Abstract
Objective
This study was performed to evaluate the accuracy and reliability of a newly designed method to achieve mandibular dental model superimposition, using voxel-based cone-beam computed tomography (CBCT) registration.
Methods
Fourteen dry cadaveric mandibles and six teeth extracted from patients with severe periodontitis were used to establish 14 orthodontic tooth-movement models. The protocol consisted of two steps: in the first step, voxel-based CBCT mandible superimposition was performed; the reference comprised the external portion of the symphysis, extending to the first molar. The laser-scanned dental model image was then integrated with the CBCT image to achieve mandibular dental model superimposition. The entire process required approximately 10 minutes. Six landmarks were assigned to the teeth to measure tooth displacement, using tooth displacement on the superimposed laser-scanned mandibles as the reference standard. Accuracy was evaluated by comparing differences in tooth displacement based on the method and the reference standard. Two observers performed superimposition to evaluate reliability.
Results
For three-dimensional tooth displacements, the differences between the method and the reference standard were not significant in the molar, premolar, or incisor groups (p > 0.05). The intraclass correlation coefficients for the inter- and intra-observer reliabilities of all measurements were > 0.92.
Orthodontic tooth movement has been evaluated using cephalometric tracing techniques and superimposition. However, cephalometric radiographs are two-dimensional and can therefore suffer from overlapping of anatomical structures, distortion, magnification, and difficulty in landmark identification1; thus, they may exhibit potential errors in the evaluation of orthodontic tooth movement. Consequently, and using the development of a digital dental model, researchers have concentrated on models based on superimposition of three-dimensional (3D) serial dental images to accurately assess orthodontic treatment outcomes in three dimensions.23 However, a method allowing mandibular dental model superimposition has not yet been developed, due to limitations regarding stable reference regions, a requirement for any superimposition.
For maxillary dental model superimposition, the reference in early studies was the palatal rugae,4 followed by the palatal vault, which was sufficiently stable for registration.56 However, for a mandibular dental model, a stable anatomical structure suitable for use as a registration reference has not yet been determined. An et al.7 assessed the stability of buccal and lingual alveolar bone surfaces for mandibular digital model superimposition; notably, the results were unsatisfactory. Without stable regions, mandibular dental model superimposition cannot be implemented.
Images acquired using cone-beam computed tomography (CBCT) can be superimposed with point-, surface-, and voxel-based registration, which allows 3D visualization of the teeth or bony effects in orthodontic and orthognathic surgery.8 However, the quality of CBCT images can be influenced by streaking artifacts. In addition, the images of the teeth are not sufficiently precise, especially if occlusal and interproximal surface data are required,9 because errors in landmark assignment and measurements may result. Nevertheless, in the absence of maxillary interference, mandibular dental model superimposition is more intuitive than CBCT for assessing treatment outcomes, including those of orthodontic treatment.
In a previous study, Park et al.10 applied surface-based superimposition to a set of mandibular CBCT images and then combined digital dental models with the CBCT images to indirectly obtain mandibular dental model superimposition. While this method was innovative, the accuracy of the evaluation was insufficient; moreover, segmentation of the mandible and teeth from the CBCT image series was time-consuming. Additionally, Almukhtar et al.11 compared the accuracy of voxel- and surface-based CBCT superimposition; they concluded that the latter was subject to high variability.
The aim of this study was to test a newly designed method of mandibular dental model superimposition based on voxel-based CBCT registration, and to evaluate its accuracy and reliability in vitro.
The samples used in this retrospective study were 14 dry cadaveric mandibles, obtained from the Department of Anatomy and Histo-embryology of Peking University Health Science Center, China. Six teeth extracted from patients who had severe periodontitis were inserted into the tooth sockets of each dry mandible to establish a model of orthodontic tooth movement (Figure 1). The extracted teeth were approved for use in this project by the bioethics committee of the Peking University School and Hospital of Stomatology (PKUSSIRB-201311103). CBCT and laser scans of each dry mandible model were taken before (T1) and after (T2) the tooth was moved in the tooth socket to simulate orthodontic tooth movement (Figure 1). CBCT scans were acquired using a NewTom VG scanner (Aperio Services, Verona, Italy) at the following settings: 110 kVp, 1–2 mA, 12 × 8 cm field of view, scan time of 10 seconds, and voxel size of 0.3 mm. The scans were exported as digital imaging and communications in medicine (DICOM) files. The laser scans were obtained using a 3DTALK Discover scanner (scanning accuracy, 0.05 mm; 3DTALK, Jiangsu, China) and exported as standard tessellation language (STL) files. The entire process, including the method and evaluation, is shown in Figure 2.
