Abstract
Optimal performance of pediatric cardiothoracic computed tomography (CT) is technically challenging and may need different approaches for different types of CT scanners. To meet the technical demands and improve clinical standards, a practical, user-friendly, and vendor-specific guideline for pediatric cardiothoracic CT needs to be developed for children with congenital heart disease (CHD). In this article, we have attempted to describe such guideline based on the consensus of experts in the Asian Society of Cardiovascular Imaging CHD Study Group. This first part describes the imaging techniques of pediatric cardiothoracic CT, and it includes recommendations for patient preparation, scan techniques, radiation dose, intravenous injection protocol, post-processing, and vendor-specific protocols.
In congenital heart disease (CHD), the role of cardiothoracic computed tomography (CT) using state-of the-art imaging techniques is evolving rapidly in clinical practice (123). The expanded role of cardiothoracic CT is attributed to the recent technical developments in CT imaging, which are temporal resolution, scan speed, longitudinal coverage, and electrocardiography (ECG)-synchronized data acquisition (4567). It is quite challenging to obtain quality cardiothoracic CT images in children with CHD. This is chiefly because of a wide spectrum of morphologic abnormalities and the resultant hemodynamic complexities that are difficult to predict. Therefore, cardiothoracic CT protocols should be highly individualized to acquire optimal images in these patients. In this regard, a guideline for pediatric cardiothoracic CT imagers would be very helpful to achieve optimized standards. Currently, pertinent specifications and parameters, such as the number of X-ray tube, temporal resolution, longitudinal coverage, and detector configuration, vary among different CT models, and these factors should be considered during the development of a truly practical CT guideline for users. However, the current technical diversity among different CT models at present is not fully incorporated into the existing guidelines (89). Moreover, CT is generally preferred to magnetic resonance imaging (MRI) for evaluating children with CHD in Asian countries; this is mainly due to high costs of MRI, lack of availability, the limitations in human resources, such as dedicated physicists and pediatric anesthesiologist for pediatric cardiac MRI, and a heavy workload of radiologists (10). However, a pediatric cardiothoracic CT guideline considering such regional differences in clinical practice in Asia is currently lacking. Therefore, we developed not only a comprehensive but also vendor-specific guideline for pediatric cardiothoracic CT on the imaging techniques based on as the consensus opinions of the experts in the Asian Society of Cardiovascular Imaging; the guideline includes recommendations for patient preparation, scan techniques, radiation dose reduction techniques, intravenous iodinated contrast agent injection protocols, and post-processing techniques. Vendor-neutral and vendor-specific check points are summarized in Tables 1 and 2, respectively.
Careful patient preparation is a prerequisite for successful performance of pediatric cardiothoracic CT. Detailed pre-procedural patient assessment including age, body size indices, developmental stage, cooperativity, vital signs, oxygen saturation, renal function, and venous accessibility, should be performed by certified medical personnel. Maintenance of body temperature during the examination is also crucial in newborns to support their immature thermoregulation. Patient positioning at the isocenter of the CT gantry is important to avoid image quality degradation and inappropriate high or low radiation exposure, especially when CT scanning is combined with attenuation-based tube current modulation and use of an additional bowtie filter (411). In this respect, radiology technicians should ensure a patient is isocentered by utilizing the laser beams on the scanner. Because a small child is commonly positioned below the isocenter even at the highest CT table position (12), an additional patient pad or a vacuum device may be necessary to lift the child further up to the isocenter; on this occasion, it is desirable to be radiolucent in order to avoid artifacts (13). Radiopaque ECG leads for ECG-synchronized CT should be placed outside the scan range (e.g., on the arms and upper abdomen) to avoid artifacts (1214). Of note, radiolucent ECG lead sets made of carbon may be used to reduce the artifacts from radiopaque ECG leads (1115). It is better to secure both arms above the head because the position is ideal for the optimization of image quality and radiation dose (1316). Because horizontal position tends to cause severe artifacts (13), the arm position in a sleeping child should be meticulously changed to other positions when possible. Patient immobilization is important not only to reduce motion artifacts but also to reduce the depth of sedation in young children. A vacuum device is helpful for this purpose (11); however, if such a device is not available, a blanket with bands may be used as an alternative.
