Abstract
Mannosylerythritol lipids (MELs) are glycolipids and have several pharmacological efficacies. MELs also show skin-moisturizing efficacy through a yet-unknown underlying mechanism. Aquaporin-3 (AQP3) is a membrane protein that contributes to the water homeostasis of the epidermis, and decreased AQP3 expression following ultraviolet (UV)-irradiation of the skin is associated with reduced skin moisture. No previous study has examined whether the skin-moisturizing effect of MELs might act through the modulation of AQP3 expression. Here, we report for the first time that MELs ameliorate the UVA-induced downregulation of AQP3 in cultured human epidermal keratinocytes (HaCaT keratinocytes). Our results revealed that UVA irradiation decreases AQP3 expression at the protein and messenger RNA (mRNA) levels, but that MEL treatment significantly ameliorated these effects. Our mitogen-activated protein kinase inhibitor analysis revealed that phosphorylation of c-Jun N-terminal kinase (JNK), but not extracellular signal-regulated kinase or p38, mediates UVA-induced AQP3 downregulation, and that MEL treatment significantly suppressed the UVA-induced phosphorylation of JNK. To explore a possible mechanism, we tested whether MELs could regulate the expression of peroxidase proliferator-activated receptor gamma (PPAR-γ), which acts as a potent transcription factor for AQP3 expression. Interestingly, UVA irradiation significantly inhibited the mRNA expression of PPAR-γ in HaCaT keratinocytes, whereas a JNK inhibitor and MELs significantly rescued this effect. Taken together, these findings suggest that MELs ameliorate UVA-induced AQP3 downregulation in HaCaT keratinocytes by suppressing JNK activation to block the decrease of PPAR-γ. Collectively, our findings suggest that MELs can be used as a potential ingredient that modulates AQP3 expression to improve skin moisturization following UVA irradiation-induced damage.
Mannosylerythritol lipids (MELs) are extracellular glycolipids that are produced from different vegetable oil substrates by the yeast strains of genus Pseudozyma [1]. MELs comprise a hydrophilic headgroup containing 4-O-β-D-mannopyranosyl erythritol or 1-O-β-D-mannopyranosyl erythritol and fatty acid-containing hydrophobic chains [1]. MELs were originally developed as a highly biodegradable biosurfactant [2], but were subsequently found to have additional biological properties such as anti-inflammation and apoptosis of melanoma cells [34567]. As the possible applications of MELs expanded, some researchers focused on using MELs as a cosmetic ingredient; to date, several studies have found that MELs can moisturize the skin. Morita et al. [89] reported that MELs exerted recovery effect on the viability of sodium dodecyl sulfate (SDS)-damaged human skin cells in a three-dimensional skin equivalent model. In addition, Yamamoto et al. [10] reported that topical application of MELs to human forearm skin resulted in increased level of water-retention and decreased level of transepidermal water loss for approximately 2 h.
Aquaporin-3 (AQP3) contributes to water homeostasis in the epidermis and is responsible for transporting water and glycerol at the plasma membranes of keratinocytes in the epidermal basal layer [111213]. Decreased AQP3 expression in the skin is associated with dry skin, reduced elasticity, decreased glycerol levels, and impaired wound healing with defective barrier function [1314]. AQP3 expression in the skin naturally declines with age [15], but it may also be reduced by harmful external stimuli, such as ultraviolet (UV) irradiation and reactive oxygen species [16]. Numerous studies have sought to identify materials that may ameliorate the UV irradiation-induced downregulation of AQP3 expression and to evaluate the possible mechanisms underlying such effects [16171819].
The mitogen-activated protein kinase (MAPK) pathways, namely the extracellular signal-regulated kinase (ERK), c-Jun N-terminal kinase (JNK), and p38 pathways, play important roles in regulating and eliciting various cellular responses, such as proliferation, differentiation, development, transformation, and apoptosis [20]. In epidermal keratinocytes, a diverse range of extracellular stimuli, including UV radiation, strongly induce the phosphorylation of MAPKs [161718]. AQP3 expression is reportedly regulated by the MAPK pathways [1621] as well as by the transcription factor, peroxidase proliferator-activated receptor gamma (PPAR-γ), which may be modulated by MAPKs or UV irradiation [222324].
To our knowledge, no previous study had examined whether MELs are involved in modulating AQP3 expression in UV-irradiated keratinocytes. Here, we evaluated whether MELs can ameliorate UVA-induced AQP3 downregulation in cultured human keratinocytes, and then explored a possible underlying mechanism.
