308.
20. Morris JC, Heyman A, Mohs RC, Hughes JP, van Belle G, Fillenbaum G, et al. The Consortium to Establish a Registry for Alzheimer’s Disease (CERAD). Part I. Clinical and neuropsychological assessment of Alzheimer's disease. Neurology. 1989; 39:1159–1165.
21. Choi SH, Na DL, Lee BH, Hahm DS, Jeong JH, Jeong Y, et al. The validity of the Korean version of global deterioration scale. J Korean Neurol Assoc. 2002; 20:612–617.
22. Tzourio-Mazoyer N, Landeau B, Papathanassiou D, Crivello F, Etard O, Delcroix N, et al. Automated anatomical labeling of activations in SPM using a macroscopic anatomical parcellation of the MNI MRI single-subject brain. Neuroimage. 2002; 15:273–289.


23. Pickut BA, Dierckx RA, Dobbeleir A, Audenaert K, Van Laere K, Vervaet A, et al. Validation of the cerebellum as a reference region for SPECT quantification in patients suffering from dementia of the Alzheimer type. Psychiatry Res. 1999; 90:103–112.


24. Soonawala D, Amin T, Ebmeier KP, Steele JD, Dougall NJ, Best J, et al. Statistical parametric mapping of (99m)Tc-HMPAO-SPECT images for the diagnosis of Alzheimer's disease: normalizing to cerebellar tracer uptake. Neuroimage. 2002; 17:1193–1202.


25. Wu JC, Gillin JC, Buchsbaum MS, Chen P, Keator DB, Wu NK, et al. Frontal lobe metabolic decreases with sleep deprivation not totally reversed by recovery sleep. Neuropsychopharmacology. 2006; 31:2783–2792.


26. Thomas M, Sing H, Belenky G, Holcomb H, Mayberg H, Dannals R, et al. Neural basis of alertness and cognitive performance impairments during sleepiness. I. Effects of 24 h of sleep deprivation on waking human regional brain activity. J Sleep Res. 2000; 9:335–352.


27. Petiau C, Harrison Y, Delfiore G, Degueldre C, Luxen A, Horne J, et al. Modification of fronto-temporal connectivity during a verb generation task after a 30 hour total sleep deprivation. A PET study. J Sleep Res. 1998; 7:Supple 2. 208.
28. Cajochen C, Foy R, Dijk DJ. Frontal predominance of a relative increase in sleep delta and theta EEG activity after sleep loss in humans. Sleep Res Online. 1999; 2:65–69.
29. Jones K, Harrison Y. Frontal lobe function, sleep loss and fragmented sleep. Sleep Med Rev. 2001; 5:463–475.


30. Cajochen C, Khalsa SB, Wyatt JK, Czeisler CA, Dijk DJ. EEG and ocular correlates of circadian melatonin phase and human performance decrements during sleep loss. Am J Physiol. 1999; 277(3 Pt 2):R640–R649.