Abstract
Halophytes are expected to possess abundant secondary metabolites and various biological activities because of habitat in extreme environments. In this study, we collected 14 halophytes (Asparagus oligoclonos, Calystegia soldanella, Carex pumila, Chenopodium glaucum, Elymus mollis, Glehnia littoralis, Limonium tetragonum, Messerschmidia sibirica, Rosa rugosa, Salsola komarovii, Spergularia marina, Suaeda glauca, Suaeda maritima, and Vitex rotundifolia) native to Korea and compared their total polyphenol contents, antioxidant and anti-inflammatory activities. The total polyphenol contents of R. rugosa (27.28%) and L. tetragonum (13.17%) were significantly higher than those of the other 12 halophytes and L. tetragonum, R. rugosa, and M. sibirica showed significantly greater antioxidant activities than the other 11 halophytes, as determined by DPPH (2,2-diphenyl-1-picrylhydrazyl). A. oligoclonos, E. mollis, and C. pumila showed significantly greater anti-inflammatory activities than the other 11, as determined by NO (Nitric oxide) and PGE2 (Prostaglandin E2) levels. In contrast, these three extracts had normal and low total polyphenol contents among the 14 halophytes. Consequently, the total polyphenol content in the 14 studied halophytes appeared to be related to antioxidant, but not anti-inflammatory activity levels.
Halophyte plants that grow in saline environments (tidal flats, sand dunes, and coast) have unique adaptive mechanisms that allow them to withstand high salt concentrations and thus have a unique physiologically active substance.1 For this reason, halophyte plants have the potential to be used as edible plants, fuel, medicine, and a source of useful chemicals.2 Recently, studies on halophyte plants have been conducted to identify unique metabolites to determine whether they correlate to bioactivities of interest to industry and medicine.3
Deleterious environmental conditions such as salinity, drought, and luminosity lead to oxidative stress in halophyte plants and to the production of reactive oxygen species (ROS: the superoxide anion, hydroxyl radical, and hydrogen peroxide) and reactive nitrogen species (RNS: nitric oxide, nitrogen dioxide, and peroxynitrite). ROS and RNS are involved in processes that cause cellular damage, metabolic disorders, senescence, aging, cancer, arthritis, arteriosclerosis, and dermatitis.4567 Halophyte plants also produce various secondary metabolites, including polyphenol, carotenoids, and vitamins to withstand the oxidative stress generated by severe environmental conditions.8 Indeed, halophyte plants are known to possess various biological activities such as neuroprotective, antioxidant, antibacterial, anti-inflammatory, anticancer, and hepatoprotective effects.3910111213
Polyphenol compounds, which are ubiquitous in nature, are characterized by an aromatic ring bearing one or more hydroxyl groups. These compounds exert beneficial health and physiological properties including antioxidant, antimicrobial, anti-inflammatory, analgesic, antipyretic, cardioprotective, antihypertensive, anticancer, anti-allergic, anti-wrinkle, and antidiabetic effects.141516171819202122 For these reasons, interest in polyphenol compounds as health supplements is increasing. In this study, we evaluated the association between polyphenol content and anti-inflammatory and anti-oxidant activities in 14 halophyte plants (Asparagus oligoclonos, Calystegia soldanella, Carex pumila, Chenopodium glaucum, Elymus mollis, Glehnia littoralis, Limonium tetragonum, Messerschmidia sibirica, Rosa rugosa, Salsola komarovii, Spergularia marina, Suaeda glauca, Suaeda maritima, and Vitex rotundifolia) found in Korea.
Halophyte plant samples [A. oligoclonos (H1), C. soldanella (H2), C. pumila (H3), C. glaucum (H4), E. mollis (H5), G. littoralis (H6), L. tetragonum (H7), M. sibirica (H8), R. rugosa (H9), S. komarovii (H10), S. marina (H11), S. glauca (H12), S. maritima (H13), and V. rotundifolia (H14)] were collected from Taean-gun, Chungcheongnam-do, Korea in June 2016. The collected halophyte plants were identified by Ph. D. J. H. Lee, Korea Medicinal Resources Herbarium (KMRH). Voucher specimens were deposited at the Marine Biodiversity Institute of Korea (MABIK). Each whole plant was lyophilized separately and extracted five times with 70% EtOH for 1 h using a sonicator (WUC-N30H, DAIHAN Scientific Co. Ltd., Korea). The resultant extracts were concentrated using a Buchi rotary evaporator R-120 system (Buchi, Switzerland) under reflux in vacuo, lyophilized, pulverized, and stored at −80 ℃ until use.