The protocol for the superimposition method consisted of two steps: i) voxel-based CBCT mandible superimposition and ii) registration of the laser-scanned dental model image onto the CBCT image (Figure 3).
In the first step, CBCT DICOM files were imported into Dolphin Imaging software (ver. 11.9; Dolphin Imaging & Management Solutions, Chatsworth, CA, USA). CBCT T1 (CT1) and T2 (CT2) scans were opened using the fusion module tab of the software; this allowed the observer to manually move CT2 as close as possible to CT1, and to perform automatic voxel superimposition using subregional volumes and (as reference) the basal bone of the mandibular body, extending from the external part of the symphysis to the first molar (Figure 3). CT2, with the new orientation, was then saved and the skeletal models were reconstructed using the segmentation tool of the software. The images were exported as STL files.
In the second step, one observer imported the CBCT STL files and the laser-scanned dental models T1 (LDT1) and T2 (LDT2) into Geomagic software (ver. 2012; Geomagic International, Morrisville, NC, USA). The occlusal surface, interproximal contact surface, and gingival area would affect registration accuracy; thus, the occlusal and buccal surfaces of the tooth from the CBCT image, as well as the interproximal portion of the tooth and the gingival area from the laser-scanned dental model image, were removed (Figure 3). Then, the laser-scanned dental model images were integrated onto the CBCT images using the registration module. Manual registration was performed by selecting three points on the lingual cusps corresponding to each laser-scanned dental model image and CBCT image. Global registration was then applied until the two images matched as closely as possible. Completion of the above-described steps resulted in mandibular dental model superimposition (Figure 3). The entire process was conducted in <10 minutes.
The 3D Euclidean distance was measured between the two superimposed surfaces in the two steps, and the results were evaluated according to a color-coded map (Figure 4), using the root mean square (RMS) (defined in Equation 1) to assess the initial superimposition error of each step.
The scanning accuracy of the laser scanner was adequate to capture 3D images of the dry mandible models. The laser-scanned mandible T1 (LMT1) and T2 (LMT2) images were imported into Geomagic software (ver. 2012). Surface-based superimposition was performed using the whole mandible, with the exception of the tooth, as the reference. The 3D Euclidean distance of the two superimposed surfaces was measured and the results were evaluated by display in a color-coded map (Figure 4). Because the mandible was identical on both LMT1 and LMT2 images, the measurements obtained from the superimposed laser-scanned mandible models could be used to replace the 3D entity measurement that served as the reference standard in this study.
Landmarks were assigned to the bilateral molar mesiobuccal cusps, premolar buccal cusps, and the midpoints of the incisor edge for LDT1; these were then transferred to LDT2, LMT1, and LMT2 using crown superimposition to avoid errors in assignment (Figure 5). A 3D coordinate system was created using the gnathion (Gn) point as the origin (0,0,0), the mandibular plane as the x-y plane, and the line between the Gn point and the midpoint of the bilateral gonion (Go) points as the x-axis (Figure 5). The 3D tooth displacements of the molar, premolar, and incisor groups were then measured.
Accuracy was evaluated based on differences in 3D tooth displacements between the method and the reference standard. Two observers performed superimposition to evaluate reliability; the measurements were repeated 2 weeks later by one of the observers.
Statistical analysis was performed using IBM SPSS Statistics software (ver. 22.0; IBM Corp., Armonk, NY, USA). The mean and standard deviation of the RMS were obtained for each superimposition step. Paired t-tests between the method and the reference standard were used to evaluate the accuracy of the method. The intra- and inter-observer reliabilities of the measurements were assessed by means of intraclass correlation coefficients (ICCs).
To evaluate the error in the initial superimposition, the 3D Euclidean distance was measured; it is represented by a color-coded map in Figure 4. Table 1 shows the RMS value for each superimposition step of the method and laser-scanned mandible model superimposition. The RMS values of the voxel-based CBCT mandible superimposition, and of the registration of the laser-scanned dental model and CBCT images, were 0.09 ± 0.04 mm and 0.23 ± 0.02 mm, respectively. The RMS value for the laser-scanned mandible model superimposition was 0.04 ± 0.01 mm, indicating that the reference standard was reasonable and that its use was feasible.
Accuracy was evaluated based on the differences in 3D tooth displacements between the method and the reference standard. A statistical description and the inferred tooth displacement differences are shown in Table 2. With respect to 3D displacements, the differences between the method and the reference standard were not significant in the molar, premolar, or incisor groups (p > 0.05); the means were all < 0.1 mm, thereby indicating statistical accuracy and clinical acceptability of the mandibular dental model superimposition method.