The MELs used in the present study were produced and provided by DKBIO (Daejeon, Korea). Briefly, MELs were synthesized in a yeast strain of Pseudozyma sp., extracted from the culture broth with ethyl acetate, and purified through a fatty acid removal process. Purified MELs were dissolved with dimethyl sulfoxide for use in the experiments. MELs are mixtures consisting of MEL-A, MEL-B, MEL-C, and MEL-D. The MELs used in this study are composed of predominant MEL-B with a purity of about 95% and their molecular weight range is 500 to 850. MAPK inhibitors were purchased from Tocris (#1879; Minneapolis, MN, USA) and used at concentrations selected with reference to previous studies (100 nM SP600125 for JNK, 10 µM PD98059 for ERK, and 5 µM SB203580 for p38) [1625].
HaCaT keratinocytes (an immortalized cell line derived from normal human keratinocytes) were purchased from the American Type Culture Collection (Manassas, VA, USA) and cultured in Dulbecco's modified Eagle's medium (DMEM; 12-604F, Lonza) supplemented with 10% fetal bovine serum (#10082-147; Thermo Fisher Scientific, Waltham, MA, USA), 100 U/ml potassium penicillin, and 100 mg/ml streptomycin sulfate (17-602E; Lonza, Basel, Switzerland) at 37℃ in a humidified incubator containing 5% CO2. The cells were washed with phosphate-buffered saline (21-031-CVR; Corning, Corning, NY, USA), detached with Accutase (SCR005; EMD Millipore, Burlington, MA, USA), and plated to 60-mm dishes at 0.3 × 106 cells/well in fresh culture medium. Cells grown to 70%–80% confluence were serum-starved for 4 h and then treated with the indicated concentrations of reagents.
UVA irradiation (365 nm) was performed using a microprocessor-controlled, cooled UV irradiation system (Bio-Sun UVH; Vilber Lourmat, Collégien, France). As previously described [26], cells were covered with serum and phenol red-free DMEM (#21063-029; Thermo Fisher Scientific) during irradiation. After irradiation, cells were returned to fresh DMEM (12-604F; Lonza) for culture.
Cell viability was determined using a Cell Counting Kit-8 (CCK-8) (CK04-05; Dojindo, Kumamoto, Japan). HaCaT keratinocytes seeded to a 96-well plate at a density of 5 × 103 cells/well were incubated with MELs for 24 h. Then, 10 µl of CCK-8 solution and 90 µl of DMEM (#31053-028; Thermo Fisher Scientific) were added to each well and the plate was incubated at 37℃ for 2 h. The absorbance was measured at 450 nm using a microplate reader (Synergy2; BioTek, Winooski, VT, USA).
Total RNA was extracted using the RNeasy Mini Kit (#74101; Qiagen, Venlo, the Netherlands). One microgram of RNA was reverse transcribed into complementary DNA (cDNA) using a SuperScript III First-Strand Synthesis System according to the manufacturer's instructions (#18080-051; Invitrogen, Carlsbad, CA, USA). For qRT-PCR, we used an ABI 7500 Fast Real-Time PCR system (Applied Biosystems, Foster City, CA, USA) with the TaqMan Gene Expression Master Mix (#4369016; Thermo Fisher Scientific) and pre-inventoried TaqMan primers for AQP3 (Hs00185020_m1), PPAR-γ (Hs01115513_m1), and glyceraldehyde-3-phosphate dehydrogenase (GAPDH, detected as a normalization control for cDNA quantity; Hs02786624_g1). The level of relative messenger RNA (mRNA) expression was calculated using the comparative ΔΔCt method [27].
Cells were lysed using RIPA Lysis and Extraction Buffer (#89900; Thermo Fisher Scientific) containing a complete mini protease inhibitor (#11836153001; Roche, Basel, Switzerland) and a phosphatase inhibitor cocktail (P5726; Sigma-Aldrich, St. Louis, MO, USA). The cell lysates were centrifuged for 20 min at 13,000 rpm and 4℃, and the supernatants were collected for analysis. Protein concentrations were determined using a BCA protein assay kit (#23225; Thermo Fisher Scientific). Equal amounts of protein (30 µg/well) were loaded to a 4%–12% gradient Bis-Tris gel (Nu PAGE; Invitrogen), separated by SDS-PAGE, and transferred to a nitrocellulose membrane (LC2000; Invitrogen). The membrane was blocked with TBST buffer (0.2% Tween 20 in Tris-buffered saline) containing 5% bovine serum albumin at room temperature for 1 h and probed overnight at 4℃ with each of the following primary antibodies: AQP3 (ab125129; Abcam, Cambridge, UK), PPAR-γ (NBP1-61399; Novus, Centennial, CO, USA), GAPDH (#2118; Cell Signaling Technology [CST], Danvers, MA, USA), JNK (#9252; CST), p-JNK (#4668; CST), ERK (#4695; CST), p-ERK (#4370; CST), p38 (#8690; CST), and p-p38 (#4511; CST). After three washes with TBST, the membrane was incubated with horseradish peroxidase-conjugated secondary antibodies at room temperature for 1 h. Chemiluminescent signals were detected with an ECL substrate (RPN2232; GE Healthcare, Hammersmith, UK) and visualized using a chemiluminescent detection system (LAS-3000; Fuji Film, Tokyo, Japan).
