Abstract
Background
This study aimed to determine the effects of single semitendinosus tendon (ST) harvesting for anterior cruciate ligament (ACL) reconstruction by comparing outcomes of single ST and semitendinosus-gracilis tendon (ST-G) harvesting.
Methods
ACL reconstruction with ST-G harvesting (D group, n = 60) or single ST harvesting (S group, n = 60) were included according to inclusion criteria. Subjective assessments included subjective International Knee Documentation Committee score, Lysholm score, and Tegner activity scale score. Objective assessments included isokinetic strength and functional tests. These tests were completed at 36 months of follow-up. Magnetic resonance imaging (MRI) and second-look arthroscopy findings were evaluated. In the S group, regeneration properties were assessed by serial ultrasonography (US).
Results
The S group showed significantly less deep flexor strength deficit than the D group (p < 0.001). Deep flexor power deficits showed significant correlation with the shift of musculotendinous junction of the ST. There was significant difference in the cocontraction test between the groups (p = 0.012), and the S group tended to show better results in other functional tests at the last follow-up. There were no significant differences in graft tension and synovial coverage on second-look arthroscopy between the groups. In the S group, the regeneration rates assessed by US at the joint line and distal insertion were 81.7% and 80%, respectively at 6 months of follow-up.
Conclusions
The S group showed significantly less deficit in deep flexor strength and tended to show better clinical results at the last follow-up than the D group. In the S group, more than 80% showed good regeneration at the 6-month follow-up. Hence, single ST harvesting is effective in minimizing flexor weakness and functional deficits and shows great potential for regeneration.
No consensus is available on the optimal graft choice for anterior cruciate ligament (ACL) reconstruction even though the outcomes of single-bundle ACL reconstruction have been reported to be excellent.1) In the past decade, both bone-patellar tendon-bone and hamstring autografts were used extensively, and all graft choices have potential advantages and disadvantages.1) A recent systematic review of level 1 studies revealed that hamstring autograft was effective in patellofemoral problems and extension deficit.1) The hamstring autograft has recently become a more popular graft choice due to its excellent mechanical properties, lower donor site morbidity including minimal effect on the extensor mechanism, and high regeneration capability after harvesting.1)
The main drawback of semitendinosus-gracilis tendon (ST-G) harvesting is the considerable deficit in knee flexion and tibial internal rotation strength.2) Hence, the single semitendinosus tendon (ST) harvesting technique preserving the gracilis tendon (G) has been recommended by several studies.34) Sharma et al.5) performed a systematic review of studies comparing the results of single ST versus ST-G harvesting and concluded that the ST-G harvesting resulted in statistically significant decrease in isokinetic and isometric hamstring strength, which were larger at higher flexion angles. However, studies reported no significant differences in subjective and functional outcomes including hamstring strength between single ST and ST-G harvesting.6)
Nevertheless, the flexion strength and functional performance of the regenerated hamstring tendon remains unclear. Stevanovic et al.7) reported that 72% showed regeneration of ST and the isokinetic strength of the hamstrings was not significantly decreased on the operated side. They suggested that the regenerated ST could be used for iterative ligament reconstruction based on macroscopic and histological analysis. Conversely, Bedi et al.8) demonstrated that the regenerated tissue after hamstring harvesting did not reveal normal excursion or physiological function similar to the native muscle-tendon unit of the hamstring. Konrath et al.9) reported that the muscle-tendon properties of the ST-G were substantially altered after harvesting, which might contribute to flexor weakness in the involved limb.
This study aimed to determine the effects of single ST tendon harvesting for ACL reconstruction by comparing functional outcomes including muscle strengths between single ST and ST-G harvesting. In addition, we investigated the morphological properties on serial ultrasonography (US) after single ST harvesting and compared the functional outcomes between regeneration and no-regeneration groups. The hypothesis was that single ST harvesting would show increased deep flexor strength and a lower degree of proximal shift of the musculotendinous junction compared with the ST-G harvesting despite no significant difference in clinical results between the two groups. We also hypothesized that the ST would be regenerated within 6 months in most patients on serial US after single ST harvesting and the regenerated group would show better clinical results than the no-regeneration group at 6 months.
From June 2009 to March 2014, 583 consecutive patients who underwent primary single-bundle ACL reconstruction using hamstring autograft were reviewed. The inclusion criteria for this study were primary unilateral ACL reconstruction, patients who were younger than 40 years, more than 2 years of follow-up, magnetic resonance imaging (MRI) at 12 months postoperatively, and second-look arthroscopy at 24 months postoperatively. Exclusion criteria were multiple ligament injuries, concomitant fractures, contralateral injuries, subtotal or total meniscectomy at index surgery, revision surgery, infection, and cartilage lesion with modified Outerbridge grade ≥ 3. Based on these selection criteria, 60 patients who underwent ACL reconstruction with dual ST-G harvesting (D group) from June 2009 to June 2012 were included. Consecutively eligible patients with single ST harvesting (S group) performed from July 2012 to March 2014 were 60, who were matched to those in group D by age, sex, and preoperative sports activity level (Fig. 1). This retrospective comparative study was performed with the approval of the Ethics Committee of Konkuk University Medical Center (No. KUH1060153). Written informed consents were obtained.
Arthroscopic anatomic ACL reconstruction with a modified transtibial technique using hamstring autograft was conducted.10) All procedures were performed by an experienced single surgeon (JGK). Hamstring tendons were harvested through an oblique 3- to 4-cm incision at 2 cm medial to the tibial tuberosity. We dissected and freed the pes anserinus using a reverse L-shaped incision at sartorius fascia, and the flap was reflected. To maximize the length of hamstring autograft, we included periosteum from the tendon insertion site, which facilitates additional 1 or 2 cm of the hamstring autograft. In the S group, a single ST was harvested first and then cut and folded in half to obtain a quadrupled ST graft. A minimum length of 24 cm and diameter of 8 mm was required to produce a single quadrupled ST. If the length of the tendon was below 24 cm, the diameter of the quadrupled tendon was below 8 mm, or the tendon condition was not fit for graft use, the G as well as the ST were used to obtain a quadrupled graft; these cases were excluded from the S group. In the D group, after ST harvesting, additional G harvesting was routinely performed to create a quadrupled ST-G graft. Then, we anatomically sutured the sartorius fascia to facilitate anatomic hamstring regeneration.
The femoral attachment was debrided to identify the lateral intercondylar ridge and the bifurcate ridge that divide the posterolateral and anteromedial bundles of the ACL. The “funnel-shaped bony trough” was made after marking at the femoral anatomic center using the microfracture awl through the anteromedial portal viewing from the anterolateral portal. An ACL guide (Linvatec, Largo, FL, USA) was used to create the tibial tunnel at an angle of 47.5°. The ACL guide was positioned in the center of the remnant ACL stump, and the guide pin was placed above the pes anserinus and in front of the medial collateral ligament. The tibial tunnel was created with the same diameter as the graft using an expansion reamer. The guide pin was inserted into the tibial tunnel towards the preformed “funnel-shaped bony trough” with a free hand technique. The smallest diameter (6 mm on average) was used to start off the reaming just near the femoral cortex, and the reamer was expanded to produce a femoral tunnel with a diameter similar to that of the graft. After the graft passage, femoral fixation was achieved using the XO Button (ConMed Linvatec, Largo, FL, USA) and the Bio-Cross Pin (RIGIDFIX, Depuy Mitek, Raynham, MA, USA). We usually use a proximal pin of RIGIDFIX to avoid intra-articular protrusion of the cross pin.1011) If the femoral tunnel length was below 30 mm, the XO Button was used solely in a press-fit approach. Tibial fixation was performed with a bioabsorbable interference screw (Matryx, ConMed Linvatec), and additional cortical screw and washer were used for tibial fixation.
