Abstract
Breast magnetic resonance imaging (MRI) has been increasingly utilized, especially in screening for high-risk cases, because of its high sensitivity and superior ability to detect cancers as compared with mammography and ultrasound. Several limitations such as higher cost, longer examination time, longer interpretation time, and low availability have hindered the wider application of MRI, especially for screening of average-risk women. To overcome some of these limitations and increase access to MRI screening, an abbreviated breast MRI protocol has been introduced. Abbreviated breast MRI is becoming popular and challenges the status quo. This review aims to present an overview of abbreviated MRI, discuss the current findings, and introduce ongoing prospective trials.
Screening with mammography is associated with a 16–40% relative reduction in breast cancer mortality among women aged 40–74 years (1234). Mammography has been the most cost-effective method of breast cancer screening to date (5). However, the ability of mammography to detect cancer is reduced significantly when the breast tissue is dense (6789). Estimated false-negative rates for cancers in mammography screening are 10–30% (10). In dense breasts, which are present in about 50% of women (11), cancers are more likely to be obscured by the overlying tissues. Thus, supplemental screening has been recommended for women with dense breast. In addition, the enactment of breast density notification legislation has led to greater support for adjunct imaging screening methods for women with dense breast tissue.
Ultrasound, a readily available technology, has become the primary supplemental imaging modality for screening women with dense breast who are at average or intermediate risk for breast cancer. Multiple studies have consistently shown that breast screening ultrasound can detect an additional 2–4 mammographically occult cancers per 1000 women screened (121314). However, breast screening ultrasound has many limitations, including time, cost, and a low positive predictive value (PPV) of approximately 8% for biopsy. A low PPV for biopsy leads to a significant number of unnecessary biopsies and a much higher rate of short-term follow-up (1213). These facts suggest that the sole use of breast ultrasound to screen women with an average or intermediate risk for breast cancer may be problematic.
Reasons supporting the use of magnetic resonance imaging (MRI) for breast cancer screening can be summarized as follows: 1) better performance of MRI and 2) the biological characteristics of MRI-detected cancers.
Table 1 summarizes trials that compared the diagnostic accuracy of MRI with screening mammography, with or without ultrasound. In brief, breast MRI is effective for the detection of breast cancer in women at an increased risk for breast cancer. When compared with mammography and ultrasound, the efficacy of MRI in detecting breast cancer is much higher. The interval cancer rate is an appropriate metric for assessing the performance of screening programs. Expected or accepted interval cancer rates in European mammographic screening programs range from 30% to 50% of cancers observed in screening participants (23). In contrast, reported interval cancer rates among MRI screening groups were much lower, with one study even reporting an interval cancer rate of 0% (24).
Regarding the biological characteristics of MRI-detected cancers, Sung et al. (25) compared the clinical, imaging, and histopathologic features of breast cancers detected with screening MRI and mammography and interval cancers in 7519 high-risk women with 18064 screening MRI and 26866 screening mammography. In contrast to mammography, cancers detected with MRI were more likely to be invasive, while cancers detected with mammography were more likely to be ductal carcinoma in situ (DCIS), presenting as calcifications. In the prospective multicenter cohort study by Kuhl et al. (16), two cancer cases were diagnosed solely by mammography (2/27), no cases were diagnosed solely by ultrasound, and 14 cases were diagnosed solely by MRI (14/27). The additional lesions depicted solely by mammography were all low-grade DCIS. In contrast, of the 14 MRI-detected cancers, eight were invasive (from a total of 16 invasive cancers) and six were DCIS (from a total of 11 DCIS). These results suggest that MRI may be able to detect clinically more significant cancers than mammography is able to. Cancers missed by MRI are usually less aggressive and not invasive. Increasing the detection of biologically significant cancers may decrease mortality and reduce overdiagnosis and overtreatment.
Based on evidence from non-randomized trials and observational studies, the American Cancer Society (ACS) recommends MRI screening each year for women with a high risk for breast cancer in addition to annual screening mammography, in particular for women with a relative lifetime risk greater than 20% (2627). MRI screening for these women has been reported to be cost-effective (2829).
Although some data suggest that breast MRI may be useful for the detection of breast cancer in women at moderately increased risk, the ACS is equivocal with regard to annual MRI screening for these women due to insufficient evidence (1730313233). Women at moderately increased risk for breast cancer, a lifetime risk of 15–20%, include those with a personal history of breast cancer, DCIS, lobular carcinoma in situ, atypical ductal hyperplasia or atypical lobular hyperplasia, and “extremely dense” or “heterogeneously dense” breasts as assessed by mammography. The cost-effectiveness of screening breast MRI depends on estimated breast cancer incidence and examination costs. As such, screening MRI women at intermediate risk, including those with dense breast tissue as the sole risk factor, may not be cost-effective.
The rationale for abbreviated screening breast MRI is to reduce the image acquisition time of breast MRI as well as the image interpretation time. Its aim is to reduce the costs associated with screening breast MRI studies, to improve women's and physicians' acceptance of breast MRI, and to improve the feasibility of breast MRI for screening.
For this purpose, Kuhl et al. (34) prospectively evaluated an abbreviated MRI protocol consisting of one T1-weighted pre-contrast and one post-contrast series and their derived images prior to evaluating the full diagnostic protocol for 606 screening breast MRIs. The diagnostic accuracy of the abbreviated protocol was equivalent to that of the full diagnostic protocol, yielding an incremental cancer detection rate of 18.2 per 1000. The abbreviated protocol reduced acquisition time to 3 minutes. After this initial study by Kuhl et al. (34), there have been many more studies on abbreviated MRI, although protocols vary from institution to institution.