Prior to define the effect of MELs on UVA-induced AQP3 downregulation, we decided to determine the optimal dose of UVA for significantly downregulating AQP3 expression in our experimental system. First, we irradiated HaCaT keratinocytes with various doses of UVA (0.5, 1, 2, or 3 J/cm2) and analyzed the protein level of AQP3 after 24 h (Fig. 1A). The AQP3 protein level was dose-dependently reduced under UVA irradiation, and significantly decreased to 50% of the level seen in the UVA non-irradiation control group under 3 J/cm2 of UVA irradiation (UVA 3 J/cm2) (Fig. 1B). Similarly, qRT-PCR revealed that the mRNA expression of AQP3 was significantly reduced to 54% of the control level at 24 h after irradiation with UVA 3 J/cm2. A time-course experiment revealed that a significant decrease in AQP3 mRNA expression could be detected as early as 6 h after UVA irradiation, and this level continued to decrease gradually until 24 h post-irradiation (Fig. 1C). Since cell viability was not significantly different from the control group at 24 h after UVA 3 J/cm2 irradiation (Fig. 1D), we subsequently used UVA 3 J/cm2 to significantly reduce AQP3 expression in HaCaT keratinocytes.
Next, we examined whether MELs (Fig. 2A) could ameliorate the UVA-induced downregulation of AQP3 expression. Noncytotoxic concentrations of MELs (0.25, 1, and 4 µg/ml) were selected based on the results of our cell viability assay (Fig. 2B) and applied to cells for 24 h following UVA 3 J/cm2 irradiation. As shown in Fig. 2C, the UVA-induced downregulation of AQP3 protein expression was markedly and dose-dependently ameliorated by 1 and 4 µg/ml of MELs. These levels were increased by 124% and 142%, respectively, compared to the UVA 3 J/cm2-irradiated group (Fig. 2D). AQP3 mRNA expression was increased by 164% when UVA 3 J/cm2-irradiated cells were treated with 1 µg/ml MELs compared to those receiving UVA 3 J/cm2-irradiation alone (Fig. 2E). This indicates that MEL 1 µg/ml is sufficient to alleviate the UVA-induced reduction of AQP3.
Since the MAPK pathway is reportedly involved in modulating AQP3 expression [1621], we investigated whether UVA-induced AQP3 downregulation was associated with changes in the MAPKs, JNK, ERK, and p38 MAPK. Serum-starved HaCaT keratinocytes were harvested at 15 min and 30 min after UVA 3 J/cm2 irradiation. Our analyses revealed that the phosphorylation levels of the three MAPKs were significantly increased at both time points compared to those in non-irradiated cells (Fig. 3A). Next, we treated serum-starved cells with an inhibitor of each MAPK for 1 h followed by UVA 3 J/cm2 irradiation, and collected cell lysates 24 h later. We found that the UVA-induced downregulation of AQP3 protein expression was markedly ameliorated by the JNK inhibitor, which brought this level up to 138% that of the UVA 3 J/cm2-irradiated group (Fig. 3B). Similarly, the mRNA expression level of AQP3 was 136% higher in the JNK inhibitor-treated group than in the UVA 3 J/cm2-irradiated group (Fig. 3C). In contrast, the inhibitors of ERK or p38 failed to rescue the UVA-induced decrease of AQP3 protein expression (Fig. 3D).
Based on the above findings, we assessed the potential involvement of the MAPK pathway in the ability of MELs to ameliorate the UVA-induced downregulation of AQP3 expression. Serum-starved HaCaT keratinocytes were pretreated with 0.5 or 1 µg/ml MELs for 1 h, subjected to UVA 3 J/cm2 irradiation, and then harvested 15 m later. Indeed, we found that the UVA-induced upregulation of phosphorylated JNK was suppressed by MEL pretreatment, whereas the levels of phosphorylated ERK and p38 were unchanged (Fig. 4A). Interestingly, a few studies have reported the relationship between JNK activation and PPAR-γ expression [222324]. Particularly, JNK activation decreased PPAR-γ mRNA expression and subsequently led to AQP3 downregulation in hepatic stellate cells. These findings suggest the possibility that the MELs could up-regulate AQP3 expression through JNK inactivation and subsequent the increased PPAR-γ expression in UVA-irradiated HaCaT cells. Thus, we decided to focus on PPAR-γ expression after MELs treatment in UVA-irraidated HaCaT cells. In line with a previous study, we found that the mRNA expression of PPAR-γ was downregulated by UVA 3 J/cm2 irradiation in HaCaT keratinocytes; however, we observed for the first time that this phenomenon was significantly mitigated by MELs or the JNK inhibitor (Fig. 4B). Taken together, MELs ameliorated UVA-induced AQP3 downregulation through JNK inactivation and PPAR-γ up-regulation in cultured human keratinocytes (Fig. 4C).