Rehabilitation was performed with the same method in both groups. Patients were permitted to bear weight with an ACL support brace (DonJoy Legend, DJO Global, Vista, CA, USA) as tolerated immediately after ACL reconstruction. Isometric quadriceps exercises, closed kinetic chain exercises, and range of motion exercises were started on the day after the surgery based on our accelerated rehabilitation program. When the meniscus was repaired, partial weight-bearing with a crutch was performed for 6 weeks. The ACL support brace was completely removed at 2 months postoperatively. Open kinetic leg curl exercises and perturbation training program were started at 8 weeks after surgery. After the home-based rehabilitation, running and competitive sports were targeted at 3 and 9 months after ACL reconstruction, respectively. Recovery of extensor muscle strength and the results of functional tests were reflected in running performance at 3 months and return to sports activity at 9 months after the surgery.
MRIs were taken at 12 months after ACL reconstruction during the period of return to sports. All patients underwent MRI examinations using a 3.0-T system apparatus (Signa HD, GE Healthcare, Milwaukee, WI, USA) following sequences of the knee of interest: (1) axial proton density fat saturated: repetition time, 2,000 ms; echo time, 35 ms; echo train length, 8 mm; bandwidth, 42 Hz/pixel; field of view, 16 cm; 384 × 384; and 3-mm contiguous; (2) coronal proton density fat saturated: repetition time, 2,000 ms; echo time, 35 ms; echo train length, 8 mm; bandwidth, 42 Hz/pixel; field of view, 16 cm; 384 × 384; and 2-mm contiguous; and (3) sagittal proton density fat saturated: repetition time, 2,000 ms; echo time, 35 ms; echo train length, 8 mm; bandwidth, 42 Hz/pixel; field of view, 16 cm; 384 × 384; and 2-mm contiguous. Analysis was performed on a picture archiving and communication system workstation (Centricity RA 1000, GE Healthcare). In the axial plane, the images at the knee joint level and 3.5 cm above the joint line were reviewed, and the ST and G were identified and classified according to the presence or absence of regeneration, based on the presence or absence of neotendon that crossed the joint line.1213) The shift in the musculotendinous junction was examined in the coronal and sagittal planes and was measured using the distal-most muscle signal intensity of the ST and G (Fig. 2).14) The distances were measured on preoperative and 12-month postoperative MRIs, and proximal and distal shifts were indicated as “+” and “−,” respectively. The shift of the distal insertion of the regenerated tendon was measured in the coronal plane as reported in a previous study, and at the upper end, both preoperative tendon insertion and regenerated tendon insertion were identified and compared (Fig. 3).14) Proximal and distal shifts were indicated as “+” and “−,” respectively. The MRI examinations in the present study were assessed independently by a musculoskeletal radiologist (JCS) and an orthopedic surgeon (JGK) with more than 10 years of experience, and each examiner evaluated MRIs twice 2 weeks apart.
Subjective knee scoring systems including International Knee Documentation Committee subjective knee score, Lysholm score, and Tegner activity scale were evaluated usually before the surgery and 6, 9, 12, 24, and 36 months postoperatively. Preoperative data and data obtained at 36 months after ACL reconstruction were used to evaluate subjective clinical outcomes.
Functional tests were performed usually before the surgery and 6, 9, 12, 24, and 36 months postoperatively, and were usually completed at 36 months of follow-up. The pivot shift tests of patients under anesthesia before the surgeries (primary ACL reconstruction and second-look arthroscopy) were conducted by the senior author (JGK), and the results were classified as grade 0, normal; grade 1, gliding; grade 2, clunk; and grade 3, locking. Anterior instability was evaluated by the side-to-side difference on a KT-2000 arthrometer (MEDmetric, San Diego, CA, USA) with maximal manual stress. The isokinetic muscle strength measurements were performed using Biodex System III dynamometer (Biodex Medical Systems, Shirley, NY, USA) at an angular velocity of 60°/sec. The isokinetic muscle strength was digitized as the peak torque (the maximum value during the four repetitions) of flexor and extensor muscles. Measurements were taken initially on the unaffected side, followed by the affected side. Standard isokinetic muscle strength in a sitting position (0° to 90°) at an angular velocity of 60°/sec was assessed, and deep flexor strength at an angular velocity of 60°/sec was assessed with the patient in prone position (60° to 120°). Strength deficit (%) of the involved side compared to the uninvolved side was calculated in all strength tests. The functional performance testing involved the single-leg hop for distance (SLHD) test reported by Noyes et al.;15) cocontraction test, which reproduces rotation movements that induce tibial translation; and the Carioca test, which reproduces pivot shift phenomenon.16) In the SLHD test, the patients were asked to hop forward as far as possible, jumping and landing with the same foot. It was performed three times, and the longest distances for the involved and uninvolved limbs were measured in cm using a ruler on the ground. The limb symmetry indices (LSI, %) were calculated by dividing the affected limb data by the unaffected limb data, and the result was multiplied by 100. The co-contraction test was performed by securing a velcro belt around the subject's waist. The belt was attached to a rubber tubing with a length of 48 inches and a diameter of 1 inch. The tube was anchored to a metal loop that was secured on a wall 60 inches above the floor. A semicircle with a radius of 96 inches from the metal loop was painted on the floor. The subjects standing with his or her toes on the line were asked to run wall to wall along the semicircular line five times (three times, right to left and two times, left to right) and the time to completion was measured. The fastest time was recorded. Carioca test was performed by asking the patient to run laterally two lengths of a 12-m distance with a crossover step. The patient ran the course from left to right and then in reverse direction and the fastest time was recorded.
Second-look arthroscopy and screw removal were performed only in patients who wanted to remove the irritable materials or voluntarily confirmed the status of the reconstructed ACL graft. All patients who underwent second-look arthroscopy were examined by the experienced single surgeon (JGK) after the cortical screw and washer were removed at least 24 months after the primary ACL reconstruction. Tension and synovial coverage of the ACL graft were evaluated using a probe with consistency between the main surgeon (JGK) and the first assistant (DWL). Tension of the ACL graft was evaluated subjectively with meticulous probing and was graded as follows: good (tautness), fair (slightly lax, but acceptable), and poor (no tension). Synovial coverage was classified as follows: covering 25% or less, 25% to 50%, 50% to 75%, and more than 75% as in previous studies.1718)
US was conducted to evaluate the process of regeneration of the ST after single ST harvesting (S group). We have performed US examination since July 2012 after single ST harvesting. Patients were imaged using a high-resolution 12-MHz linear array transducer (IU 22; Philips Medical Imaging, Bothell, WA, USA) by a musculoskeletal radiologist (JCS) with more than 10 years of musculoskeletal ultrasound experience. The patients underwent US before ST harvesting at 2 days and 1, 3, and 6 months postoperatively. During US evaluation, the knee was positioned in 30° of flexion, which placed the ST in it most superficial position. ST structure and margins as well as musculotendinous junction were assessed with the sagittal views, and thickness and width were measured with the axial views. The axial images obtained at the level of the medial joint line were used to calculate the cross-sectional area of the regenerated tissue. In addition, we compared clinical results between regeneration and no-regeneration groups at 6 months after surgery.