The inter-observer reliability of the method was determined by comparing the tooth displacement measurements of two observers, using ICCs and 95% confidence intervals. The same observer repeated the measurements after 2 weeks to determine intra-observer reliability.
Table 3 shows the ICC results for the inter- and intraobserver reliabilities of all measurements. The ICC values were consistently > 0.92, indicating that this method is reliable.
This study presented and tested a new method for mandibular dental model superimposition. A previously described, indirect method of mandibular dental model superimposition using CBCT surface-based registration and an integrated 3D digital model with CBCT tooth portions10 was insufficiently accurate and time-consuming for usage.
In contrast, in our method, the first step consists of voxel-based CBCT mandible superimposition; in the second step, the skull model is reconstructed without segmenting the mandible and teeth from the CBCT image, which results in considerable time savings. Voxel-based CBCT superimposition differs from point- or surface-based superimposition, which uses volumetric units stored in a CBCT DICOM format. Each voxel has a unique gray-scale value that depends on the opacity of the structure scanned in that volume.12 Gray-scale differences in the voxels are then used to align the two CBCT images based on a maximum mutual information algorithm.13 The voxel-based method for CBCT superimposition was introduced by Cevidanes et al.14 and proven to be accurate and reliable.151617 Following developments in voxel-based CBCT mandible superimposition,1819 Koerich et al.19 evaluated the accuracy of the modality by calculating the RMS. The results obtained with living subjects (RMS ≤ 0.105) were similar to those obtained with dry skulls (RMS ≤ 0.184). Those results provided further evidence to support the accuracy and reproducibility of voxel-based CBCT mandible superimposition; this method was less time-consuming than surface-based superimposition.
Previous studies have recommended various methods for the registration of laser-scanned dental model images onto CBCT images.920 Noh et al.9 compared the influence of the registration area on the accuracy of registration and found no significant differences in accuracy for the mandibular arch, according to the area selected; however, differences in the maxillary arch were significant. Ye et al.21 integrated dental model and CBCT images using global registration; the registration error ranged from 0.163 to 0.345 mm, according to the voxel size. In our method, the global registration region comprised the whole tooth crown, except for the occlusal and buccal surfaces, based on the occlusion contact area. Because more tooth-crown data can be obtained from dry mandible models than from living patients, occlusal and buccal tooth surfaces were removed from the dry mandible models, in order to avoid any influence of registration area and to simulate clinical patients as much as possible.
In this study, the RMS results for voxel-based CBCT mandible superimposition, and for the registration of the laser-scanned dental model images onto the CBCT images, were similar to those reported in previous publications; this supported the accuracy of each step of this method and its clinical utility. Each step of the method is independent of the others, such that adding the RMS value of each step to evaluate the accuracy of the whole process would have been inappropriate. In previous publications, in the absence of a reference standard, the RMS value was considered a reliable index of the superimposition error. However, this assumption may cause bias when the RMS value is used to evaluate the superimposition error of two surface models from different sources, such as CBCT and laser-scanned dental images. In addition, the loss of occlusal and interproximal surface data for the CBCT-reconstructed model would magnify, or distort, the RMS value. To some extent, evaluating superimposition error in the context of multisource data registration is imprecise.
To evaluate the accuracy of our method, a 3D entity measurement would have been the gold standard. However, it was not possible to establish a common coordinate system in the entity models to measure tooth movement in three dimensions. We instead adopted laser-scanned mandible superimposition as the reference standard. The RMS value of laser-scanned mandible superimposition was 0.04 mm, which indicated that the two mandibles were in the same position and justified the use of 3D tooth displacements on the superimposed laser-scanned mandible as the reference standard. The accuracy of the method was then appropriately evaluated, based on the difference in tooth displacement between the method and the reference standard. The absence of statistically significant differences indicated the accuracy of the method and supported its clinical relevance. Furthermore, the ICC values of all measurements were excellent, demonstrating the reliability of the method. Furthermore, the whole process required less than 10 minutes in vitro, which was slightly smaller than that required in a patient (approximately 12 minutes).
