Abstract
Sasa quelpaertensis Nakai leaves contain a mixture of polysaccharides, amino acids, and polyphenols, which mediate various biological activities. For efficient utilization of its leaf, we reported the preparation procedure for phytochemical-rich extract (PRE) using the leaf residue, which was by-product of hot water extraction. This study was undertaken to evaluate the effects of PRE and its major constituent, p-coumaric acid,on the growth of several human cancer cell lines (MKN-74, MKN-45, SNU-1, SNU-16, and HL-60). The ethyl acetate fraction of PRE and p-coumaric acid significantly inhibited the proliferation of MKN-74 and HL-60 cells, respectively, and induced cell apoptosis, down-regulated Bcl-2 and poly (ADP-ribose) polymerase levels, and up-regulated those of Bax and caspase-3. These results show the potential utility of S. quelpaertensis Nakai leaves in cancer prevention.
Cancer is one of the leading causes of death worldwide. The incidence and mortality rates of cancer have increased steadily due to both inherited and environmental causes, e.g., adoption of a Westernized diet.1 Gastric cancer is the second leading cause of cancer death in both sexes worldwide (approximately 738,000 deaths in 2008; 9.7% of all cancer deaths), and the highest incidence rates are seen in Eastern Asia, Eastern Europe, and South America.2 Surgery and chemotherapy are the main treatments for gastric cancer; however, adverse reactions can occur with both. To reduce side effects, research on anticancer agents in natural substances that have been proven safe has been ongoing.3 In recent years, cancer cell apoptosis has been researched as a potential therapeutic target,4 and natural products are becoming an increasingly important option for inducing apoptosis.5 Apoptosis is a selective process of physiological cell deletion that regulates the balance between cell proliferation and cell death. Failure of apoptosis is considered to contribute to the development of human malignancies.6 Recent studies have shown the benefits of cancer chemotherapeutics and their pharmacological effects in part by triggering apoptotic cell death; as such, the induction of apoptosis in cancer cells has become a target in cancer treatment.7
Sasa quelpaertensis Nakai is an endemic dwarf bamboo which is distributed Mt. Halla of Jeju Island, Republic of Korea. Its leaf extract has been reported to possess several health-promoting properties, including anti-inflammatory, anticancer, and anti-obesity activities.8910 S. quelpaertensis Nakai also protects against gamma radiation-induced damage in mice.11 We previously reported that the phytochemical-rich extract (PRE), which was prepared using a by-product of hot water extract of S. quelpaertensis Nakai leaves, had high anti-oxidant and anti-inflammatory properties.12 PRE is a mixture of polysaccharides, amino acids, and polyphenols, including p-coumaric acid and tricin known for their anti-inflammatory and anti-obesity effects.1314 However, the effects of PRE and p-coumaric on human cancer cells, and the underlying molecular mechanisms of action, are not yet completely understood. In this study, we investigated the effects of the ethyl acetate fraction of PRE (EPRE) and p-coumaric on the proliferation of several human cancer cell types.
We obtained RPMI 1640 medium, fetal bovine serum (FBS), and penicillin–streptomycin (PS) from Gibco (Grand Island, NY, USA). Antibodies to Bcl-2, Bax, procaspase-3, and poly (ADP-ribose) polymerase (PARP) were purchased from Santa Cruz Biotechnology (Santa Cruz, CA, USA). Antibodies to cleavage caspase-3 were purchased from Cell Signaling Technology (Beverly, MA, USA). Phosphate-buffered saline (PBS) (pH 7.4), 3-(4,5-dimethyl thiazol-2yl)-2,5-diphenyltetrazolium bromide (MTT), propidium iodide (PI), RNase A and p-coumaric acid were purchased from Sigma Chemical Co. (St. Louis, MO, USA). p-coumaric acid was dissolved in dimethyl sulfoxide (DMSO) to make a 200-mM solution. All other reagents were purchased from Sigma Chemical Co., unless otherwise stated.