A pediatric radiologist, a cardiologist, or an anesthesiologist may be involved in patient sedation for pediatric cardiothoracic CT based on the policies at each institution. Special caution should be exercised in sedating children with CHD, because they often have the American Society of Anesthesiologists physical status of class III or greater, which is associated with higher occurrence of sedation-related adverse events (17). Levels of sedation are categorized into minimal, moderate, deep sedation, and general anesthesia (17). In contrast to cardiac MRI, which requires a long examination time in the range of 30 minutes to one hour, cardiothoracic CT requires a short examination time less than several minutes; therefore, a lighter level of sedation is generally sufficient for the latter. In addition to the well-known risk of cardiovascular and respiratory complications, long-term neurodevelopmental impairment has recently been recognized to be a potential complication of deeper, repeated, or prolonged sedation in young children (18). It should be emphasized that sufficient levels of sedation necessary for quality pediatric cardiothoracic CT can almost always be achieved without using general anesthesia and endotracheal intubation. Consequently, the minimal level of sedation required for pediatric cardiothoracic CT should be appropriately and individually adjusted, depending on the patient's condition as well as the utilized CT protocol, to avoid these adverse events. In this respect, imagers determining the CT protocol should be actively involved in this important decision-making process to ensure patient safety. Instead of using sedative medications, a feed-and-swaddle technique utilizing oral 25% dextrose solution and a pacifier may be used in neonates and young infants (89); however, this technique is seldom used in Asia. Despite the above general statements, sedation strategies for pediatric cardiothoracic CT often depend on the institutional policies and preferences. After completion of the CT examination, full monitoring should be maintained in a recovery area until the child is awake.
Beta-blockers and nitroglycerin are commonly administered to reduce the heart rate and to dilate coronary arteries, respectively, during coronary CT angiography in adults; both measures contribute in improving image quality (1920). In adolescents whose coronary arteries are the main diagnostic concern, the same medications may be utilized to increase the diagnostic accuracy of CT (2122). On the contrary, coronary CT angiography in young children is usually performed without these medications, and sufficient image quality can still be achieved (4615212324252627). On the other hand, Rigsby et al. (28) reported the initial experience of using beta-blocker and nitroglycerin in 41 children (mean age 6.5 years; range, 2 days–18 years) who had undergone single-source 32-detector-row ECG-gated coronary CT angiography under general anesthesia with intubation and apnea. Despite the use of bi-segment reconstruction to increase the temporal resolution and the heart rate drop of 5–10 beats per minute (bpm) due to apnea and injection of a contrast agent, the target heart rate was achieved in merely 54% of study participants. Although there were no major complications, hypotension occurred in 44% of the patients, bradycardia in 5%, and respiratory distress in 2%. In addition, two intravenous lines were required for administration of medications and a contrast agent (one line for each), and the total examination time, which was approximately 30 minutes, was substantially prolonged in spite of the intravenous administration of an ultra-short-acting beta-locker (esmolol). Patients should be prudently screened for contraindications to each drug. For beta-blockers, the contraindications are severe asthma, allergy, severe aortic stenosis, severe ventricular systolic dysfunction, atrioventricular block, and use of a calcium channel blocker; for nitroglycerin, the contraindications are hypersensitivity, obstructive hypertrophic cardiomyopathy, severe aortic stenosis, systolic hypotension, constrictive pericarditis, and acute right ventricular infarction. During the CT examination while using these mediations, blood pressure and heart rate should be carefully monitored for early detection of adverse events. Considering the procedure-related complexity and the uncertain benefits in improving image quality, the use of beta-blocker and nitroglycerin for pediatric cardiothoracic CT is not highly recommended. It should be kept in mind that these medications should not be used when CT is used to assess the ventricular function and the hemodynamics in cardiovascular structures.
A variety of scan techniques including non-ECG-synchronized spiral or helical scan, retrospectively ECG-gated spiral or helical scan, prospectively ECG-triggered sequential scan, myocardial delayed enhancement scan, four-dimensional (4D) scan, and dual-energy scan may be used for evaluating children with CHD (Table 1). These scan techniques are generally available in a modern CT scanner. Pediatric cardiothoracic CT should be performed by or under the supervision of an experienced radiologist. Performance of a pediatric cardiac CT examination by a CT technician alone is not recommended because of the complexity of the pathophysiological changes in CHD as well as the diagnostic quality concerns.