Statistical analysis was performed using IBM SPSS ver. 20.0 (IBM Corp., Armonk, NY, USA). The independent t-test or Mann-Whitney U-test was used to compare parametric or nonparametric variables between two groups. Preoperative and postoperative parametric or nonparametric variables were compared using the paired t-test or Wilcoxon's signed-rank test in each group. Intraclass correlation coefficients (ICCs) were calculated to determine interobserver reliability of different measurements for MRI assessments. Pearson or Spearman correlation test was used to identify correlation between isokinetic muscle strength and functional tests. The linear correlations were interpreted as follows: |r| = 0.5 – 1.0 as strong; |r| = 0.3 – 0.5 as moderate; and |r| = 0.1 – 0.3 as weak. The chi-square test was used to compare categorical data; if more than 20% of the expected frequencies were > 5, Fisher exact test was applied. As chi-square test for trend, linear by linear association test was used. Statistical significance was defined as p < 0.05. To detect a difference of 20% in deep flexor deficit between the two groups with a level of significance of 5% and a power of 80%, the required sample size was determined to be 22 patients per group. Assuming a dropout of 15%, 26 patients per each group were required.
Demographic data and clinical results showed no significant differences between the two groups (Table 1). Regeneration rates on MRI at 12 months after harvesting of the ST were 85% and 81.7% in the S group and D group, respectively; the G was regenerated in 76.7% of the D group (Table 2). The musculotendinous junction of ST shifted proximally by 3.8 ± 2.1 cm and 4.2 ± 2.2 cm in the S group and D group, respectively, and there was no significant statistical difference (p = 0.310). The mean shift of distal insertion of ST was −0.5 ± 0.7 cm and 0.3 ± 0.9 cm in the S group and D group, respectively, and there was significant difference (p = 0.041) (Table 2). The interobserver variability of MRI assessments was determined by ICCs. The ICCs for the shift of the musculotendinous junction of ST and G were 0.92 and 0.89, respectively, and those for the shift of distal insertion of ST and G were 0.89 and 0.87, respectively.
In involved limbs of two groups, significant knee extension and flexion deficits compared with uninvolved limbs were found in isokinetic muscle strength tests (all p < 0.001) (Table 3). On the extension peak torque and standard peak torque of involved limbs, the S group showed 189.1 ± 43.6 N·m and 102.3 ± 25.3 N·m, respectively, and the D group showed 183.5 ± 46.9 N·m and 95.2 ± 22.4 N·m, respectively. Although both groups showed no significant differences in extensor and flexor strength deficits, the mean extensor and standard flexor strength deficits were less in absolute value in the S group than in the D group (p = 0.228, p = 0.248, respectively). However, a significant difference between the two groups in deep flexor strength deficit was found as the S group showed significantly fewer deep flexor strength deficits than the D group (approximately 13.4% vs. 24.2%, p < 0.001). There were significant differences in co-contraction tests between both groups (p = 0.012), and the S group tended to show better results in other functional tests (Table 4). Extensor strength deficits and standard flexor strength deficits revealed moderate to strong positive or negative correlations with the mean time for co-contraction test and Carioca test, and LSI for SLHD. Deep flexor strength deficits showed mild positive correlation with the co-contraction test and moderate positive correlation with the shift of musculotendinous junction of ST on MRI (Table 5). There were no significant differences in graft tension and synovial coverage on second-look arthroscopy performed 24 months postoperatively between the two groups (Table 6).
In serial ultrasonographic evaluation of the S group, regeneration rates at the joint line level and distal insertion below the joint line were 76.7% and 73.3%, respectively, at 3 months after harvesting, and 81.7% and 80%, respectively, at 6 months after harvesting (Table 7 and Fig. 4). Two days after harvesting, the ST was absent in all cases (Fig. 4B). At 1 month after harvesting, an irregular echogenic and hypoechogenic tissue mass with hematoma, which was much larger in cross-section than a normal ST appeared in an anatomic tendon position. Hematoma-like soft tissue mass was found in 43 cases (71.7%) (Fig. 4C). Isolated soft tissue mass with a more echogenic signal was found at the joint level at 3 months after harvesting, and the mass was reduced in cross-sectional area compared to the earlier examination (Table 7 and Fig. 4D). Six months after harvesting, more uniform echo structure with more defined border, which resembled a normal ST was apparent although irregularities were still evident (Fig. 4E). Regenerated tendon tissue, which we call a neotendon, was found at distal insertion. There was no significant difference in regeneration rates between 3 months and 6 months after harvesting (Fig. 5). The average cross-sectional area at the medial joint level was preoperatively 0.13 ± 0.07 cm2 and at 1, 3, and 6 months after harvesting was 0.28 ± 0.11 cm2, 0.16 ± 0.09 cm2, and 0.14 ± 0.07 cm2, respectively (Fig. 5). In terms of the cross-sectional area of regenerated tissue, the preoperative value was not significantly different from the value at 6 months after harvesting (p = 0.436) contrary to the values at 1 and 3 months after harvesting (p < 0.001 and p = 0.044, respectively) as the echogenicity of the regenerated tissue was similar to that of the normal ST (Figs. 4 and 5). Even though no significant differences in functional performance tests were noted between regeneration and no-regeneration groups, the regenerated group tended to show better results, especially in the co-contraction test (Table 8).
The principal findings of the current study were that single ST harvesting showed significantly lower deep flexor strength deficit compared with ST-G harvesting, and the S group tended to show better clinical results at the last follow-up. In the S group, more than 80% showed good regeneration at the 6-month follow-up. Hence, single ST harvesting is effective in minimizing such flexor weakness and functional deficits showing great potential for regeneration. Moreover, proximal shift of musculotendinous junction, which showed moderate correlation with deep flexor strength deficit, was significantly greater in the D group, although the regeneration rates of tendon were high in both groups.
Several studies reported weakness of flexor strength and internal rotational strength after hamstring tendon harvesting, especially when both ST and G were harvested.219) Flexor strength weakness after hamstring harvesting was more prominent at deep flexion angles than at standard flexion angles. Nakamura et al.19) showed that flexor strength of the involved knee was restored less effectively at 90° of deep flexion than at the angle of peak torque generation. In their study, the LSI was 80.2% at deep flexion and 93.7% at the angle of peak torque generation in single ST harvesting, and the value was 78.8% at deep flexion and 91.3% at the angle of peak torque generation in ST-G harvesting. Tashiro et al.20) demonstrated that deep flexor strength at 70° or more was significantly decreased in both single ST harvesting and ST-G harvesting, excluding compensation by semimembranosus and biceps femoris, and ST-G harvesting resulted in considerably less strength than single ST harvesting at 18 months. They concluded that G preservation minimized weakness of deep flexor strength after hamstring harvesting. Our results support their findings. We performed the isokinetic deep flexor strength test in prone position at angles between 60° and 120°, where the ST and G play a main role, contrary to the standard flexion angles, at which point the semimembranosus and biceps femoris compensate making it difficult to assess the precise hamstring strength.1221) The present study demonstrated that the S group showed significantly less deep flexor strength deficits than the D group, whereas both groups showed no significant differences in extensor and standard flexor strength deficits, although the mean values of extensor and standard flexor strength deficits were lower in the S group than in the D group. Tadokoro et al.22) suggested that residual hamstring weakness at minimum 2 years of follow-up usually did not appear as a significant functional deficit in daily activities, however, certain sports activities such as wrestling and judo, which require deep flexor strength, might be considerably affected.