Human gastric cancer cells (MKN-74, MKN-45, SNU-1, SNU-16) and acute leukemia (HL-60) were obtained from the Korean Cell Line Bank (KCLB; Seoul, Korea). The cells were cultured in RPMI-1640 medium (Gibco) supplemented with 10% FBS and antibiotics (penicillin, 100 U/mL; Gibco). Cells cultures were maintained at 37 ℃ in a humidified atmosphere of 5% CO2. Cells in the exponential growth phase were used throughout the experiments.
Cell viability was determined using MTT assay. Briefly, the cells were plated in 96-well plates at 2.0 × 105 ~ 3.0×105 cells/mL, incubated overnight, treated with different concentrations of PRE and p-coumaric acid, and then incubated for an additional 72 hr. Each well was supplemented with 50 µL of MTT and incubated for 4 hr at 37 ℃. The formazan crystals that formed were subsequently dissolved in 150 µL of DMSO, and the optical density of the resultant reaction solution was read at 540 nm using a microplate reader (Bio-Tek, Winooski, VT, USA).
Cells were harvested and fixed with ice-cold 70% ethanol for 30 min.The fixed cells were stained with 5 µL of PI at room temperature in the dark for 30 min. Apoptotic cells were counted using a flow cytometer (FACS Calibur; Becton Dickinson, Franklin Lakes, NJ, USA).
Cells were washed with ice-cold PBS, collected, and centrifuged. The resulting cell pellets were resuspended in lysis buffer [1 × RIPA; 1 mM phenylmethylsulfonyl fluoride, 1 mM Na3VO4, 1 mM NaF, 1 µg/mL aprotinin, 1 µg/mL pepstatin, and 1 µg/mL leupeptin (Upstate Biotechnology, Temecula, CA, USA)] and incubated on ice for 1 hr. Cell debris was then removed by centrifugation, and lysate protein concentrations were determined using Bio-Rad Protein Assay Reagent (Bio-Rad Laboratories, Hercules, CA,USA). Cell lysates were then subjected to electrophoresis on 10 – 15% polyacrylamide gels containing sodium dodecyl sulfate (SDS) and transferred to polyvinylidene difluoride membranes. The membranes were blocked by incubation for 1 hr at room temperature with a 0.1% solution of Tween 20 in Tris-buffered saline containing 5% nonfat dry milk or 5% bovine serum albumin (BSA). After incubation overnight at 4 ℃ with primary antibody, the membranes were incubated for 1 hr at room temperature with horseradish peroxidase-conjugated secondary antibody. Immunodetection was carried out using enhanced chemiluminescence (ECL) Western blotting detection reagent (Cyanagen, Bologna, Italy).
All qualitative data are representative of at least three independent experiments. Values are expressed as means ± standard deviation (SD). Oneway analysis of variance (ANOVA) was used for multiple comparisons. Treatment effects were analyzed using Duncan's multiple range test, performed with SPSS software (ver. 12.0; SPSS Inc., Chicago, IL, USA). Differences were considered statistically significant at p < 0.05.
In this study, we evaluated the cell growth inhibition activity of PRE fractions and p-coumaric acid using four human gastric cancer cell lines (MKN-74, MKN-45, SNU-16, and SNU-1 cell) and acute leukemia cell line (HL-60 cell). Hexane, chloroform, ethylacetate and butanol fractions of PRE inhibited the proliferation of these cells to differing extents (Fig. 1). Among the fractions, chloroform fractions of PRE (CPRE) and ethylacetate fractions of PRE (EPRE) showed a significant inhibitory effect on the proliferation of MKN-74 cells. Because CPRE showed cytotoxic effects on a normal cell line (Hs68 cells; data not shown), we used EPRE in subsequent experiment. As shown in Fig. 1A, EPRE (100 µg/mL) exhibited the anti-proliferative effects against various cancer cell lines in the order of MKN-74 cells (81%) > SNU-16 cells (80%) > HL-60 cells (60%) > MKN-45 cells (51%) > SNU-1 cells (30%).