AQP3 is well-known to be involved in skin hydration [28]. Thus, it seems logical that restoring a reduced AQP3 expression level (i.e., that triggered by environmental factors, such as UV irradiation) to its normal level could help improve skin hydration. Numerous studies have sought to identify materials that can effectively ameliorate UVB-induced AQP3 downregulation in HaCaT keratinocytes [16171819]. However, UVA irradiation can also induce photo-aging of skin [29] and was reported to reduce AQP3 expression in cultured human keratinocytes [26]. Hwang and Shim [26] showed that AQP3 mRNA expression was significantly downregulated in UVA 5 J/cm2-irradiated normal human epidermal keratinocytes, but the authors did not examine any potential underlying mechanism. In the present study, we confirm that UVA irradiation markedly reduces AQP3 expression at both the protein and mRNA levels in HaCaT keratinocytes. Furthermore, we demonstrate that, of the tested MAPK inhibitors, only the JNK inhibitor ameliorates the UVA-induced downregulation of AQP3 in our system. This suggests that the UVA-activated JNK pathway may be an underlying mechanism through which this irradiation downregulates AQP3. The UVB-induced downregulation of AQP3 expression has been linked with activation of the ERK or p38 pathways [161718]. These reports found that a JNK inhibitor did little to mitigate the UVB-induced downregulation of AQP3. Although further systematic investigations are warranted, the previous and present findings suggest that the MAPK involved in reducing AQP3 expression in UV-irradiated keratinocytes may differ by the type of UV irradiation.
Various biological effects have been reported for MELs, such as induction of differentiation in mouse melanoma cells and rat pheochromocytoma cells [45], enhancement of plasmid DNA transfection efficiency [7], and anti-inflammatory activity [3]. Thus, MELs are thought to be versatile candidates for use in various research and industrial fields. In addition, MELs may have potential for cosmetic use in the areas of skin care, damaged hair care, anti-oxidative effects, and facial color make-up [89103031]. Therefore, it is important to explore their yet-unidentified functions. The present study demonstrates a new biological function of MELs, in that they can alleviate the UVA-induced decrease of AQP3 expression in keratinocytes by suppressing JNK activation. We believe that our findings strengthen the value of MELs as a skin-moisturizing ingredient when combined with its previously reported efficacies in restoring damaged cells and augmenting water retention in the stratum corneum to hydrate dry skin [910]. Given that AQP3 expression was found to be downregulated by severe UVA irradiation of normal human skin fibroblasts [2132], future efforts are warranted to study the effects of MELs against the UVA-induced downregulation of AQP3 in human fibroblasts.
JNK is reportedly involved in regulating PPAR-γ expression. For example, Camp et al. [22] found that JNK activation reduced the effect of PPAR-γ ligands by decreasing PPAR-γ mRNA expression. Meanwhile, Tardelli et al. [24] found that activation of the JNK pathway suppressed PPAR-γ to downregulate AQP3 expression in hepatic stellate cells carrying the genetic polymorphism I148M of the patatin-like phospholipase domain-containing 3 (PNPLA3 I148M). In the abovementioned study, restoration of AQP3 expression was triggered by SP600125-mediated JNK inhibition and/or PPAR-γ stimulation [24]. These findings led us to investigate whether PPAR-γ expression was altered in our system. Consistent with a previous study showing that PPAR-γ mRNA expression was reduced in UVA-irradiated human adipose tissue-derived mesenchymal stem cells [23], we found that PPAR-γ mRNA expression was downregulated in UVA-irradiated HaCaT keratinocytes. Moreover, this downregulation of PPAR-γ mRNA expression was markedly counteracted by treatment with a JNK inhibitor or MELs. Based on our present findings, we propose that MELs suppress the UVA-induced phosphorylation of JNK, which prevents the UVA-induced decrease of PPAR-γ expression to ameliorate the reduction of AQP3 expression (Fig. 4C).
In summary, we herein show for the first time that MELs can ameliorate UVA-induced AQP3 downregulation in cultured human keratinocytes. We further reveal that UVA-induced AQP3 downregulation can be ameliorated via the ability of JNK to modulate PPAR-γ expression in cultured human keratinocytes. Collectively, our findings support the potential development of MELs for use in moisturizing skin that has been damaged by UVA irradiation.
Figures and Tables
Fig. 1
AQP3 expression is downregulated by UVA irradiation.
(A) HaCaT keratinocytes were irradiated with the indicated doses of UVA or left non-irradiated (control) and harvested after 24 h for Western blot analysis. (B) The protein level of AQP3 was quantified relative to that of GAPDH using the Image J software from National Institutes of Health. The results are presented as the mean expression level obtained from three independent experiments ± SD (**p < 0.01). (C) UVA 3 J/cm2-irradiated and non-irradiated control cells were harvested at the indicated time points, and qRT-PCR analysis of the AQP3 mRNA level was performed. The data were normalized with respect to the expression of GAPDH, and the results are presented as the mean expression ± SD (n = 3, **p < 0.01). (D) HaCaT keratinocytes were treated with the indicated doses of UVA and cell viability was determined using a CCK-8 kit (Dojindo, Kumamoto, Japan) 24 h after irradiation of indicated doses of UVA. The viability of cells treated with UVA 3 J/cm2 decreased to 90% without significance. AQP3, aquaporin-3; UVA, ultraviolet A; N.S., not significant; GAPDH, glyceraldehyde-3-phosphate dehydrogenase; SD, standard deviation; qRT-PCR, quantitative real-time polymerase chain reaction; mRNA, messenger RNA.