In our study, deep flexor strength deficits showed mild positive correlation with shift of musculotendinous junction of ST in the co-contraction test and moderate positive correlation on MRI. Meanwhile, extensor strength deficits and standard flexor strength deficits revealed moderate to strong positive or negative correlations with the mean time in the co-contraction test and Carioca test and LSI for SLHD. The co-contraction test was designed to reproduce the tibial rotational force necessitating control of tibial translation by the thigh musculature and requires deep flexor strength partially.16) In the current study, there was significant difference in the co-contraction test between both groups (p = 0.012). The ST is similar to the G in the shape of tendon fibers, length, and direction of tendon, whereas different from the biceps femoris and semimembranosus; thus, if a ST is sacrificed, the G can effectively compensate the deficit of flexor strength, especially at deep angles, compared to the biceps femoris and semimembranosus.1221) Therefore, we harvested the single ST to minimize the deficit of flexor strength, especially the deep flexor strength.
In the current study, the regeneration rate on MRI at 12 months after harvesting of the ST was 85% in the S group and 81.7% in the D group, and G was regenerated in 76.7% of the D group. Further, the preserved G served as a scaffold for the distal part of the regenerated ST. In our study, the mean shift of distal insertion of ST was −0.5 ± 0.7 cm in the S group and 0.3 ± 0.9 cm in the D group, showing significant difference. Our results were similar to previous studies, which demonstrated the regenerated tendon inserted at the pes anserinus in an almost anatomic position.1323) In addition, in serial ultrasonographic evaluation of the S group, the regeneration rates at the medial joint line and distal insertion below the joint line were 76.7% and 73.3%, respectively, at 3 months after harvesting and 81.7% and 80%, respectively, at 6 months after harvesting. At 1 month after harvesting, hematoma-like soft tissue mass was found in 43 (71.7%). The hematoma contains a high concentration of platelets, growth factors, and fibrin, which promotes vasculogenesis, fibroblast activation, and proliferation, and acts as a scaffold for mesenchymal stem cells, which differentiates into tenocytes.24) Tendon regeneration has been postulated to occur from an extrasynovial hematoma in the harvested tendon canal because hematoma acts as a scaffold for fibroblasts to invade and promote the mechanism for collagen production and tendon regeneration.13) Recently, Suydam et al.25) demonstrated ST regeneration and recovery of functional biomechanical properties including shear elastic and viscous moduli with time using imaging and elastography data after ST-G harvesting. They demonstrated that the elastic modulus of the regenerated ST potentially transmitted muscle force across the joint and restored ST function. In our study, however, there were no significant differences in functional performance tests between the regeneration and no-regeneration groups after single ST harvesting; the regenerated group tended to show better results, especially in the co-contraction test. Nevertheless, the relationship between isokinetic muscle strength and status of tendon regeneration has not been fully understood, and we anticipate future studies to elucidate it clearly.1222)
Konrath et al.9) evaluated morphological characteristics and hamstring strength at 2 years after hamstring harvesting and concluded that the muscle-tendon properties of the ST and G were substantially altered and these alterations contributed to flexor weakness. They showed that the regenerated ST and G were on average 3.3 cm and 2.9 cm longer than in the uninvolved knee, and the deficit of ST and G muscles was significantly correlated with flexor strength deficit. Nakamae et al.26) reported that proximal shift of musculotendinous junction of ST was about 7 cm at 6 months after harvesting, with no further retraction at 12 months. Choi et al.14) showed that musculotendinous junction shifted proximally by a mean of 4.39 ± 2.24 cm for ST tendon and 3.09 ± 2.22 cm for G, and it significantly correlated with isokinetic deep flexor strength in prone position. In our study, the musculotendinous junction of ST shifted proximally by 3.8 ± 2.1 cm and 4.2 ± 2.2 cm in the S group and D group, respectively. It showed moderate correlation with deep flexor strength deficit. We suggest that the magnitude of retraction of musculotendinous junction during hamstring regeneration influences flexor strength restoration and functional performance recovery.
The equivalent results on second-look arthroscopy at minimum 24 months after ACL reconstruction were meaningful in that even though the single ST was harvested, graft tension and synovial coverage were not inferior compared to ST-G harvesting. We performed the same fixation method using the suspensory system and a biocross pin in both groups because our goal was to achieve secure graft fixation and early rehabilitation. This dual fixation could overcome the possibility of weak fixation related with the relative short graft length in bone tunnels in single ST harvesting.1011) Moreover, previous biomechanical studies revealed that the acceptable intra-tunnel graft length was at least 15 mm to obtain optimal strength and stiffness of the ACL graft.2728)
We conducted a controlled trial and a comparative study of 120 patients. Of the 583 patients, 60 who underwent ACL reconstruction using ST-G from June 2009 to June 2012 and 60 consecutive patients who underwent ACL reconstruction using ST from July 2012 to March 2014 were included. All the eligible patients underwent follow-up MRI along with second-look arthroscopy postoperatively. Functional tests were conducted at a minimum of 36 months after primary ACL reconstruction. Hence, a comprehensive evaluation of morphological properties of tendon regeneration and clinical and functional outcomes was performed.
Several limitations of the current study should be noted. First, selection bias may have affected our study results because we included only patients who underwent MRI, second-look arthroscopy, and functional tests. However, although we did not conduct a prospective randomized trial, we tried to maintain even allocation of the patients and proceed in a prospective consecutive manner. Second, the mean 1-year follow-up might not be long enough to identify the regeneration of graft after harvesting on MRI. Third, we could not measure internal rotation strength. It would be helpful if the motion of ankle and hip joints, which affect the results of internal rotation strength, was controlled appropriately. Forth, the US examination was conducted in only the S group. The D group was included from June 2009 to June 2012 and the S group was included from July 2012 to March 2014. We have conducted the US examination since July 2012 after single ST harvesting. Finally, personal variation in the rehabilitation status after harvesting was also a limitation despite our efforts to standardize the protocol.
The S group showed significantly less deficit in deep flexor strength and tended to show better clinical results at the last follow-up than the D group. In the S group, more than 80% showed good regeneration at the 6-month follow-up. Hence, single ST harvesting is effective in minimizing such flexor weakness and functional deficits showing great potential for regeneration.
References
1. Chee MY, Chen Y, Pearce CJ, et al. Outcome of patellar tendon versus 4-strand hamstring tendon autografts for anterior cruciate ligament reconstruction: a systematic review and meta-analysis of prospective randomized trials. Arthroscopy. 2017; 33(2):450–463. PMID: 28040335.