Non-ECG-synchronized spiral or helical scan has been commonly utilized for pediatric cardiothoracic CT since the introduction of the slip-ring technology-which enables the use of continuously rotating scan-in 1987. This scan mode chiefly allows the assessment of the extracardiac thoracic vessels, the lungs, and the airways that is still clinically relevant (2930). Hardware advancements in multi-detector row CT scanners with respect to longitudinal coverage and gantry rotation time have increasingly reduced the cardiac and respiratory motion artifacts on pediatric cardiothoracic CT images acquired with this scan mode (5). Moreover, breath-hold scan, which exceedingly improves the image quality, has become possible in a subset of young children. The scan speed is proportional to a pitch value, which is defined as a ratio of table increment per 360° gantry rotation to beam collimation width. It is noteworthy that image quality and radiation dose may or may not be affected by the pitch value depending on the CT scanner used. To obtain a gapless scan dataset for image reconstruction, the upper pitch value is mostly limited to 1.5 for a single-source scan and 3.2 for a dual-source scan (31). In free-breathing young children with CHD, high-pitch dual-source non-ECG-synchronized spiral or helical scan further reduces cardiac and respiratory motion artifacts during cardiothoracic CT (53233). Nonetheless, ECG-synchronized data acquisition is necessary for detailed and accurate assessment of the coronary arteries and the ventricular function.
ECG-synchronized scan substantially improves the morphologic evaluation of the intracardiac structures, coronary arteries, aortic root, and pulmonary trunk. Appropriate cardiac phase selection is crucial in ensuring acquisition of motionless image quality of pediatric cardiothoracic CT: the mid-diastolic phase at low heart rate and the end-systolic phase at high heart rate. The definition of borderline heart rate, generally in the range of 65–80 bpm, differs among different institutions, and it also depends on temporal resolution of a CT scanner. For the ventricular function assessment, the end-systolic and end-diastolic phases should be obtained. The best cardiac phase is usually determined by visual analysis of the two-dimensional (2D) or three-dimensional (3D) multi-phase datasets or it may be suggested after automatic analysis of a 4D motion map where available (434). In general, the end-systolic, mid-diastolic, and end-diastolic phases are in the ranges of 35–50%, 65–80%, and 90–100%, respectively, of the RR interval. It is noteworthy that the trigger delays may indicate the starting point or the middle point of the acquisition window depending on a vendor. In subjects with arrhythmia, targeting the end-systolic phase with an absolute trigger delay in msec, where possible, is highly recommended to avoid image degradation; alternatively, a wider data acquisition window, in terms of percentage, with ECG editing may be necessary to capture the target cardiac phase at the expense of a higher radiation dose. To certain the proper end-systolic phase, the T wave position may be utilized as a landmark on ECG (456). There are three different ways to acquire ECG-synchronized CT dataset: retrospectively ECG-gated spiral or helical scan, prospectively ECG-triggered sequential scan, and prospectively ECG-triggered high-pitch dual-source spiral or helical scan.
Before the era of multi-detector row CT, electron-beam CT was used to obtain ECG-synchronized CT images by utilizing prospective ECG triggering (3536). However, electron-beam CT is no longer utilized because retrospectively ECG-gated spiral or helical CT provides excellent image quality, and is widely available (37). The major disadvantage of this scan mode is the high radiation dose resulting from the low pitch values (−0.2). Therefore, imagers involved in pediatric cardiothoracic CT should not only utilize all the available radiation dose reduction techniques including ECG-controlled tube current modulation and minimal scan range. Furthermore, functional evaluation utilizing this scan mode should be judiciously performed in children because it is associated with a high radiation dose. Breath-holding is usually recommended to avoid respiratory motion artifacts in retrospectively ECG-gated spiral or helical scan (4), but this scan mode may be used in free-breathing young children with a slightly high radiation dose and an acceptable image quality (152538).