In general, a typical breast MRI examination generates several hundred images for interpretation and requires up to 40 minutes for image acquisition. In contrast, an abbreviated protocol may consist of only one pre-contrast image, one post-contrast image, and their derived images (subtraction and maximum-intensity projection [MIP] images). These methods can significantly reduce acquisition and interpretation times (34353637383940). Table 2 provides a summary of the abbreviated protocols. Some studies evaluating abbreviated MRI have included T2-weighted images or later post-contrast phases (Table 2) (353738).
The rationale for using the first post-contrast sequence is based on the observation that cancer is best visualized during the early arterial phase after contrast injection when the contrast between the angiogenic tumor and the adjacent fibroglandular tissue is greatest (4142). Later dynamic sequences and other pulse sequences are traditionally used for further characterization, rather than for detection of enhancing lesions. In contrast to malignant lesions, benign changes and background parenchymal enhancement (BPE) of normal fibroglandular tissue exhibit slow persistent enhancement, causing decreased visualization and lower false positive interpretations.
Summaries of performance, acquisition time, and interpretation time are provided in Table 3. Overall, abbreviated MRI has been shown to have a diagnostic accuracy similar to that of full diagnostic MRI protocols, while reducing the time for image acquisition and interpretation. Despite the variability of the pulse sequence design, results published by different groups consistently indicate that abbreviated protocols provide equivalent cancer detection rates and diagnostic accuracy compared with full diagnostic protocols. Overall, interpretation times needed for abbreviated protocols are reduced compared with full diagnostic protocols, mainly because of the decreased number of sequences to review.
In their prospective observational study of 443 women at mild to moderately increased breast cancer risk who underwent 606 screening MRI examinations, Kuhl et al. (34) reported that the diagnostic accuracy of the abbreviated protocol was equivalent to that of the full diagnostic protocol, yielding an incremental cancer detection rate of 18.2 per 1000. The researchers in this study used non-fat-suppressed axial imaging. Like the full diagnostic protocol, the complete abbreviated protocol detected all cancers present (11 of 11 [100%]). Specificity and PPV of the abbreviated protocol were equivalent to those of the full diagnostic protocol (94.3% vs. 93.9% and 24.4% vs. 23.4%, respectively). MIP reading only missed one cancer (1/11; sensitivity, 90.9%) when compared with the complete abbreviated protocol and the full diagnostic protocol; there was however no statistical significance (p = 0.317). With the abbreviated MRI protocol, acquisition time was reduced to 3 minutes. Sixty-one percent of the examinations were negative on MIP and could be read in less than 3 seconds. The authors noted that this compared favorably to times published for batch reading of screening mammograms, which ranged between 60 and 120 seconds (4344).
Heacock et al. (35) evaluated abbreviated MRI images of 107 biopsy-proven breast cancers. In this study, the abbreviated MRI protocol consisted of fat-suppressed sagittal T1-weighted pre-contrast, post-contrast, and subtracted images. Three readers conducted the assessments using 3 abbreviated protocols: 1) without clinical information and prior imaging, 2) with clinical information and prior imaging, and 3) after adding T2-weighted images; they reported sensitivities ranging from 97.8% to 99.4% for these 3 protocols. The researchers concluded that T2-weighted imaging did not improve the detection rate and confirmed that abbreviated MRI has a high rate of cancer detection. Interestingly, a shorter interpretation time was associated with masses as compared with non-mass enhancements and increased initial enhancement ratios (the mean percentage increase in signal between the pre-contrast and first post-contrast series). The researchers also observed that the initial enhancement ratio correlated significantly with increasing tumor grade, invasive disease, and lesion conspicuity, and they suggested that the rapid wash-in characteristics of malignancy may contribute to the efficacy of abbreviated MRI protocols.
In a study by Mango et al. (36) involving 100 consecutive breast MRI examinations in patients with biopsy-proven unifocal breast carcinomas, four readers evaluated only the first post-contrast T1-weighted images and their subtractions, as well as the respective MIP. They also used fat-suppressed sagittal imaging. The average sensitivity among the four readers was 96%. The average time needed to read was 44 seconds for the abbreviated protocol, while the published mean time to read a standard MRI was 4.7 minutes (45). Mango et al. (36) and Heacock et al. (35) conducted their studies in known breast cancer patients, which does not reflect real clinical situations. In addition, they did not perform an observer test for the full diagnostic protocol. Therefore, a comparison of cancer detection rates between the abbreviated and full diagnostic protocols cannot be made.
Grimm et al. (37) conducted a multi-reader study using two different abbreviated protocols on 48 patients (24 normal, 12 benign, and 12 malignant). Protocol 1 consisted of fat-saturated T2-weighted images, followed by one pre-contrast and the first post-contrast T1-weighted acquisition. Protocol 2 consisted of protocol 1 images plus a second post-contrast acquisition. The authors reported no statistically significant differences in cancer detection rates (sensitivity) between abbreviated protocols 1 (86%; p = 0.22) and 2 (89%; p = 0.38) and the diagnostic protocol (95%). Recorded interpretation times for abbreviated protocol 1 and the full protocol were similar (2.98 minutes vs. 3.56 minutes). It was postulated that the three to four additional sequences in the full protocol (non-fat-saturated T1 and two to three dynamic post-contrast sequences) may not be routinely used in clinical decision making, or that the readers may have spent more time reviewing the available sequences in the abbreviated protocol to ensure confidence in their interpretations.