With this method, CBCT imaging is necessary to obtain model superimposition. It requires additional radiation and cost, which may be a shortcoming of the current method. Furthermore, dry cadaveric mandibles without soft tissue were used in this study; although their CBCT images may differ from those of living patients, Lee et al.13 previously used dried human skulls to evaluate 3D CBCT image fusion based on a maximum mutual information algorithm. In the method of Koerich et al.,19 the accuracy of voxel-based CBCT mandible superimposition was assessed using CBCT scans of dried mandibles, and mandibles in living patients; importantly, the registration error results were similar. Nairn et al.22 used six dry skulls to determine the accuracy of replacing CBCT dentition images with digital dentition images. Although the CBCT images of dried skulls differed from those of living patients, the superimposition error was similar, supporting the feasibility of using dried skulls in accuracy evaluations. Moreover, the artifacts of CBCT images produced by metallic restorations cannot yet be resolved; we recommend that these portions should be removed before superimposition.
As noted above, the registration reference for voxel-based CBCT superimposition must be stable and remain unaffected by treatment or growth. Nada et al.23 compared the anterior cranial base and zygomatic arches for voxel-based CBCT superimposition and concluded that the left zygomatic arch could be used as a stable structure for the superimposition of smaller field of view CBCT scans where the anterior cranial base is not visible. Ruellas et al.24 evaluated three reference regions for mandibular superimposition based on voxel registration and concluded that the mandibular body mask was a reliable reference. In our study, the dry mandible models featured no bone remodeling, such that the reference area used in voxel-based CBCT superimposition was stable in adult patients; however, whether this area is also stable in growing patients remains to be determined. Consequently, our method is suitable for adult patients, but an analogous method for growing patients, in which a stable area has been confirmed, is still needed.
Before applying this method to evaluate orthodontic treatment outcomes in three dimensions, more data from real patients should be used to further validate our approach. Thus, this can serve as a pilot study for subsequent superimposition.
Figures and Tables
Figure 1
Dry mandible model acquisition. A, Six teeth were inserted into the tooth sockets of each dry cadaveric mandible to establish a dry mandible model. B, Laser-scan of a dry mandible model.
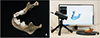
Figure 2
Flowchart of the method and evaluation process.
3D, Three-dimensional; CBCT, cone-beam computed tomography.
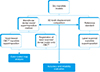
Figure 3
Steps comprising the mandibular dental model superimposition method. A–D, Step 1: Voxel-based cone-beam computed tomography (CBCT) mandible superimposition. The red frame indicates the registration reference area. D, Results of voxel-based CBCT mandible superimposition. E–H, Step 2: registration of the laser-scanned dental model and CBCT images. Selected reference points on the CBCT (E) and laser-scanned dental model (F) images; G, initial registration of the two images according to the reference points; H, completion of the two steps yields mandibular dental model superimposition.
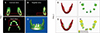
Figure 4
Color-coded map of the registration procedure. A–D, Color-coded visualization charts show the differences between the two images after the registration procedure. Results of (A) voxel-based cone-beam computed tomography (CBCT) superimposition and (B) registration of the laser-scanned dental model image onto the CBCT image; C, the reference standard: laser-scanned mandible superimposition; D, color-coded visualization chart showing the superimposition error.
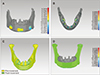
Figure 5
Landmark assignment and three-dimensional (3D) tooth displacement measurements. A, Landmarks were assigned to the bilateral molar mesio-buccal cusps, premolar buccal cusps, and midpoints of the incisor edges. B, Red circle: landmark transferred using crown superimposition to avoid errors in assignment. C, The 3D coordinate system.
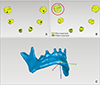
Table 1
Descriptive statistics for differences between the two images after the registration procedure (mm)
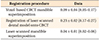
ACKNOWLEDGEMENTS
This work was supported by the Capital Health Research and Development of Special (Grant No. 2016-1-4102), the National Natural Science Foundation of China (Grant No. 81470717, Grant No. 81671015, Grant No. 81571815), the International Science & Technology Cooperation Program of China (Grant No. 2015DFB30040) and Beijing New-star Plan of Science and Technology (Grant No. Z171100001117018), Youth Fund of Peking University School of Stomatology (Grant No. PKUSS20150208).
The authors thank the Department of Anatomy and Histo-embryology of Peking University Health Science Center for providing the dry cadaveric mandibles for this research, as well as Trustworthy Technology Co. Ltd. (Beijing) for providing laser-scanner.
References
1. Bergersen EO. Enlargement and distortion in cephalometric radiography: compensation tables for linear measurements. Angle Orthod. 1980; 50:230–244.