The absorbance was measured using a microplate reader (EL800, Bio-Tek, USA). RPMI (Roswell Park Memorial Institute) 1640, FBS (Fetal Bovine Serum), DMEM (Dulbecco Modified Eagle Medium), penicillin, and streptomycin were purchased from Gibco/BRL Life Technologies Inc. (USA), and CCKs (Cell Counting Kit-8) were purchased from Dojindo Laboratories (Japan). Gallic acid, Folin-Ciocalte reagent, Na2CO3 (Sodium carbonate), DPPH (2,2-diphenyl-1-picrylhydrazyl), potassium persulfate, LPS (lipopolysaccharide), and Griess reagent were obtained from Sigma (USA). All other reagents were of analytical grade.
The total polyphenol contents of the halophyte extracts and gallic acid (10~500 µg/mL) were determined using Folin–Ciocalteu reagent.23 Briefly, 20 µL of each extract was added to 100 µL Folin-Ciocalteu reagent and then allowed to react in the dark for 3 min at 25℃. This mixture was added to 80 µL 7.5% Na2CO3 and placed in the dark for 20 min at 25 ℃. The absorbance was then determined in triplicate at 765 nm using a microplate reader. The total polyphenol content was calculated with a calibration curve created with a gallic acid standard and expressed as a percentage (%) of gallic acid equivalents per gram of extract (mg/GAEg).
DPPH assays of the halophyte extracts were performed according to the method described by Lee et al..23 Briefly, 100 µL of various concentrations (1.563~400 µg/mL) of the halophyte extract was added to 100 µL of 60 µM DPPH in a 96-well plate. The mixture was shaken vigorously and left in the dark at 25 ℃ for 30 min, after which absorbance was measured at 516 nm with ascorbic acid as a positive control. DPPH scavenging activity was expressed as the half maximal inhibitory concentration (IC50) value.
RAW 264.7 macrophages cells were obtained from the American Type Culture Collection (USA). The cells were cultured in RPMI 1640 and supplemented with 10% FBS, penicillin (100 U/mL), and streptomycin (100 µg/mL) at 37 ℃ in a 5% CO2 incubator. Cells were washed with DMEM and treated in serum-free medium for at least 4 h prior to treatment. The cells were then stimulated with LPS.
The cell growth inhibition was assessed using a CCK assay described by Cho et al..24 The cells were seeded at a density of 3 × 105 cell/mL into 96-well plates and then the 96-well plate was treated with the indicated difference concentrations (10~500 µg/mL) of each halophyte extract. After incubation for 24 h, CCK-8 was added and incubated at 37 ℃ for 2 h, the 96-well plates were read at 450 nm with a microplate reader.
NO generated by the cells was measured using the Griess reaction method.23 Briefly, RAW 264.7 cells were plated in 96-well plates at a density of 1.5 × 105 cell/mL and then treated with the indicated different concentrations (10~500 µg/mL) of each halophyte extract. The cells were stimulated with 1 µg/mL LPS for 24 h after which 100 µL cell culture medium was added to 100 µL Griess reagent and the mixture was incubated at 25 ℃ for 10 min. The absorbance at 540 nm was measured using a microplate reader. The NO production was expressed as a percentage (%).
PGE2 production of the halophyte extracts was measured according to the method described by Lee et al..23 Briefly, RAW 264.7 cells were plated in 24-well plates and treated with the indicated concentrations (10~500 µg/mL) of each extract. The cells were then stimulated with 1 µg/mL LPS for 24 h. PGE2 expression levels were measured using an enzyme immunosorbent assay (ELISA) kit (Cayman chemical, USA). The amount of PGE2 released was expressed as a percentage (%).
The total polyphenol content of the 14 halophyte extracts ranged between 1.50% and 27.28% (Table 1). The highest total polyphenol content was found in the R. rugosa extract (27.28%, H9), followed by the L. tetragonum extract (13.17%, H7). The total polyphenol contents in the other 12 halophytes decreased as follows: C. pumila (7.53%, H3), S. komarovii (5.93%, H10), C. soldanella (5.01%, H2), M. sibirica (5.01%, H8), V. rotundifolia (4.43%, H14), C. glaucum (3.32%, H4), G. littoralis (3.28%, H6), A. oligoclonos (3.01%, H1), S. glauca (2.99%, H12), S. marina (1.86%, H11), S. maritima (1.59%, H13), and E. mollis (1.50%, H5). Of note is that the phenol content of R. rugosa (H9) was twice as high as that of L. tetragonum (H7) and approximately twenty times higher than that of E. mollis (H5).