Moschetta et al. (38) conducted a study of 470 patients with 185 breast lesions undergoing breast MRI for a variety of clinical indications (screening, staging, problem-solving). The term “abbreviated” in this study was used to indicate that the respective pulse sequence was somewhat shorter than the sequence used on a regular basis by the same group. The authors reported equivalent sensitivity, specificity, PPV, and negative predictive value for the “abbreviated” and full MRI protocols. The finding that reading times reported by Moschetta et al. (38) were longer than those reported by Kuhl et al. (34) and Mango et al. (36) is likely due to the addition of morphologic pre-contrast sequences. The post-processing and reading times for the abbreviated protocol were significantly shorter than those for the standard protocol.
Harvey et al. (39) conducted a study on the use of abbreviated protocols for high-risk screening. The regular breast MRI protocol that they used took 30 minutes to acquire, while the abbreviated protocol decreased that time to just over 10 minutes. The researchers reported an interpretation time of 1.55 minutes for the abbreviated protocol and observed equivalent diagnostic accuracies for both the abbreviated and full protocols. They explained that changes in patient management were observed in 12 out of 568 MRI studies (2.1%). What should be noted, though, is that images used in this study were from patients with cancer. Thus, the readers were aware of cancer in each case, and their results cannot be applied to a real screening program.
Chen et al. (40) conducted a study on the use of two abbreviated protocols for 356 women with dense breast examined by mammography. Protocol 1 consisted of fat-suppressed T2-weighted imaging, one pre-contrast image, and the first (earlier) post-contrast T1-weighted image. Interestingly, protocol 2 consisted of protocol 1 images plus a diffusion-weighted imaging (DWI). There were no significant differences among the three protocols in diagnosing breast cancer. However, the specificity of protocol 1 was significantly lower than that of protocol 2 and the full diagnostic protocol, whereas there was no difference between protocol 2 and the full diagnostic protocol. The researchers concluded that protocol 1 (commonly used abbreviated protocol) combined with DWI is effective in improving the specificity of breast cancer detection.
There is a growing body of evidence suggesting that abbreviated MRI demonstrates good performance and has many advantages when compared to full diagnostic MRI. However, to ensure that it is used appropriately and safely, several items should be considered.
First, eliminating additional sequences from the full diagnostic protocol may limit interpretation and affect potential recall rates in screening breast MRI. In such cases, indeterminate findings from abbreviated MRI screening should be recalled for additional diagnostic evaluation to enable further characterization. This would, however, lead to an overall increase in cost. For example, Kuhl et al. (34) found that more than one-third of potentially benign findings in the abbreviated protocol images could be downgraded to benign after interpretation in the full diagnostic protocol, obviating short-term follow-up. This finding substantiates the concern that abbreviated protocols may result in higher recall rates and cost. To increase the specificity and confidence of diagnosis, three studies of abbreviated MRI included T2-weighted sequences within their abbreviated protocols (353738). Although T2-weighted sequences did not improve reader cancer detection in the study by Heacock et al. (35), two of the three readers reported significantly increased confidence in lesion evaluation when these were included. In addition, the lack of kinetic information may necessitate recall for diagnostic MRI evaluation. However, Grimm et al. (37) found that the addition of the second post-contrast series for lesion kinetic analysis did not affect reader sensitivity or specificity. This may be in accordance with previous work which indicated that fewer post-contrast sequences may not significantly impact diagnostic accuracy (46).
Second, there is the possibility of a lack of lesion characterization or further analysis. The usual, multiparametric, full diagnostic breast MRI provides information on high-resolution, cross-sectional lesion morphology, as well as functional information on a variety of tissue perfusion, vessel permeability, tissue relaxation times, tissue cellularity/proliferation rate, and interstitial pressure. Such information can be useful for tissue characterization and contribute to the distinction of benign versus malignant lesions, as well as for a further classification of the biological and prognostic importance of breast cancers. For example, in most abbreviated MRI protocols, only early kinetic characteristics of the lesion are obtained, while delayed characteristics are not. Lesions with a fast uptake of contrast followed by a wash-out at the delayed phase are more likely to be malignant, while lesions with a slow but persistent uptake are more likely to be benign; although there is overlap between the kinetic assessment of benign and malignant lesions using MRI. To overcome this shortcoming, combination of ultrafast MRI into abbreviated MRI protocol has been investigated (47).
Third, we are not certain that preliminary results can be translated to community clinical practice, because published studies were performed by expert readers. However, if abbreviated MRI is adopted into clinical screening programs, reader expertise would increase over time, as observed in mammography screening programs. Minimum standards for reader qualification could be introduced to ensure that the benefits of abbreviated MRI are realized while minimizing false positives caused by reader inexperience. Similarly, minimum requirements for scanners would be necessary.
Fourth, since abbreviated MRI is performed for screening purposes, we have to pay attention to scheduling. Usually, when the BPE of normal fibroglandular breast tissue is low, abnormal findings are more conspicuous, and false positive findings are less frequent (484950). Marked BPE can limit the sensitivity of the examination (51), because differentiation of enhancing abnormalities from BPE may present a diagnostic challenge (48). It is recommended that premenopausal women should ideally be scanned during the second week of the menstrual cycle, avoiding the fourth week if possible (41). Scheduling appointments for premenopausal women requires flexibility, and appointment times would need to be adjusted on an individual basis.