2. Kim JG, Yang SJ, Lee YS, Shim JC, Ra HJ, Choi JY. The effects of hamstring harvesting on outcomes in anterior cruciate ligament-reconstructed patients: a comparative study between hamstring-harvested and -unharvested patients. Arthroscopy. 2011; 27(9):1226–1234. PMID: 21875529.


3. Yosmaoglu HB, Baltaci G, Ozer H, Atay A. Effects of additional gracilis tendon harvest on muscle torque, motor coordination, and knee laxity in ACL reconstruction. Knee Surg Sports Traumatol Arthrosc. 2011; 19(8):1287–1292. PMID: 21298255.


4. Janssen RP, van der Velden MJ, Pasmans HL, Sala HA. Regeneration of hamstring tendons after anterior cruciate ligament reconstruction. Knee Surg Sports Traumatol Arthrosc. 2013; 21(4):898–905. PMID: 22763570.


5. Sharma A, Flanigan DC, Randall K, Magnussen RA. Does gracilis preservation matter in anterior cruciate ligament reconstruction? A systematic review. Arthroscopy. 2016; 32(6):1165–1173. PMID: 26874800.


6. Karimi-Mobarakeh M, Mardani-Kivi M, Mortazavi A, Saheb-Ekhtiari K, Hashemi-Motlagh K. Role of gracilis harvesting in four-strand hamstring tendon anterior cruciate ligament reconstruction: a double-blinded prospective randomized clinical trial. Knee Surg Sports Traumatol Arthrosc. 2015; 23(4):1086–1091. PMID: 24531357.


7. Stevanovic V, Blagojevic Z, Petkovic A, et al. Semitendinosus tendon regeneration after anterior cruciate ligament reconstruction: can we use it twice. Int Orthop. 2013; 37(12):2475–2481. PMID: 23982635.
8. Bedi A, Srinivasan RC, Salata MJ, Downie B, Jacobson JA, Wojtys EM. Structural and functional analysis of the semitendinosus tendon after harvest for soft tissue reconstructive procedures: a dynamic ultrasonographic study. Knee Surg Sports Traumatol Arthrosc. 2013; 21(3):606–614. PMID: 22476527.


9. Konrath JM, Vertullo CJ, Kennedy BA, Bush HS, Barrett RS, Lloyd DG. Morphologic characteristics and strength of the hamstring muscles remain altered at 2 years after use of a hamstring tendon graft in anterior cruciate ligament reconstruction. Am J Sports Med. 2016; 44(10):2589–2598. PMID: 27432052.


10. Lee DW, Kim JG. Anatomic single-bundle anterior cruciate ligament reconstruction using the modified transtibial technique. Arthrosc Tech. 2017; 6(1):e227–e232. PMID: 28409105.