2. Ashmore JL, Kurland BF, King GJ, Wheeler TT, Ghafari J, Ramsay DS. A 3-dimensional analysis of molar movement during headgear treatment. Am J Orthod Dentofacial Orthop. 2002; 121:18–29. discussion 29-30.


3. Miller RJ, Kuo E, Choi W. Validation of Align Technology's Treat III digital model superimposition tool and its case application. Orthod Craniofac Res. 2003; 6:Suppl 1. 143–149.


4. Hoggan BR, Sadowsky C. The use of palatal rugae for the assessment of anteroposterior tooth movements. Am J Orthod Dentofacial Orthop. 2001; 119:482–488.


5. Choi DS, Jeong YM, Jang I, Jost-Brinkmann PG, Cha BK. Accuracy and reliability of palatal superimposition of three-dimensional digital models. Angle Orthod. 2010; 80:497–503.


6. Chen G, Chen S, Zhang XY, Jiang RP, Liu Y, Shi FH, et al. Stable region for maxillary dental cast superimposition in adults, studied with the aid of stable miniscrews. Orthod Craniofac Res. 2011; 14:70–79.


7. An K, Jang I, Choi DS, Jost-Brinkmann PG, Cha BK. Identification of a stable reference area for superimposing mandibular digital models. J Orofac Orthop. 2015; 76:508–519.


8. Cevidanes LH, Bailey LJ, Tucker SF, Styner MA, Mol A, Phillips CL, et al. Three-dimensional cone-beam computed tomography for assessment of mandibular changes after orthognathic surgery. Am J Orthod Dentofacial Orthop. 2007; 131:44–50.



9. Noh H, Nabha W, Cho JH, Hwang HS. Registration accuracy in the integration of laser-scanned dental images into maxillofacial cone-beam computed tomography images. Am J Orthod Dentofacial Orthop. 2011; 140:585–591.


10. Park TJ, Lee SH, Lee KS. A method for mandibular dental arch superimposition using 3D cone beam CT and orthodontic 3D digital model. Korean J Orthod. 2012; 42:169–181.



11. Almukhtar A, Ju X, Khambay B, McDonald J, Ayoub A. Comparison of the accuracy of voxel based registration and surface based registration for 3D assessment of surgical change following orthognathic surgery. PLoS One. 2014; 9:e93402.


12. MacDonald-Jankowski DS, Orpe EC. Computed tomography for oral and maxillofacial surgeons. Part 2: cone-beam computed tomography. Asian J Oral Maxillofac Surg. 2006; 18:85–92.


13. Lee JH, Kim MJ, Kim SM, Kwon OH, Kim YK. The 3D CT superimposition method using image fusion based on the maximum mutual information algorithm for the assessment of oral and maxillofacial surgery treatment results. Oral Surg Oral Med Oral Pathol Oral Radiol. 2012; 114:167–174.


14. Cevidanes LH, Bailey LJ, Tucker GR Jr, Styner MA, Mol A, Phillips CL, et al. Superimposition of 3D cone-beam CT models of orthognathic surgery patients. Dentomaxillofac Radiol. 2005; 34:369–375.



15. Choi JH, Mah J. A new method for superimposition of CBCT volumes. J Clin Orthod. 2010; 44:303–312.

16. Weissheimer A, Menezes LM, Koerich L, Pham J, Cevidanes LH. Fast three-dimensional superimposition of cone beam computed tomography for orthopaedics and orthognathic surgery evaluation. Int J Oral Maxillofac Surg. 2015; 44:1188–1196.



17. Bazina M, Cevidanes L, Ruellas A, Valiathan M, Quereshy F, Syed A, et al. Precision and reliability of Dolphin 3-dimensional voxel-based superimposition. Am J Orthod Dentofacial Orthop. 2018; 153:599–606.


18. Koerich L, Weissheimer A, de Menezes LM, Lindauer SJ. Rapid 3D mandibular superimposition for growing patients. Angle Orthod. 2017; 87:473–479.


19. Koerich L, Burns D, Weissheimer A, Claus JD. Three-dimensional maxillary and mandibular regional superimposition using cone beam computed tomography: a validation study. Int J Oral Maxillofac Surg. 2016; 45:662–669.


20. Lin X, Chen T, Liu J, Jiang T, Yu D, Shen SG. Point-based superimposition of a digital dental model on to a three-dimensional computed tomographic skull: an accuracy study in vitro. Br J Oral Maxillofac Surg. 2015; 53:28–33.


21. Ye N, Long H, Xue J, Wang S, Yang X, Lai W. Integration accuracy of laser-scanned dental models into maxillofacial cone beam computed tomography images of different voxel sizes with different segmentation threshold settings. Oral Surg Oral Med Oral Pathol Oral Radiol. 2014; 117:780–786.


22. Nairn NJ, Ayoub AF, Barbenel J, Moos K, Naudi K, Ju X, et al. Digital replacement of the distorted dentition acquired by cone beam computed tomography (CBCT): a pilot study. Int J Oral Maxillofac Surg. 2013; 42:1488–1493.