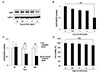
Fig. 2
MELs ameliorate the UVA-induced downregulation of AQP3 expression in HaCaT keratinocytes.
(A) A structure of MELs. Two fatty acids were attached to −OH groups on mannosylerythritol as an ester bond. The fatty acid contains mainly 6–18 carbon atoms and the major is 8 carbon atoms. (B) HaCaT keratinocytes were treated with the indicated concentrations of MELs for 24 h and cell viability was determined using a CCK-8 kit (Dojindo, Kumamoto, Japan). DMSO was used as the vehicle control. (C) Cells treated with UVA 3 J/cm2 and the indicated concentrations of MELs for 24 h were subjected to Western blot analysis of AQP3 proteins. (D) The protein levels of AQP3 were quantified relative to that of GAPDH using the Image J software. (E) The mRNA expression of AQP3 was analyzed by qRT-PCR. The results are presented as the mean expression level obtained from three independent experiments ± SD (**p < 0.01). MELs, mannosylerythritol lipids; UVA, ultraviolet A; AQP3, aquaporin-3; DMSO, dimethyl sulfoxide; GAPDH, glyceraldehyde-3-phosphate dehydrogenase; mRNA, messenger RNA; qRT-PCR, quantitative real-time polymerase chain reaction; SD, standard deviation.
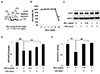
Fig. 3
Involvement of JNK phosphorylation in the UVA-induced downregulation of AQP3.
(A) Cells were irradiated with UVA 3 J/cm2 and harvested at the indicated time points. Protein samples were subjected to Western blotting for phospho-JNK, total JNK, phospho-ERK, total ERK, phospho-p38, total p38, and GAPDH. (B) Cells pretreated with 100 nM SP600125 (a JNK inhibitor) for 1 h were irradiated with UVA 3 J/cm2 and harvested after 24 h. The protein level of AQP3 was analyzed by Western blotting and quantified relative to the level of GAPDH using the ImageJ software. (C) The mRNA expression of AQP3 was examined by qRT-PCR. (D) Cells pretreated with 10 µM PD98059 (an ERK inhibitor) or 5 µM SB203580 (a p38 inhibitor) for 1 h were irradiated with UVA 3 J/cm2 and harvested after 24 h. The protein level of AQP3 was analyzed by Western blotting and quantified relative to the level of GAPDH using the ImageJ software. Values represent the mean expression level ± SD (n = 3, *p < 0.05, **p < 0.01). JNK, c-Jun N-terminal kinase; UVA, ultraviolet A; ERK, extracellular signal-regulated kinase; GAPDH, glyceraldehyde-3-phosphate dehydrogenase; AQP3, aquaporin-3; mRNA, messenger RNA; qRT-PCR, quantitative real-time polymerase chain reaction; SD, standard deviation.
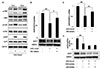
Fig. 4
The effects of MELs on JNK phosphorylation and PPAR-γ expression in UVA-irradiated HaCaT keratinocytes.
(A) Cells pretreated with the indicated concentrations of MELs for 1 h were irradiated with UVA 3 J/cm2 and harvested after 15 min. The protein levels of phospho-JNK, total JNK, phospho-ERK, total ERK, phospho-p38, total p38, and GAPDH were evaluated by Western blot analysis. (B) Cells treated with UVA 3 J/cm2 and the indicated concentrations of MELs or a JNK inhibitor for 16 h were prepared for qRT-PCR analysis of PPAR-γ mRNA expression. Values represent the mean expression level obtained from three independent experiments ± SD (*p < 0.05, **p < 0.01). (C) Proposed model showing how MELs ameliorate the UVA-induced downregulation of AQP3. MELs, mannosylerythritol lipids; JNK, c-Jun N-terminal kinase; PPAR-γ, peroxidase proliferator-activated receptor gamma; UVA, ultraviolet A; ERK, extracellular signal-regulated kinase; GAPDH, glyceraldehyde-3-phosphate dehydrogenase; qRT-PCR, quantitative real-time polymerase chain reaction; SD, standard deviation.
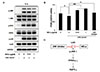
ACKNOWLEDGEMENTS
This research was supported and funded by Amorepacific R&D Center, and the Basic Science Research Program through the National Research Foundation of Korea (NRF) funded by the Ministry of Education (Grant Number: 2018R1D1A1B07049402).
Notes
Author contributions I.H.B., C.S.L., and D.Y.K. designed the study. I.H.B., S.H.L., S.O., H.W.C., P.A.M., J.W.Y., J.Y.K., and E.S.L. performed the experiments. I.H.B., T.R.L., C.S.L., and D.Y.K. analyzed the data and contributed to writing the manuscript. All authors read and approved the final version of the manuscript.
References
1. Morita T, Fukuoka T, Imura T, Kitamoto D. Mannosylerythritol lipids: production and applications. J Oleo Sci. 2015; 64:133–141.