Fifth, the indications for abbreviated MRI should be validated. The ACS has established recommendations (27) based on the current literature recommending annual breast MRI as an adjunct to annual mammography for women aged 30 and over with a high lifetime risk (> 20–25%) of developing breast cancer, as determined by risk-assessment computer models of key factors such as family history. Women with a ≥ 20% lifetime risk for breast cancer are recommended to undergo a standard full breast MRI and not an abbreviated MRI until its role in this high-risk population has been fully established. The target population for screening with abbreviated MRI is recommended for women with an average to intermediate risk for breast cancer (with a 15–20% lifetime risk) who have mammographically dense breasts.
Sixth, we have to consider the benefits and risks of using abbreviated MRI for screening average-risk women, as abbreviated MRI uses contrast material. Since McDonald et al. (52) reported that the use of intravenous gadolinium-based contrast agents is associated with neuronal tissue deposition, even without renal dysfunction, many further studies have been performed, one of which reported that gadolinium deposition could be extensive in multiple organs in rats (53). Based on these observations, a screening interval with abbreviated MRI or a combination with other modalities should be considered. Annual check-ups would not be appropriate. Similar to full diagnostic MRI, abbreviated MRI should also be avoided in women with severely impaired renal function, as gadolinium-based contrast agents may cause a rare condition known as nephrogenic systemic fibrosis (54).
Comstock and Kuhl have completed the prospective enrollment of the multicenter Eastern Cooperative Oncology Group (ECOG)-American College of Radiology Imaging Network (ACRIN) study: Comparison of Abbreviated Breast MRI and Digital Tomosynthesis in Breast Cancer Screening in Women with Dense Breasts (EA1141). Asymptomatic women aged 40–75 years old with dense breast tissue (mammographic density categories C and D) and without breast cancer-related risk factors underwent digital breast tomosynthesis and abbreviated breast MRI in randomized order for two consecutive years. Cancer detection rates achieved by digital breast tomosynthesis versus abbreviated breast MRI in the same women in the first (prevalence) and second (incidence) screening rounds will be calculated. Additionally, PPV and the types of cancer found by the two modalities will be compared. The target sample size includes 1450 women.
In Korea, a prospective multicenter trial using abbreviated MRI has also been ongoing (clinicaltrials.gov Identifier: NCT03475979). Women aged 25–69 years old, who are indicated for the BRCA mutation test with a history of breast cancer surgery will be screened with two rounds of mammography, ultrasound, and abbreviated MRI. The primary endpoint is to compare the cancer detection yields and false positive rates in BRCA mutation carriers with non-carriers for each screening modality. The researchers will also compare the sensitivity, specificity, and interval cancer rates between mammography, ultrasound, and abbreviated MRI in patients with BRCA mutations and in those treated for breast cancer. In addition, they will analyze the characteristics of subsequent cancer over a 5-year follow-up period. The target patient number is approximately 1600.
Using the abbreviated MRI protocol for breast cancer screening, the high diagnostic accuracy of full MRI protocols is maintained, while the time and cost associated with traditional MRI examinations are minimized. To be used optimally, abbreviated MRI is recommended for screening moderate-risk women with dense breasts. Screening should be performed taking the menstrual cycle into consideration, and interpretations should be conducted by experienced readers.
Figures and Tables
Table 1
Comparison of Performance of Mammography, Ultrasound, and MRI
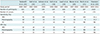
Sardanelli et al. (15) | Kuhl et al. (16) | Lehman et al. (17) | Kuhl et al. (18) | Leach et al. (19) | Leach et al. (19) | Warner et al. (20) | Morris et al. (21) | Vreemann et al. (22) |
---|---|---|---|---|---|---|---|---|
Study period | 2000–2007 | 2002–2005 | 2005 | 1996–2002 | 1997–2004 | 1997–2003 | 2000–2001 | 2003–2014 |
Number of participants | 501 | 687 | 195 | 529 | 648 | 256 | 367 | 2463 |
Number of cancers | 52 | 27 | 6 | 43 | 35 | 22 | 14 | 170 |
Sensitivity (%) | ||||||||
MRI | 91 | 93 | 100 | 93 | 77 | 77 | 100 | 81.4 |
Mammography | 50 | 33 | 33 | 33 | 40 | 36 | 0 | 51.7 |
US | 52 | 37 | 17 | 40 | NA | 33 | NA | NA |
PPV (%) | ||||||||
MRI | 65 | 48 | 43 | 50 | 7 | 49 | 17 | 28 |
Mammography | 71 | 39 | 50 | 23 | 10 | 83 | NA | 40 |
US | 62 | 33 | 25 | 11 | NA | 23 | NA | NA |
Table 2
Summary of MRI Sequences for Abbreviated MRI Protocols
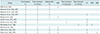
Study | Pre-Contrast T1 | Post-Contrast T1 (First) | Subtraction T1 | MIP | Post-Contrast T1 (Second) | Post-Contrast T1 (Third) | T2 | STIR | DWI |
---|---|---|---|---|---|---|---|---|---|
Kuhl et al. (34) | √ | √ | √ | √ | |||||
Heacock et al. (35), AP1 | √ | √ | √ | ||||||
Heacock et al. (35), AP2 | √ | √ | √ | ||||||
Heacock et al. (35), AP3 | √ | √ | √ | √ | |||||
Mango et al. (36) | √ | √ | √ | √ | |||||
Grimm et al. (37), AP1 | √ | √ | √ | ||||||
Grimm et al. (37), AP2 | √ | √ | √ | √ | |||||
Moschetta et al. (38) | √ | √ | √ | √ | |||||
Harvey et al. (39) | √ | √ | √ | √ | |||||
Chen et al. (40), AP1 | √ | √ | √ | √ | √ | ||||
Chen et al. (40), AP2 | √ | √ | √ | √ | √ | √ |
Table 3
Summarized Results from Six Studies of Abbreviated MRI Protocols
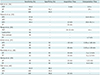
Sensitivity (%) | Specificity (%) | Acquisition Time | Interpretation Time | |
---|---|---|---|---|
Kuhl et al. (34) | ||||
MIP | 90.9 | - | 3 min | 2.8 s |
AP | 100 | 94.3 | - | 28 s |
FP | 100 | 93.9 | - | - |
Heacock et al. (35) | ||||
AP1 | 97.8 | - | - | 14.0–25.4 s |
AP2 | 99.4 | - | - | - |
AP3 | 99.4 | - | 12 min | 19.0–35.3 s |
FP | - | - | 35 min | - |
Mango et al. (36) | ||||
MIP | 93 | - | 10–15 min | 44 s |
Subtraction | 96 | - | - | - |
Post-contrast | 96 | - | - | - |
FP | - | - | - | 4.7 min |
Grimm et al. (37) | ||||
AP1 | 86 | 52 | −10 min | 2.98 ± 1.86 min |
AP2 | 89 | 45 | - | - |
FP | 95 | 52 | 20 min | 2.95 ± 1.59 min |
Moschetta et al. (38) | ||||
AP | 89 | 91 | 10 min | 2 ± 1.2 min |
FP | 92 | 92 | 16 min | 6 ± 3.2 min |
Harvey et al. (39) | ||||
AP | 89 | 91 | 10 min | 1.55 min |
FP | 92 | 92 | 16 min | 6.43 min |
Chen et al. (40) | ||||
AP1 | 92.9 | 86.5 | - | - |
AP2 | 100 | 95.0 | - | - |
FP | 100 | 96.8 | 32 min | - |
References
1. de Koning HJ. Mammographic screening: evidence from randomized controlled trials. Ann Oncol. 2003; 14:1185–1189.