Antioxidant activity of halophytes was determined using DPPH assay (Fig. 1), and the DPPH radical scavenging activities were expressed as IC50 values. The highest antioxidant activities were found in L. tetragonum (17.28 µg/mL, H7), R. rugosa (29.73 µg/mL, H9), and M. sibirica (60.33 µg/mL, H8). V. rotundifolia (85.11 µg/mL, H14), G. littoralis (89.82 µg/mL, H6), C. soldanella (112.13 µg/mL, H2), and C. pumila (235.80 µg/mL, H3) exhibited moderate antioxidant activity. C. glaucum (409.75 µg/mL, H4), A. oligoclonos (421.25 µg/mL, H1), E. mollis (H5), S. komarovii (H10), S. glauca (H12), S. marina (H11), and S. maritima (H13) showed weak or nonexistent antioxidant activities.
We examined cell viability after treatment with the 14 halophyte extracts via a CCK-8 assay (Fig. 2). No significant cytotoxicity was observed, although treatment with C. glaucum (H4) reduced cell viability to 60% at a dose of 50 µg/mL and 67% at a dose of 100 µg/mL.
Anti-inflammatory activity of the halophyte extracts was evaluated by measuring NO production and PGE2 levels in LPS-stimulated RAW264.7 cells after treatment with the extracts. As shown in Fig. 3., all the halophyte extracts suppressed NO production. A. oligoclonos (500 µg/mL, H1) and E. mollis (500 µg/mL, H5) inhibited NO production by 11.83 and 30.41%, respectively. In particular, C. pumila (100 µg/mL, H3) and G. littoralis (100 µg/mL, H6) inhibited NO production by 44.22 and 26.39%, respectively.
As shown in Fig. 4., A. oligoclonos (H1) and E. mollis (H5) at 300 µg/mL inhibited PGE2 release by 44.10 and 33.72%, respectively, whereas at 500 µg/mL they inhibited PGE2 release by 30.93 and 11.49%, respectively. C. pumila (H3) caused the strongest inhibition of PGE2 release at 30, 50, and 100 µg/mL of 53.94, 36.6 and 8.74%, respectively.
A. oligoclonos (H1), E. mollis (H5), and C. pumila (H3) showed strong anti-inflammatory activities at concentrations that did not affect cell viability.
Polyphenol compounds found in halophytes that possess antioxidant, antimicrobial, and anticancer properties have recently gained interest as important biological source materials.1225 In this study, we investigated the relationship between total polyphenol content and antioxidant and anti-inflammatory activities in 14 halophytes from Korea.
Three halophyte extracts (L. tetragonum (H7), M. sibirica (H8) and R. rugosa (H9)) showed strong antioxidant activities, while L. tetragonum (H7) and R. rugosa (H9) also showed high total polyphenol contents. In previous studies, four flavonoids that had been isolated from L. tetragonum (H7) were investigated by measuring DPPH, peroxynitrite scavenging, intracellular ROS, lipid peroxidation, and DNA oxidation levels in HT-1080 cells and showed strong antioxidant activities.2627 In another study, the polyphenolic contents of R. rugosa (H9) was found to be 6.9~19.8 mg catechin equivalents (CE)/g fresh weight (FW) and was investigated by measuring DPPH, superoxide, and hydroxyl free radical levels.28 A gallic acid derivative of R. rugosa (H9) was also found to exhibit antioxidative activity.29 These results demonstrate a close relationship between polyphenolic concentration and antioxidant activity of R. rugosa (H9) and L. tetragonum (H7). Accordingly, polyphenols of L. tetragonum (H7) and R. rugosa (H9) are expected to be developed as natural antioxidants. On the other hand, despite a lower polyphenol content than R. rugosa (H9) and L. tetragonum (H7), M. sibirica (H8) possessed a strong antioxidant activity. There has been little research on M. sibirica (H8) and its biological activities. Accordingly, chemical studies on M. sibirica (H8) related with antioxidant activity are required.