In this scan mode first proposed in 2006 (39), sequential scans are acquired at a predefined time point in the cardiac cycle with prospective ECG triggering and at consecutive table positions (42638). The low radiation dose achieved by negligibly overlapped data acquisition is regarded to be the major merit of this scan mode (40). In contrast, as the name "step-and-shoot" implies, the prolonged examination time due to the required time intervals for table movement between sequential scans is the major drawback of this scan mode, which often produces "stair-step" misregistration artifacts due to respiration and heart rate variability between adjacent imaging stations. The number of sequential scans is inversely proportional to the longitudinal coverage; for example, a CT scanner with a 16-cm longitudinal coverage can cover the entire scan range in young children in a single sequential scan or one heartbeat (41). Based on many studies in adults (42), it is recommended to use this scan mode in patients with stable and low heart rates (≤ 70 bpm). Because of this recommendation and the concerns about the stair-step artifacts in CT scanners necessitating the use of more than two imaging slabs, this scan mode has been underutilized in free-breathing young children with high heart rates. End-systolic images obtained using this scan mode in children, unlike in adults, have excellent image quality, and the stair-step artifacts are less pronounced due to smaller tidal volumes and smaller time intervals between sequential scans at high heart rates. In addition, a difference in contrast enhancement may occur between adjacent slabs; however, this difference in children is usually trivial and does not substantially affect image interpretation. This scan mode can be used for ventricular function assessment if "padding," which allows for an extended acquisition window in the cardiac cycle, is utilized (434445).
Myocardial lesions with delayed enhancement can be demonstrated during the delayed phase (i.e., between 5 minutes and 15 minutes after intravenous administration of iodinated contrast agent) of ECG-synchronized CT with either retrospective ECG gating or prospective ECG triggering (46). Initial experience with this imaging technique in 29 consecutive children and young adults with suspected coronary artery or myocardial abnormality was recently reported (47). The study showed that sufficiently high contrast-to-noise ratios (10.3–25.4) could be achieved in delayed hyperenhancing myocardial lesions at low tube voltages (70–80 kV) and a shorter delay time (6–8 minutes); in addition, delayed-phase CT findings were equivalent to those of delayed-enhancement MRI in all cases and to those of thallium single-photon emission CT in 70% of the cases. This CT technique can be used as the "one-stop shop" examination in select patients when delayed enhancement cardiac MRI is not possible because of the presence of MRI-incompatible implants or in critically ill patients.
4D CT allows acquisitions of multiple consecutive 3D thoracic CT data with the same longitudinal scan range (up to 16 cm) without movement of the CT table. The scan time usually includes at least one respiratory cycle and is in the range of 2.0–4.6 seconds depending on the child's respiratory rate (6484950515253). Although respiratory rates in intubated patients were artificially manipulated in an institution in the United States of America (4852), 4D CT is generally performed during free breathing in most institutions. This scan technique may be used with or without intravenous injection of iodinated contrast agent. Simultaneous evaluation of the cardiovascular structures, the airways, and the lungs is feasible using contrast-enhanced 4D CT. In contrast, non-contrast 4D CT may be added to the cardiac CT protocol in select children.
Dual-energy scan, which utilizes two X-ray sources with two different energy spectra, was introduced with a first-generation dual-source CT scanner in 2006; other dual-energy scan techniques, including rapid tube voltage switching, use of a dual-layer detector, and use of a twin beam with a split filter, have been introduced since then (54). Initial experience of dual-energy lung perfusion CT in children showed that it is useful for detecting lung perfusion defects caused by pulmonary thromboembolism (55). This scan technique also may be used for evaluating lung ventilation using xenon gas inhalation in children (56). One study (57) demonstrated that 3-minutes delayed dual-energy CT can enhance the detection of silent thrombi in the extracardiac Fontan conduit by utilizing monochromatic 50 keV images. However, it should be noted that dual-energy CT images are acquired with a lower temporal resolution and are susceptible to various artifacts; both of these factors considerably degrade the image quality of the pediatric cardiothoracic CT. Therefore, dual-energy scan should be used as a complementary method in children with CHD.
In order to comply with the As Low As Reasonably Achievable principle, the radiation dose of pediatric cardiothoracic CT should be strictly optimized and adapted to the body size while maintaining the diagnostic image quality (4558). Body weight is the body size index that is commonly utilized for body size-adapted pediatric cardiothoracic CT protocols. However, the use of cross-sectional dimensions and body attenuation is highly recommended for a better body size adaptation (59). In this regard, a water-equivalent cross-sectional dimension seems to be the single best body size index for this purpose. In contrast, the patient age is not recommended as a body size index because of a broad overlap of body sizes as a function of age in children (60).