2. Tabar L, Yen MF, Vitak B, Chen HH, Smith RA, Duffy SW. Mammography service screening and mortality in breast cancer patients: 20-year follow-up before and after introduction of screening. Lancet. 2003; 361:1405–1410.


3. Nelson HD, Cantor A, Humphrey L, Fu R, Pappas M, Daeges M, et al. Screening for breast cancer: a systematic review to update the 2009 U.S. preventive services task force recommendation [Internet]. Report No. 14-05201-EF-1. Rockville, MD: Agency for Healthcare Research and Quality (US);2016.
4. Lauby-Secretan B, Scoccianti C, Loomis D, Benbrahim-Tallaa L, Bouvard V, Bianchini F, et al. Breast-cancer screening: viewpoint of the IARC Working Group. N Engl J Med. 2015; 372:2353–2358.
5. Feig S. Cost-effectiveness of mammography, MRI, and ultrasonography for breast cancer screening. Radiol Clin North Am. 2010; 48:879–891.


6. Mandelson MT, Oestreicher N, Porter PL, White D, Finder CA, Taplin SH, et al. Breast density as a predictor of mammographic detection: comparison of interval- and screen-detected cancers. J Natl Cancer Inst. 2000; 92:1081–1087.


7. Crystal P, Strano SD, Shcharynski S, Koretz MJ. Using sonography to screen women with mammographically dense breasts. AJR Am J Roentgenol. 2003; 181:177–182.