We found that three halophyte extracts (A. oligoclonos (H1), E. mollis (H5), and C. pumila (H3)) possessed strong anti-inflammatory activities along with normal or low total polyphenol content. In previous studies, total polyphenol content of A. oligoclonos (H1) was not determined and the anti-inflammatory effect of A. oligoclonos (H1) extract was preliminarily screened by measuring nitrite, TNF-α, and IL-6 levels. A steroidal saponins isolated from A. oligoclonos (H1) has been reported that possess anti-inflammatory activity. Therefore, we posit that the anti-inflammatory effect shown in our results is due to the effect of steroidal saponins.303132 The research on the anti-inflammatory properties of C. pumila (H3) has been not conducted. In previous studies, a stilbene was isolated from C. pumila (H3), and these stilbenes have been reported to possess anti-inflammatory activity, and the anti-inflammatory effect shown in our results may be caused by stilbene.33343536 No studies as of yet have examined the biological properties and secondary metabolites of E. mollis (H5). Future studies are needed to determine if E. mollis (H5) possesses significant antiinflammatory properties.
In conclusion, we evaluated the association between polyphenol content and anti-inflammatory and antioxidant activities in 14 halophyte plants found in Korea. The polyphenols found in L. tetragonum (H7) and R. rugosa (H9), but not M. sibirica (H8), were found to be associated with antioxidant activity. However, the polyphenols found in A. oligoclonos (H1), E. mollis (H5), and C. pumila (H3) extract were not associated with their anti-inflammatory activity. Therefore, additional studies are required to isolate, identify, and measure the antioxidant and anti-inflammatory activities of secondary metabolites found in M. sibirica (H8), A. oligoclonos (H1), E. mollis (H5), and C. pumila (H3).
Figures and Tables
Fig. 1
DPPH radical scavenging activity of 14 halophyte extracts expressed as half maximal inhibitory concentration (IC50). All the data are shown as mean ± SD from at least three independent experiments performed in triplicate.
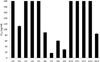
Fig. 2
Cell viability was evaluated using a CCK-8 assay after treatment with various concentrations of halophytes (10~500 µg/mL). The data are represented as % of the untreated control. All the data are shown as mean ± SD from at least three independent experiments performed in triplicate.
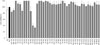
Fig. 3
Inhibition of NO production after the treatment of lipopolysaccharide-stimulated RAW 264.7 cells with extracts from 14 halophytes. The experiment was performed in triplicate and data are represented as mean ± SD. *p<0.05; **p<0.01; ***p<0.001.
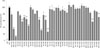
Acknowledgments
This study was supported by the National Marine Biodiversity Institute of Korea Research Program (2018M00700). The authors thank Ph. D. Jeong Hoon Lee, Department of Herbal Crop Research, National Institute of Horticultural and Herbal Science (NIHHS), Korea, for the identification of 14 halophytes.
References
1. Liebezeit G, Künnemann TD, Gad G. J Biotech. 1999; 70:77–84.
2. Abdelly C, Barhoumi Z, Ghnaya T, Debez A, Ben Hamed K, Ksouri R, Talbi O, Zribi F, Ouerghi Z, Smaoui A, Huchzernmeyer B, Grignon C. Potential utilization of halophytes for the rehabilitation and valorization of salt-affected areas in Tunisia. In : Öztürk M, Waisel Y, Khan MA, Görk G, editors. Biosaline Agriculture and Salinity Tolerance in Plants. New York: Springer;2006. p. 161–170.
3. Ksouri R, Ksouri WM, Jallali I, Debez A, Magné C, Hiroko I, Abdelly C. Crit Rev Biotechnol. 2012; 32:289–326.
4. Virág L, Szabó E, Gergely P, Szabó C. Toxicol Lett. 2003; 140-141:113–124.
5. Wang B, Lüttge U, Ratajczak R. J Plant Physiol. 2004; 161:285–293.
6. Drel VR, Pacher P, Vareniuk I, Pavlov I, Ilnytska O, Lyzogubov VV, Tibrewala J, Groves JT, Obrosova IG. Eur J Pharmacol. 2007; 569:48–58.