Attenuation-based tube current modulation is an essential radiation dose reduction technique, which allows approximately 16% reduction of the tube current without degradation of the image quality (61). Therefore, it should be always used during pediatric cardiothoracic CT examination when available. Imagers should recognize that the basic principles differ among different CT manufacturers (62), and the imagers should also determine the optimal level of image quality unique for each manufacturer (e.g., noise index for General Electric Healthcare, quality reference mAs for Siemens Healthineers, reference image for Philips Healthcare, or standard deviation for Canon Medical Systems) that they wish to achieve. Of interest, a recent study demonstrated that the user-determined level of image quality that was entered before the adjustment of the scan range resulted in an additional 18% reduction in the radiation dose compared with that entered after the adjustment of the scan range (13). As previously mentioned, patient isocentering is of critical importance in ensuring to take full benefits of tube current modulation and to avoid image degradation caused by off-centering. In addition, a suboptimal CT scout image should be repeated after changing the patient position or removing a radiopaque material from the patient to ensure accurate tube current modulation. The radiation dose parameters of a CT scout image also should be minimized to reduce radiation exposure to a patient.
The use of lower tube voltages is beneficial in pediatric cardiothoracic CT, because it produces a higher iodine contrast-to-noise ratio (456). From the radiation dose efficiency point of view, imagers should be careful to avoid tube current saturation at lower tube voltages during cardiothoracic CT using tube current modulation and fast gantry rotation speed (6364). It should be kept in mind that increased image noise at low tube voltage should be adequately compensated for by increasing the tube current.
The scan range of pediatric cardiothoracic CT should be adequately tailored to include anatomical structures of interest only; this is because the radiation dose is proportional to the longitudinal scan range. In the majority of cases, the scan range is similar to or slightly shorter than that of chest CT; the range generally includes the aortic arch superiorly and the bottom of the heart inferiorly. For the assessment of anomalous pulmonary venous connections, major aortopulmonary collateral arteries, or cavopulmonary connections, the lower neck and/or the upper abdomen should be included using a longer scan range as required.
Iterative reconstruction algorithms enabling image noise reduction without substantially affecting the anatomic details are useful in reducing the CT radiation dose, and the image quality is comparable to or better than that of filtered back projection (66566). It is not surprising that CT images reconstructed using these new algorithms are not always favorably ranked by radiologists with different and complex subjective criteria and opinions. In general, newer iterative reconstruction algorithms are better than old ones in terms of image noise reduction as well as preservation of anatomic details, contrast, and sharpness (6768). A lower limit in reducing radiation dose without image degradation or a point of diminishing returns by utilizing an iterative reconstruction algorithm was reported to be present; therefore, the image quality may begin to deteriorate below this limit (6970).
ECG-controlled tube current modulation or ECG pulsing is a useful method to reduce radiation dose for retrospectively ECG-gated spiral or helical scanning (456). Usually, the tube current outside a predefined window in the cardiac cycle can be reduced to 20% of the full tube current. A more aggressive mode of ECG pulsing is available, and ECG pulsing also can be utilized in prospectively ECG-triggered sequential scan in some CT models.
Adaptive collimation technology blocks radiation at both ends of spiral or helical scanning that is not used for image reconstruction. In most modern CT scanners, this function is usually turned on to reduce unnecessary radiation exposure due to z-overranging (671). To reduce radiation exposure to the breasts, an organ-based tube current modulation or a breast shield may be used. A breast shield is limited by the increased image noise in adjacent tissues (72), whereas the breasts may not be included in the reduced dose zone of organ-based tube current modulation (73).
A review of the data on the cumulative pediatric CT radiation dose over 5 years showed that the median value in children with CHD was relatively low (74). Based on the results of a multi-center, multi-vendor study on radiation dose of pediatric cardiothoracic CT, it has been suggested that sex, tube voltage, scan mode, and cardiac function assessment should be considered as essential radiation dose-influencing factors in developing the optimal pediatric cardiac CT protocols (75).