11. Lee DW, Kim JG, Lee JH, Park JH, Kim DH. Comparison of modified transtibial and outside-in techniques in anatomic single-bundle anterior cruciate ligament reconstruction. Arthroscopy. 2018; 34(10):2857–2870. PMID: 30197202.


12. Carofino B, Fulkerson J. Medial hamstring tendon regeneration following harvest for anterior cruciate ligament reconstruction: fact, myth, and clinical implication. Arthroscopy. 2005; 21(10):1257–1265. PMID: 16226656.


13. Eriksson K, Larsson H, Wredmark T, Hamberg P. Semitendinosus tendon regeneration after harvesting for ACL reconstruction: a prospective MRI study. Knee Surg Sports Traumatol Arthrosc. 1999; 7(4):220–225. PMID: 10462211.
14. Choi JY, Ha JK, Kim YW, Shim JC, Yang SJ, Kim JG. Relationships among tendon regeneration on MRI, flexor strength, and functional performance after anterior cruciate ligament reconstruction with hamstring autograft. Am J Sports Med. 2012; 40(1):152–162. PMID: 21993976.


15. Noyes FR, Barber SD, Mangine RE. Abnormal lower limb symmetry determined by function hop tests after anterior cruciate ligament rupture. Am J Sports Med. 1991; 19(5):513–518. PMID: 1962720.


16. Jang SH, Kim JG, Ha JK, Wang BG, Yang SJ. Functional performance tests as indicators of returning to sports after anterior cruciate ligament reconstruction. Knee. 2014; 21(1):95–101. PMID: 24075101.
17. Guo L, Chen H, Luo JM, Yang L, Gu LC, Fu DJ. An arthroscopic second-look study on the effect of remnant preservation on synovialization of bone-patellar tendonbone allograft in anterior cruciate ligament reconstruction. Arthroscopy. 2016; 32(5):868–877. PMID: 26821962.


18. Noh JH, Yang BG, Roh YH, Lee JS. Synovialization on second-look arthroscopy after anterior cruciate ligament reconstruction using Achilles allograft in active young men. Knee Surg Sports Traumatol Arthrosc. 2011; 19(11):1843–1850. PMID: 21409462.


19. Nakamura N, Horibe S, Sasaki S, et al. Evaluation of active knee flexion and hamstring strength after anterior cruciate ligament reconstruction using hamstring tendons. Arthroscopy. 2002; 18(6):598–602. PMID: 12098120.


20. Tashiro T, Kurosawa H, Kawakami A, Hikita A, Fukui N. Influence of medial hamstring tendon harvest on knee flexor strength after anterior cruciate ligament reconstruction: a detailed evaluation with comparison of single- and double-tendon harvest. Am J Sports Med. 2003; 31(4):522–529. PMID: 12860539.


21. Ohkoshi Y, Inoue C, Yamane S, Hashimoto T, Ishida R. Changes in muscle strength properties caused by harvesting of autogenous semitendinosus tendon for reconstruction of contralateral anterior cruciate ligament. Arthroscopy. 1998; 14(6):580–584. PMID: 9754475.


22. Tadokoro K, Matsui N, Yagi M, Kuroda R, Kurosaka M, Yoshiya S. Evaluation of hamstring strength and tendon regrowth after harvesting for anterior cruciate ligament reconstruction. Am J Sports Med. 2004; 32(7):1644–1650. PMID: 15494328.


23. Eriksson K, Kindblom LG, Hamberg P, Larsson H, Wredmark T. The semitendinosus tendon regenerates after resection: a morphologic and MRI analysis in 6 patients after resection for anterior cruciate ligament reconstruction. Acta Orthop Scand. 2001; 72(4):379–384. PMID: 11580127.


24. Thaker H, Sharma AK. Engaging stem cells for customized tendon regeneration. Stem Cells Int. 2012; 2012:309187. PMID: 22685473.


25. Suydam SM, Cortes DH, Axe MJ, Snyder-Mackler L, Buchanan TS. Semitendinosus tendon for ACL reconstruction: regrowth and mechanical property recovery. Orthop J Sports Med. 2017; 5(6):2325967117712944. PMID: 28680900.


26. Nakamae A, Deie M, Yasumoto M, et al. Three-dimensional computed tomography imaging evidence of regeneration of the semitendinosus tendon harvested for anterior cruciate ligament reconstruction: a comparison with hamstring muscle strength. J Comput Assist Tomogr. 2005; 29(2):241–245. PMID: 15772546.
27. Yuan F, Zhou W, Cai J, Zhao J, Huangfu X, Yin F. Optimal graft length for anterior cruciate ligament reconstruction: a biomechanical study in beagles. Orthopedics. 2013; 36(5):e588–e592. PMID: 23672910.


28. Qi L, Chang C, Jian L, Xin T, Gang Z. Effect of varying the length of soft-tissue grafts in the tibial tunnel in a canine anterior cruciate ligament reconstruction model. Arthroscopy. 2011; 27(6):825–833. PMID: 21624677.