8. Kerlikowske K, Grady D, Barclay J, Sickles EA, Ernster V. Effect of age, breast density, and family history on the sensitivity of first screening mammography. JAMA. 1996; 276:33–38.


9. Buist DS, Porter PL, Lehman C, Taplin SH, White E. Factors contributing to mammography failure in women aged 40-49 years. J Natl Cancer Inst. 2004; 96:1432–1440.


10. Fletcher SW, Elmore JG. Clinical practice. Mammographic screening for breast cancer. N Engl J Med. 2003; 348:1672–1168.


11. Price ER, Hargreaves J, Lipson JA, Sickles EA, Brenner RJ, Lindfors KK, et al. The California breast density information group: a collaborative response to the issues of breast density, breast cancer risk, and breast density notification legislation. Radiology. 2013; 269:887–892.


12. Berg WA, Blume JD, Cormack JB, Mendelson EB, Lehrer D, Böhm-Vélez M, et al. Combined screening with ultrasound and mammography vs mammography alone in women at elevated risk of breast cancer. JAMA. 2008; 299:2151–2163.



13. Hooley RJ, Greenberg KL, Stackhouse RM, Geisel JL, Butler RS, Philpotts LE. Screening US in patients with mammographically dense breasts: initial experience with Connecticut Public Act 09-41. Radiology. 2012; 265:59–69.


14. Weigert J, Steenbergen S. The Connecticut experiments second year: ultrasound in the screening of women with dense breasts. Breast J. 2015; 21:175–180.


15. Sardanelli F, Podo F, Santoro F, Manoukian S, Bergonzi S, Trecate G, et al. Multicenter surveillance of women at high genetic breast cancer risk using mammography, ultrasonography, and contrast-enhanced magnetic resonance imaging (the high breast cancer risk italian 1 study): final results. Invest Radiol. 2011; 46:94–105.

16. Kuhl C, Weigel S, Schrading S, Arand B, Bieling H, König R, et al. Prospective multicenter cohort study to refine management recommendations for women at elevated familial risk of breast cancer: the EVA trial. J Clin Oncol. 2010; 28:1450–1457.


17. Lehman CD, Lee JM, DeMartini WB, Hippe DS, Rendi MH, Kalish G, et al. Screening MRI in women with a personal history of breast cancer. J Natl Cancer Inst. 2016; 108:djv349.


18. Kuhl CK, Schrading S, Leutner CC, Morakkabati-Spitz N, Wardelmann E, Fimmers R, et al. Mammography, breast ultrasound, and magnetic resonance imaging for surveillance of women at high familial risk for breast cancer. J Clin Oncol. 2005; 23:8469–8476.