2. Kitamoto D, Yanagishita H, Shinbo T, Nakane T, Kamisawa C, Nakahara T. Surface active properties and antimicrobial activities of mannosylerythritol lipids as biosurfactants produced by Candida antarctica. J Biotechnol. 1993; 29:91–96.


3. Morita Y, Tadokoro S, Sasai M, Kitamoto D, Hirashima N. Biosurfactant mannosyl-erythritol lipid inhibits secretion of inflammatory mediators from RBL-2H3 cells. Biochim Biophys Acta. 2011; 1810:1302–1308.


4. Zhao X, Wakamatsu Y, Shibahara M, Nomura N, Geltinger C, Nakahara T, Murata T, Yokoyama KK. Mannosylerythritol lipid is a potent inducer of apoptosis and differentiation of mouse melanoma cells in culture. Cancer Res. 1999; 59:482–486.

5. Wakamatsu Y, Zhao X, Jin C, Day N, Shibahara M, Nomura N, Nakahara T, Murata T, Yokoyama KK. Mannosylerythritol lipid induces characteristics of neuronal differentiation in PC12 cells through an ERK-related signal cascade. Eur J Biochem. 2001; 268:374–383.


6. Im JH, Yanagishita H, Ikegami T, Takeyama Y, Idemoto Y, Koura N, Kitamoto D. Mannosylerythritol lipids, yeast glycolipid biosurfactants, are potential affinity ligand materials for human immunoglobulin G. J Biomed Mater Res A. 2003; 65:379–385.