Fig. 1
Flowchart of inclusion and exclusion of participants. ST-G: semitendinosus-gracilis tendon, ST: semitendinosus tendon.
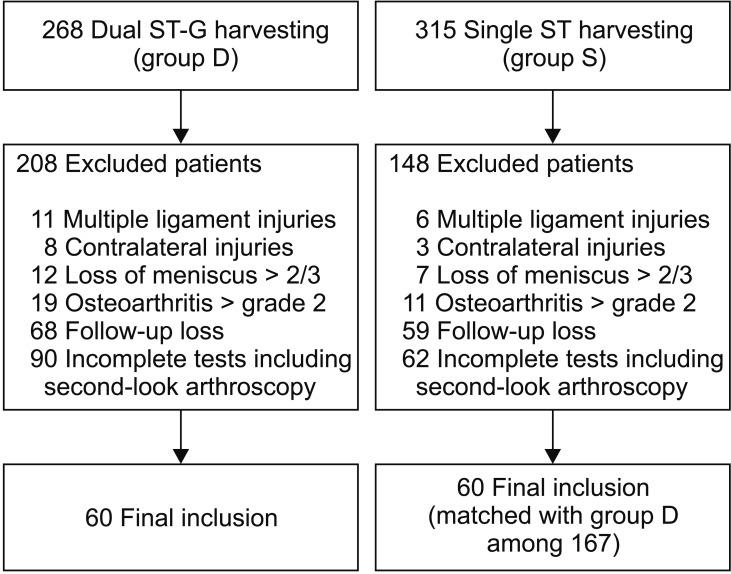
Fig. 2
The shift of the musculotendinous junction is evaluated on the sagittal plane. (A, B) Sagittal magnetic resonance imaging showing the musculotendinous junction (black arrows). The regenerated semitendinosus tendon (B) is more proximally shifted than the preoperative semitendinosus tendon (A).
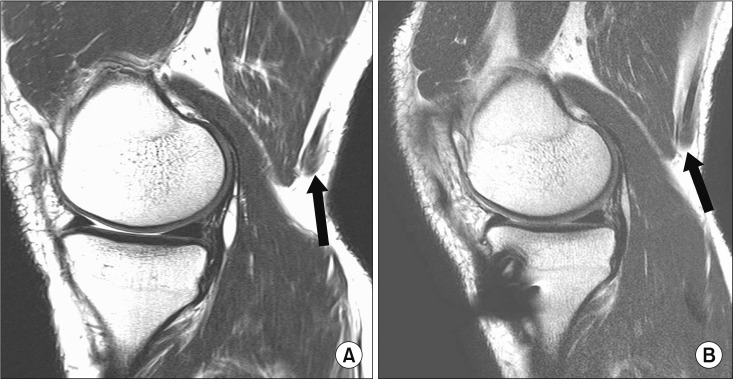
Fig. 3
The shift of the distal insertion (black arrows) of the regenerated tendon is measured in the coronal plane. Both preoperative tendon insertion (A) and regenerated tendon insertion (B) are identified and compared.
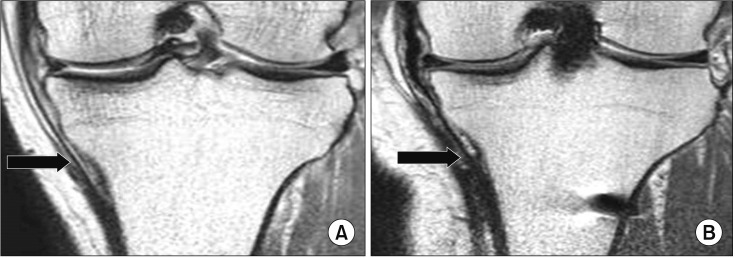
Fig. 4
Longitudinal ultrasonography of a semitendinosus (ST) tendon (white arrows) performed preoperatively (A), 2 days after tendon harvesting (absence of ST tendon) (B), 1 month after tendon resection (C), 3 months after harvesting (D), and 6 months after harvesting (E).