19. Leach MO, Boggis CR, Dixon AK, Easton DF, Eeles RA, Evans DG, et al. Screening with magnetic resonance imaging and mammography of a UK population at high familial risk of breast cancer: a prospective multicentre cohort study (MARIBS). Lancet. 2005; 365:1769–1778.

20. Warner E, Plewes DB, Hill KA, Causer PA, Zubovits JT, Jong RA, et al. Surveillance of BRCA1 and BRCA2 mutation carriers with magnetic resonance imaging, ultrasound, mammography, and clinical breast examination. JAMA. 2004; 292:1317–1325.

21. Morris EA, Liberman L, Ballon DJ, Robson M, Abramson AF, Heerdt A, et al. MRI of occult breast carcinoma in a high-risk population. AJR Am J Roentgenol. 2003; 181:619–626.


22. Vreemann S, Gubern-Mérida A, Schlooz-Vries MS, Bult P, van Gils CH, Hoogerbrugge N, et al. Influence of risk category and screening round on the performance of an MR imaging and mammography screening program in carriers of the BRCA mutation and other women at increased risk. Radiology. 2018; 286:443–451.


23. European guidelines for quality assurance in breast cancer screening and diagnosis. Accessed August 24, 2018. Available at: http://www.google.com/url?sa=t&rct=j&q=&esrc=s&source=web&cd=1&ved=2ahUKEwiMlNy6vNbdAhWihOAKHU3PAdsQFjAAegQIChAC&url=http%3A%2F%2Fwww.euref.org%2Fdownloads%3Fdownload%3D24%3Aeuropean-guidelines-for-quality-assurance-in-breast-cancer-screening-and-diagnosis-pdf&usg=AOvVaw33H5VVb-pU7xQuLzzO8KcM.
24. Kuhl CK, Strobel K, Bieling H, Leutner C, Schild HH, Schrading S. Supplemental breast MR imaging screening of women with average risk of breast cancer. Radiology. 2017; 283:361–370.
25. Sung JS, Stamler S, Brooks J, Kaplan J, Huang T, Dershaw DD, et al. Breast cancers detected at screening MR imaging and mammography in patients at high risk: method of detection reflects tumor histopathologic results. Radiology. 2016; 280:716–722.



26. American College of Radiology. ACR practice parameter for the performance of contrast-enhanced magnetic resonance imaging (MRI) of the breast. Resolution 34. Revised 2018. Accessed September 25, 2018. Available at: https://www.acr.org/-/media/ACR/Files/Practice-Parameters/mr-contrast-breast.pdf.
27. Saslow D, Boetes C, Burke W, Harms S, Leach MO, Lehman CD, et al. American Cancer Society guidelines for breast screening with MRI as an adjunct mammography. CA Cancer J Clin. 2007; 57:75–89.

28. Plevritis SK, Kurian AW, Sigal BM, Daniel BL, Ikeda DM, Stockdale FE, et al. Cost-effectiveness of screening BRCA1/2 mutation carriers with breast magnetic resonance imaging. JAMA. 2006; 295:2374–2384.


29. Taneja C, Edelsberg J, Weycker D, Guo A, Oster G, Weinreb J. Cost effectiveness of breast cancer screening with contrast-enhanced MRI in high-risk women. J Am Coll Radiol. 2009; 6:171–179.


30. Kriege M, Brekelmans CT, Boetes C, Besnard PE, Zonderland HM, Obdeijn IM, et al. Efficacy of MRI and mammography for breast-cancer screening in women with a familial or genetic predisposition. N Engl J Med. 2004; 351:427–437.


31. Brennan S, Liberman L, Dershaw DD, Morris E. Breast MRI screening of women with a personal history of breast cancer. AJR Am J Roentgenol. 2010; 195:510–516.


32. Schacht DV, Yamaguchi K, Lai J, Kulkarni K, Sennett CA, Abe H. Importance of a personal history of breast cancer as a risk factor for the development of subsequent breast cancer: results from screening breast MRI. AJR Am J Roentgenol. 2014; 202:289–292.


33. Destounis S, Arieno A, Morgan R. Personal history of premenopausal breast cancer as a risk factor for referral to screening breast MRI. Acad Radiol. 2016; 23:353–357.


34. Kuhl CK, Schrading S, Strobel K, Schild HH, Hilgers RD, Bieling HB. Abbreviated breast magnetic resonance imaging (MRI): first postcontrast subtracted images and maximum-intensity projection-a novel approach to breast cancer screening with MRI. J Clin Oncol. 2014; 32:2304–2310.


35. Heacock L, Melsaether AN, Heller SL, Gao Y, Pysarenko KM, Babb JS, et al. Evaluation of a known breast cancer using an abbreviated breast MRI protocol: correlation of imaging characteristics and pathology with lesion detection and conspicuity. Eur J Radiol. 2016; 85:815–823.