7. Ueno Y, Inoh Y, Furuno T, Hirashima N, Kitamoto D, Nakanishi M. NBD-conjugated biosurfactant (MEL-A) shows a new pathway for transfection. J Control Release. 2007; 123:247–253.


8. Morita T, Fukuoka T, Imura T, Kitamoto D. Production of mannosylerythritol lipids and their application in cosmetics. Appl Microbiol Biotechnol. 2013; 97:4691–4700.


9. Morita T, Kitagawa M, Suzuki M, Yamamoto S, Sogabe A, Yanagidani S, Imura T, Fukuoka T, Kitamoto D. A yeast glycolipid biosurfactant, mannosylerythritol lipid, shows potential moisturizing activity toward cultured human skin cells: the recovery effect of MELA on the SDS-damaged human skin cells. J Oleo Sci. 2009; 58:639–642.


10. Yamamoto S, Morita T, Fukuoka T, Imura T, Yanagidani S, Sogabe A, Kitamoto D, Kitagawa M. The moisturizing effects of glycolipid biosurfactants, mannosylerythritol lipids, on human skin. J Oleo Sci. 2012; 61:407–412.


11. Sugiyama Y, Ota Y, Hara M, Inoue S. Osmotic stress up-regulates aquaporin-3 gene expression in cultured human keratinocytes. Biochim Biophys Acta. 2001; 1522:82–88.


12. Hara-Chikuma M, Verkman AS. Aquaporin-3 functions as a glycerol transporter in mammalian skin. Biol Cell. 2005; 97:479–486.


13. Ma T, Hara M, Sougrat R, Verbavatz JM, Verkman AS. Impaired stratum corneum hydration in mice lacking epidermal water channel aquaporin-3. J Biol Chem. 2002; 277:17147–17153.