Fig. 5
Graph showing the cross-sectional area of the semitendinosus tendon at the medial joint level at preoperative and 1, 3, and 6 months of follow-up. The preoperative value of the cross-sectional area of the regenerated tissue is not significantly different from the value at 6 months after harvesting (p = 0.436) contrary to the values at 1 and 3 months after harvesting (p < 0.001 and p = 0.044, respectively). Postop: postoperative.
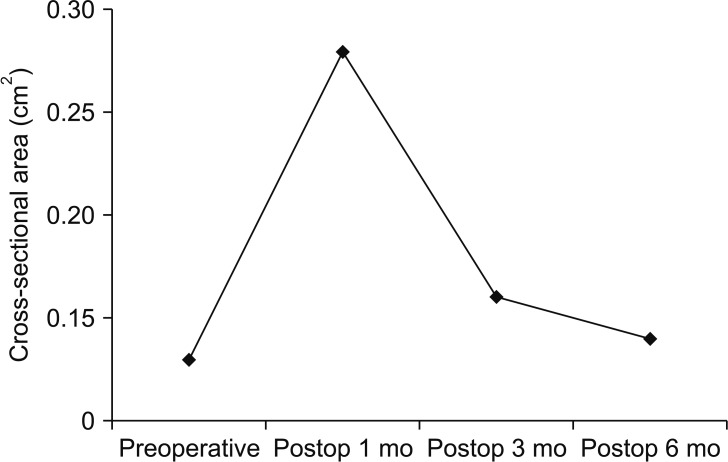
Table 1
Demographic Data and Clinical Results
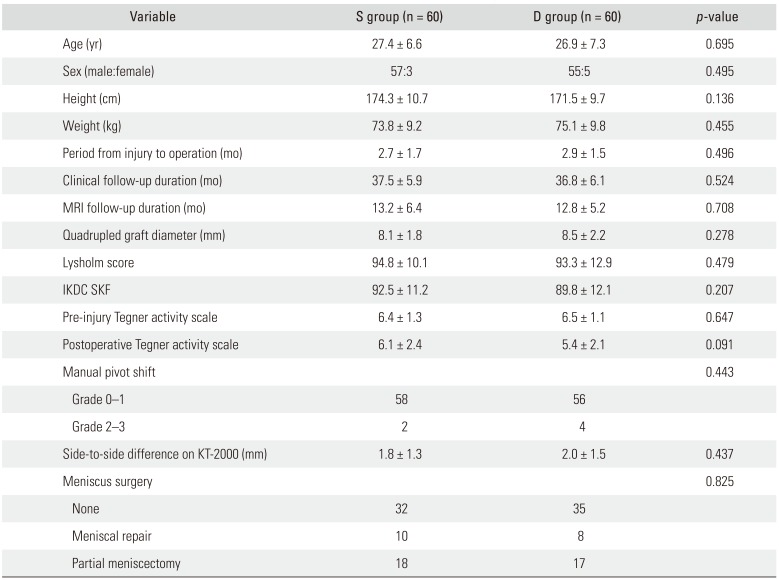
Table 2
Comparison of Tendon Regeneration Characteristics with Magnetic Resonance Imaging at 12 Months after Harvesting between Both Groups
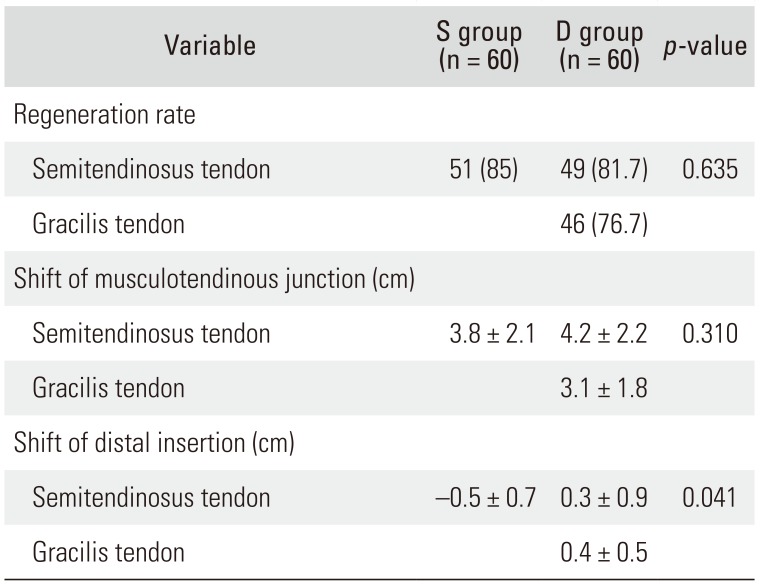
Table 3
Comparison of Isokinetic Muscle Strength Test Results between Groups
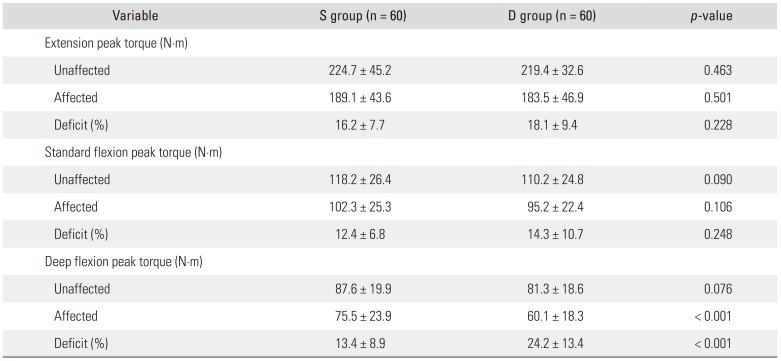
Table 4
Comparison of Functional Performance Test Results between Groups
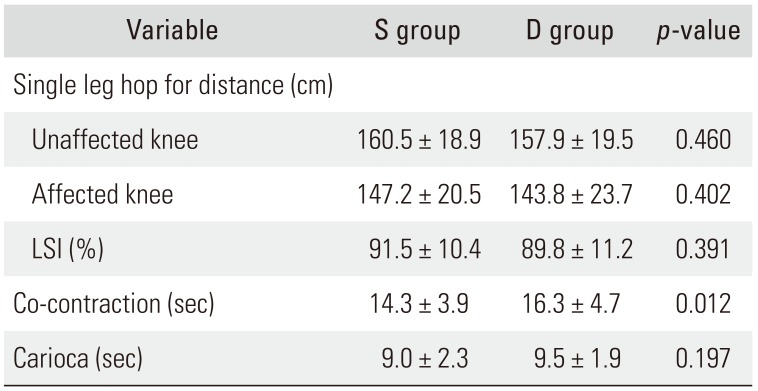
Table 5
Correlation Analysis of Deficit of Isokinetic Strength Tests and Functional Performance Tests, and Characteristics on Magnetic Resonance Imaging
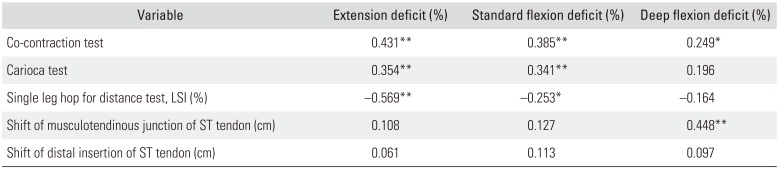
Table 6
Comparison of Graft Tension and Synovial Coverage between Groups on Second-Look Arthroscopy
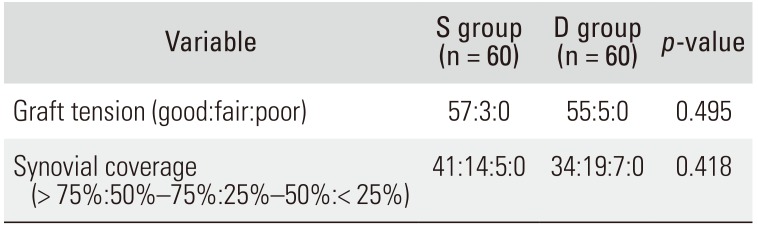
Variable | S group (n = 60) | D group (n = 60) | p-value |
---|---|---|---|
Graft tension (good:fair:poor) | 57:3:0 | 55:5:0 | 0.495 |
Synovial coverage (> 75%:50%–75%:25%–50%:<25%) | 41:14:5:0 | 34:19:7:0 | 0.418 |
Table 7
Results of Serial Ultrasonography of S group (n = 60)
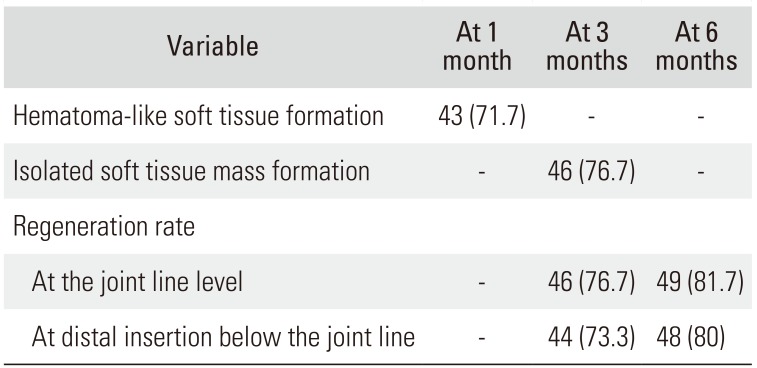
Table 8
Comparison of Clinical Results between Regeneration and No-regeneration Groups in the Single Semitendinosus Tendon Harvesting Group at 6 Months after Harvesting
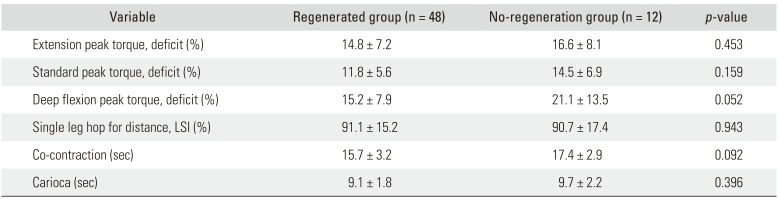