p-Coumaric acid is the most abundant phytochemical (44.10 mg/g) in PRE,12 thus the bioavailability of p-coumaric acid may be relatively higher comparing other constituents within body fluid. Recent study have suggested that a 1,500 µM concentration of p-coumaric acid is within a physiological range in the colon after consumption of plant extracts.15 On the basis of this report, we used 2,000 µM of p-coumaric acid as the highest concentration in the present study. As shown in Fig. 1.B, p-coumaric acid (2,000 µM) effectively inhibited the growth of HL-60 cells without cytotoxicity. Also, it inhibited the proliferation of gastric cancer cell lines in the order of HL-60 cells (88%) >SNU-16 cells (48%) > SNU-1 cells (40%) > MKN-74 cells (25%) > MKN-45 cells (21%) (Fig. 1.B).
It has been reported that the extract of S. quelpaertensis Nakai leaves and p-coumaric acid induced apoptosis in human colon cancer cells, as well as in human leukemia cells.169 Consistent with previous studies, our results demonstrated that EPRE and p-coumaric acid effectively inhibited the growth of human gastric cancer cells (MKN-74 cells) and human leukemia cells (HL-60 cells), respectively. p-Coumaric acid showed anti-cancer activity at relatively higher concentration comparing to EPRE, suggesting that p-coumaric acid is not a major active compound. Therefore, it is suggested that potent anticancer activity of EPRE is exerted through the additive and synergistic actions of various phenolic compounds, such as p-coumaric acid, chlorogenic acid, orientin, vitexin, and tricin.17
Induction of apoptosis by EPRE and p-coumaric acid was evaluated using flow cytometry by measuring the amount of sub-G1 DNA. EPRE and p-coumaric acid induced apoptosis in HL-60 cells (Fig. 2). In the control group, the sub-G1 DNA content was 4.37%, compared with 52.36% with a concentration of 200 µg/mL EPRE and 54.37% with 2,000 µM of p-coumaric acid, revealing that G1 arrest, which is an indicator of apoptosis, increased with increasing concentrations of EPRE and p-coumaric acid. Therefore, it was confirmed that the cell growth inhibitory activity by EPRE and p-coumaric acid was due to the apoptosis rather than by cell necrosis.
Apoptosis can be activated through two major pathways: the mitochondria-dependent pathway and the death receptor-dependent pathway. In the mitochondria-dependent signaling pathway, the Bcl-2 family plays an important role in pro- and anti-apoptosis signaling. The Bcl-2 family includes both pro-apoptotic (Bax, Bad, and Bak) and anti-apoptotic members (Bcl-2).18 Bcl-2 is among the best-known apoptosis-suppressing gene products,19 and Bax is a prototype apoptosis-promoting gene product.20 Regulation of the expression of Bcl-2 family members on the mitochondria is followed by activation of caspase-3 and caspase-9, and cleavage of PARP.21 Caspase-3 is one of the key executioners of apoptosis, being cleaved by numerous proteins including nuclear enzyme PARP.22
To elucidate the molecular mechanism underlying apoptosis by EPRE and p-coumaric acid, we assessed the expression of Bax, Bcl-2, procapspase-3, and PARP in MKN-74 cells and HL-60 cells. As shown in Figure 3, EPRE and p-coumaric acid significantly decreased the expression of Bcl-2, procaspase-3 and PARP and increased the expression of Bax in a dose-dependent manner. These data suggest that the apoptosis induced by EPRE and p-coumaric acid also involves caspase-3-mediated mechanisms.
In summary, we demonstrated that EPRE and p-coumaric acid effectively inhibit the proliferation of MKN-74 cells and HL-60 cells by inducing apoptosis. The relative expression levels of pro- and anti-apoptotic proteins are key factors in the inhibitory effects of EPRE and p-coumaric acid. Further studies on the anticancer properties of EPRE and p-coumaric acid could further reveal the potential of S. quelpaertensis leaves in cancer prevention.