14. Hara M, Ma T, Verkman AS. Selectively reduced glycerol in skin of aquaporin-3-deficient mice may account for impaired skin hydration, elasticity, and barrier recovery. J Biol Chem. 2002; 277:46616–46621.


15. Li J, Tang H, Hu X, Chen M, Xie H. Aquaporin-3 gene and protein expression in sun-protected human skin decreases with skin ageing. Australas J Dermatol. 2010; 51:106–112.


16. Cao C, Wan S, Jiang Q, Amaral A, Lu S, Hu G, Bi Z, Kouttab N, Chu W, Wan Y. All-trans retinoic acid attenuates ultraviolet radiation-induced down-regulation of aquaporin-3 and water permeability in human keratinocytes. J Cell Physiol. 2008; 215:506–516.


17. Jeon BK, Kang MK, Lee GT, Lee KK, Lee HS, Woo WH, Mun YJ. EPA attenuates ultraviolet radiation-induced downregulation of aquaporin-3 in human keratinocytes. Arch Pharm Res. 2015; 38:1552–1560.


18. Ji C, Yang Y, Yang B, Xia J, Sun W, Su Z, Yu L, Shan S, He S, Cheng L, Wan Y, Bi Z. Trans-Zeatin attenuates ultraviolet induced down-regulation of aquaporin-3 in cultured human skin keratinocytes. Int J Mol Med. 2010; 26:257–263.


19. Shan SJ, Xiao T, Chen J, Geng SL, Li CP, Xu X, Hong Y, Ji C, Guo Y, Wei H, Liu W, Li D, Chen HD. Kanglaite attenuates UVB-induced down-regulation of aquaporin-3 in cultured human skin keratinocytes. Int J Mol Med. 2012; 29:625–629.


20. Zhang W, Liu HT. MAPK signal pathways in the regulation of cell proliferation in mammalian cells. Cell Res. 2002; 12:9–18.


21. Cao C, Sun Y, Healey S, Bi Z, Hu G, Wan S, Kouttab N, Chu W, Wan Y. EGFR-mediated expression of aquaporin-3 is involved in human skin fibroblast migration. Biochem J. 2006; 400:225–234.



22. Camp HS, Tafuri SR, Leff T. c-Jun N-terminal kinase phosphorylates peroxisome proliferator-activated receptor-gamma1 and negatively regulates its transcriptional activity. Endocrinology. 1999; 140:392–397.

23. Lee J, Lee J, Jung E, Kim YS, Roh K, Jung KH, Park D. Ultraviolet A regulates adipogenic differentiation of human adipose tissue-derived mesenchymal stem cells via up-regulation of Kruppel-like factor 2. J Biol Chem. 2010; 285:32647–32656.



24. Tardelli M, Bruschi FV, Claudel T, Moreno-Viedma V, Halilbasic E, Marra F, Herac M, Stulnig TM, Trauner M. AQP3 is regulated by PPARXMLLink_XYZ and JNK in hepatic stellate cells carrying PNPLA3 I148M. Sci Rep. 2017; 7:14661.



25. Silvers AL, Bachelor MA, Bowden GT. The role of JNK and p38 MAPK activities in UVA-induced signaling pathways leading to AP-1 activation and c-Fos expression. Neoplasia. 2003; 5:319–329.



26. Hwang HS, Shim JH. Brazilin and Caesalpinia sappan L. extract protect epidermal keratinocytes from oxidative stress by inducing the expression of GPX7. Chin J Nat Med. 2018; 16:203–209.


27. Livak KJ, Schmittgen TD. Analysis of relative gene expression data using real-time quantitative PCR and the 2−ΔΔCT method. Methods. 2001; 25:402–408.

28. Hara-Chikuma M, Verkman AS. Roles of aquaporin-3 in the epidermis. J Invest Dermatol. 2008; 128:2145–2151.


30. Morita T, Kitagawa M, Yamamoto S, Sogabe A, Imura T, Fukuoka T, Kitamoto D. Glycolipid biosurfactants, mannosylerythritol lipids, repair the damaged hair. J Oleo Sci. 2010; 59:267–272.