36. Mango VL, Morris EA, David Dershaw D, Abramson A, Fry C, Moskowitz CS, et al. Abbreviated protocol for breast MRI: are multiple sequences needed for cancer detection? Eur J Radiol. 2015; 84:65–70.


37. Grimm LJ, Soo MS, Yoon S, Kim C, Ghate SV, Johnson KS. Abbreviated screening protocol for breast MRI: a feasibility study. Acad Radiol. 2015; 22:1157–1162.

38. Moschetta M, Telegrafo M, Rella L, Stabile Ianora AA, Angelelli G. Abbreviated combined MR protocol: a new faster strategy for characterizing breast lesions. Clin Breast Cancer. 2016; 16:207–211.


39. Harvey SC, Di Carlo PA, Lee B, Obadina E, Sippo D, Mullen L. An abbreviated protocol for high-risk screening breast MRI saves time and resources. J Am Coll Radiol. 2016; 13:374–380.


40. Chen SQ, Huang M, Shen YY, Liu CL, Xu CX. Abbreviated MRI protocols for detecting breast cancer in women with dense breasts. Korean J Radiol. 2017; 18:470–475.



41. Kuhl C. The current status of breast MR imaging. Part I. Choice of technique, image interpretation, diagnostic accuracy, and transfer to clinical practice. Radiology. 2007; 244:356–378.


42. Mann RM, Kuhl CK, Kinkel K, Boetes C. Breast MRI: guidelines from the European Society of Breast Imaging. Eur Radiol. 2008; 18:1307–1318.



43. Garg AS, Rapelyea JA, Rechtman LR, Torrente J, Bittner RB, Coffey CM, et al. Full-field digital mammographic interpretation with prior analog versus prior digitized analog mammography: time for interpretation. AJR Am J Roentgenol. 2011; 196:1436–1438.


44. Tchou PM, Haygood TM, Atkinson EN, Stephens TW, Davis PL, Arribas EM, et al. Interpretation time of computer-aided detection at screening mammography. Radiology. 2010; 257:40–46.


45. Lehman CD, Blume JD, DeMartini WB, Hylton NM, Herman B, Schnall MD. Accuracy and interpretation time of computer-aided detection among novice and experienced breast MRI readers. AJR Am J Roentgenol. 2013; 200:W683–W689.


46. Partridge SC, Stone KM, Strigel RM, DeMartini WB, Peacock S, Lehman CD. Breast DCE-MRI: influence of postcontrast timing on automated lesion kinetics assessments and discrimination of benign and malignant lesions. Acad Radiol. 2014; 21:1195–1203.


47. Oldrini G, Fedida B, Poujol J, Felblinger J, Trop I, Henrot P, et al. Abbreviated breast magnetic resonance protocol: value of high-resolution temporal dynamic sequence to improve lesion characterization. Eur J Radiol. 2017; 95:177–185.


48. DeMartini WB, Liu F, Peacock S, Eby PR, Gutierrez RL, Lehman CD. Background parenchymal enhancement on breast MRI: impact on diagnostic performance. AJR Am J Roentgenol. 2012; 198:W373–W380.


49. Kuhl CK, Bieling HB, Gieseke J, Kreft BP, Sommer T, Lutterbey G, et al. Healthy premenopausal breast parenchyma in dynamic contrast-enhanced MR imaging of the breast: normal contrast medium enhancement and cyclical-phase dependency. Radiology. 1997; 203:137–144.


50. Ellis RL. Optimal timing of breast MRI examinations for premenopausal women who do not have a normal menstrual cycle. AJR Am J Roentgenol. 2009; 193:1738–1740.


51. Amarosa AR, McKellop J, Klautau Leite AP, Moccaldi M, Clendenen TV, Babb JS, et al. Evaluation of the kinetic properties of background parenchymal enhancement throughout the phase of the menstrual cycle. Radiology. 2013; 268:356–365.


52. McDonald RJ, McDonald JS, Kallmes DF, Jentoft ME, Murray DL, Thielen KR, et al. Intracranial gadolinium deposition after contrast-enhanced MR imaging. Radiology. 2015; 275:772–782.


53. McDonald RJ, McDonald JS, Dai D, Schroeder D, Jentoft ME, Murray DL, et al. Comparison of gadolinium concentrations within multiple rat organs after intravenous administration of linear versus macrocyclic gadolinium chelates. Radiology. 2017; 285:536–545.

54. American College of Radiology. Committee on Drugs and Contrast Media. ACR Manual on contrast media, Version 10.1. Reston, VA: American College of Radiology;2015.