Figures and Tables
Fig. 1
Growth-inhibiting effects of phytochemical-rich extract (PRE) fraction (A) and p-coumaric acid (B) on the several human cancer cell lines. Data are means ± standard deviation (SD) of three independent experiments. ***p < 0.001, **p < 0.01, and *p < 0.05 compared to the untreated group.
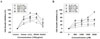
Fig. 2
Apoptosis inducing effects of ethyl acetate fraction of PRE (EPRE) (A) and p-coumaric acid (B) in HL-60 cells. Data (means ± SD of triplicate determinations) are representative of at least three independent experiments. ***p < 0.001 compared to the untreated group. (A) PRE, (B) p-coumaric acid.
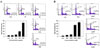
Acknowledgment
This research was supported by Business for Cooperative R&D between Industry, Academy, and Research Institute funded Korea Small and Medium Business Administration in 2016 (grant number C0394780).
References
1. Doll R. Cancer Res. 1992; 52:2024S–2029S.
2. Jemal A, Bray F, Center MM, Ferlay J, Ward E, Forman D. CA Cancer J Clin. 2011; 61:69–90.
3. Kim EJ, Part H, Lim SS, Kim JS, Shin HK, Yoon JH. Korean J Food Sci Technol. 2008; 40:207–214.
4. Fulda S, Debatin KM. Curr Cancer Drug Targets. 2004; 4:569–576.
5. Ji YB, Qu ZY, Zou X. Exp Toxicol Pathol. 2011; 63:69–78.
6. Shinkai K, Akedo H, Mukai M, Imamura F, Isoai A, Kobayashi M, Kitagawa I. Jpn J Cancer Res. 1996; 87:357–362.
7. Ahmad N, Feyes DK, Nieminen AL, Agarwal R, Mukhtar H. J Natl Cancer Inst. 1997; 89:1881–1886.
8. Hwang JH, Choi SY, Ko HC, Jang MG, Jin YJ, Kang SI, Park JG, Chung WS, Kim SJ. Food Sci Biotechnol. 2007; 16:728–733.
9. Jang MG, Park SY, Lee SR, Choi SY, Hwang JH, Ko HC, Park JG, Chung WS, Kim SJ. Food Sci Biotechnol. 2008; 17:188–190.
10. Kim M, Kim YS, Kim KM, Ko HC, Kim SJ, Kim JH, Kim Y. Integr Cancer Ther. 2014; 13:529–540.
11. Kim A, Bing SJ, Cho J, Herath K, Ahn G, Park E, Kim MJ, Kim DS, Kim JS, Lee NH, Jee Y. J Prev Vet Med. 2015; 39:169–174.
12. Lee JY, Ko HC, Jang MG, Kim SJ. J Life Sci. 2016; 26:1330–1335.
13. Kang SW, Kang SI, Shin HS, Yoon SA, Kim JH, Ko HC, Kim SJ. Food Chem Toxicol. 2013; 59:380–385.
14. Kim A, Bing SJ, Cho J, Herath K, Ahn G, Park E, Kim MJ, Kim DS, Kim JS, Lee NH, Jee Y. J Prev Vet Med. 2016; 39:169–174.
15. Janicke B, Onning G, Oredsson SM. J Agric Food Chem. 2005; 53:6658–6665.
16. Jaganathan SK, Supriyanto E, Mandal M. World J Gastroenterol. 2013; 19:7726–7734.
17. Liu MH, Ko CH, Ma N, Tan PW, Fu WM, He JY. J Funct Foods. 2016; 22:533–546.
18. Hunt A, Evan G. Science. 2001; 293:1784–1785.
19. Burlacu A. J Cell Mol Med. 2003; 7:249–257.
20. Kuwana T, Mackey MR, Perkins G, Ellisman MH, Latterich M, Schneiter R, Green DR, Newmeyer DD. Cell. 2002; 111:331–342.
21. Green DR, Reed JC. Science. 1998; 281:1309–1312.
22. Fernandes-Alnemri T, Litwack G, Alnemri ES. J Biol Chem. 1994; 269:30761–30764.