7. Korda M, Kubant R, Patton S, Malinski T. Am J Physiol Heart Circ Physiol. 2008; 295:H1514–H1521.
8. Jaleel CA, Gopi R, Manivannan P, Panneerselvam R. Turk J Bot. 2007; 31:245–251.
9. Manikandan T, Neelakandan T, Usha Rani G. J Phytol. 2009; 1:441–443.
10. Falleh H, Ksouri R, Medini F, Guyot S, Abdelly C, Magné C. Ind Crops Prod. 2011; 34:1066–1071.
11. Gnanadesigan M, Ravikumar S, Inbaneson SJ. Asian Pac J Trop Med. 2011; 4:462–465.
12. Oueslati S, Ksouri R, Falleh H, Pichette A, Abdelly C, Legault J. Food Chem. 2012; 132:943–947.
13. Kim MS, Seo JY, Oh J, Jang YK, Lee CH, Kim JS. J Med Food. 2017; 20:140–151.
14. Kojima T, Akiyama H, Sasai M, Taniuchi S, Goda Y, Toyoda M, Kobayashi Y. Allergol Int. 2000; 49:69–73.
15. An BJ, Kwak JH, Park JM, Lee JY, Park TS, Lee JT, Son JH, Jo C, Byun MW. Dermatol Surg. 2005; 31:848–854.
16. dos Santos MD, Almeida MC, Lopes NP, de Souza GE. Biol Pharm Bull. 2006; 29:2236–2240.
17. Moreno S, Scheyer T, Romano CS, Vojnov AA. Free Radic Res. 2006; 40:223–231.
18. Mohan KV, Gunasekaran P, Varalakshmi E, Hara Y, Nagini S. Cell Biol Int. 2007; 31:599–608.
19. Mokni M, Limam F, Elkahoui S, Amri M, Aouani E. Arch Biochem Biophys. 2007; 457:1–6.
20. Yamabe N, Kang KS, Matsuo Y, Tanaka T, Yokozawa T. Biol Pharm Bull. 2007; 30:1289–1296.
21. Cienfuegos-Jovellanos E, Quiñones Mdel M, Muguerza B, Moulay L, Miguel M, Aleixandre A. J Agric Food Chem. 2009; 57:6156–6162.
22. Perron NR, Brumaghim JL. Cell Biochem Biophys. 2009; 53:75–100.
23. Lee JM, Yim MJ, Choi G, Lee MS, Park YG, Lee DS. Nat Prod Sci. 2018; 24:40–46.
24. Cho MS, Park WS, Jung WK, Qian ZJ, Lee DS, Choi JS, Lee DY, Park SG, Seo SK, Kim HJ, Won JY, Yu BC, Choi IW. Pharm Biol. 2014; 52:926–932.
25. Ksouri R, Falleh H, Megdiche W, Trabelsi N, Mhamdi B, Chaieb K, Bakrouf A, Magné C, Abdelly C. Food Chem Toxicol. 2009; 47:2083–2091.
26. Lee JI, Kong CS, Jung ME, Hong JW, Lim SY, Seo Y. Biotechnol Bioprocess Eng. 2011a; 16:992–999.
27. Lee JI, Kong CS, Jung ME, Hong JW, Noh I, Seo Y. Ocean Polar Res. 2011b; 33:185–191.
28. Youwei Z, Yonghong P. N Z J Crop Hortic Sci. 2007; 35:397–401.
29. Ng TB, He JS, Niu SM, Zhao L, Pi ZF, Shao W, Liu F. J Pharm Pharmacol. 2004; 56:537–545.
30. Kim GS, Kim HT, Seong JD, Oh SR, Bang JK, Seong NS, Song KS. J Nat Prod. 2005; 68:766–768.
31. Adão CR, de Silva BP, Parente JP. Fitoterapia. 2011; 82:1175–1180.
32. Balica G, Vostinaru O, Tamas M, Crisan G, Mogosan C. J Food Agric Environ. 2013; 11:106–108.
33. Kurihara H, Kawabata J, Ichikawa S, Mizutani J. Agric Biol Chem. 1990; 54:1097–1099.
34. Kawabata J, Mishima M, Kurihara H, Mizutani J. Phytochemistry. 1991; 30:645–647.
35. Kawabata J, Mishima M, Kurihara H, Mizutani J. Phytochemistry. 1995; 40:1507–1510.
36. Chung EY, Kim BH, Lee MK, Yun YP, Lee SH, Min KR, Kim Y. Planta Med. 2003; 69:710–714.