In CHD, all cardiovascular structures, including the left and the right side of the heart and the systemic and pulmonary vessels, need to be sufficiently and evenly visualized on pediatric cardiothoracic CT. Therefore, a tri-phasic injection protocol, in which an undiluted contrast agent is followed by a 30–60% diluted contrast agent and then by a saline chaser, is usually recommended to achieve optimal contrast enhancement and to reduce perivenous streak artifacts (576). If a 5% dilated contrast agent is used instead of saline in the third phase of the tri-phasic protocol, visualization of the thoracic systemic veins may be improved with decreasing perivenous artifacts (5). With regard to the injection site, the leg veins tend to show less perivenous streak artifacts than the arm veins do because the injected contrast agent is mixed gradually with unopacified blood while passing through abdominal visceral veins before arriving in the right atrium. When an arm vein is used, use of the right side can avoid streak artifacts around the left brachiocephalic vein; the streak artifacts are more pronounced and extensive in patients with bilateral superior vena cavae, in which the use of a right arm vein is highly recommended. It is difficult to achieve uniform vascular enhancement in patients with superior or total cavopulmonary connection because of the preferential blood flow and streaming (47778). To obtain optimal vascular enhancement in subjects with superior cavopulmonary connection, leg vein injection with a longer scan delay of approximately 50–60 seconds depending on the patient size is recommended. For imaging of total cavopulmonary connection or Fontan pathway, several injection methods for a single CT scan have been suggested to avoid two repeated (i.e., early and delayed) CT scans: simultaneous injection of an undiluted or a 50% diluted contrast agent through arm and leg veins (4798081); a 3-minutes delayed scanning irrespective of the site of intravenous injection (82); a venous two-phase or split injection method, in which 30–50% of the contrast agent is given and followed by a 30–60 seconds pause and then by the second injection of contrast agent (9); and arm, leg, or dual vein injection with a 60–70-second delayed scanning (7782). The last injection method appears to offer balanced opacification of all thoracic cardiovascular structures and requires a simple injection procedure. However, further comparative studies are needed to determine the best injection method in a patient with Fontan pathway.
With regard to the amount of iodinated contrast agent, 1.5–2.0 mL/kg of body weight is usually sufficient for pediatric cardiothoracic CT. A larger amount of contrast agent may be required in subjects with abundant shunt flow, severe valvular regurgitation, and severely enlarged cardiac chambers (4). In most cases, the use of a power injector is preferred to the use of hand injection. Hand injection may be used for a long and narrow central catheter, especially a peripherally inserted central line, and for an angiocatheter inserted into the hand or foot. Before power injection, unobstructed delivery of the saline flush and unrestricted return of blood should be confirmed to avoid extravasation of the contrast agent. Because patients with CHD often have right-to-left shunt, which has an increased risk of air embolism, particular attention should be paid to clearing air bubbles from the tubes and connections.
The maximum injection rate (1.5–4.0 mL/sec) of iodinated contrast agent depends on the size of the placed angiocatheter (5). In general, such high injection rates are not necessary in children with CHD, and lower injection rates (0.3–3.0 mL/sec) with longer scan delays (20–30 seconds) (except for subjects with superior or total cavopulmonary connection) are acceptable in attempting to achieve optimal and homogeneous cardiovascular enhancement, especially when scanning is performed using a low tube voltage (4569). Real-time pressure monitoring, when available, is quite helpful for early detection of malfunctioning intravenous line or extravasation of contrast agent, particularly in sedated children. Studies in adult (8384) have shown that small fenestrated catheters with side holes have promising results with respect to the injection rates, vascular enhancement, and safety; these catheters may be useful in patients with small and fragile peripheral veins. Further investigations are warranted to confirm whether equivalent effects would be observed in pediatric patients.
To determine the scan delay, an empirical method, a test-bolus injection method, or a bolus tracking method may be utilized. The use of a fixed delay appears to be inappropriate in children with CHD having complex hemodynamics. A test-bolus injection may accurately predict an optimal scan delay that reflects the individual's hemodynamics, but the use of an additional contrast agent and the increased radiation exposure in small children are its shortcomings. Bolus tracking is the most commonly used method in current clinical practice. The location of a region of interest for bolus tracking and the threshold attenuation to start CT scanning may be different according to each imager's strategy and the diagnostic purpose of the CT examination; a mid-cardiac level that shows the cardiac chambers is often used because complex hemodynamics of CHD can be easily appreciated during the monitoring scans (45611). A monitoring delay should be long enough to minimize additional radiation exposure from monitoring scans (4511). In addition, radiation dose parameters, including the tube voltage and the tube current, of the monitoring scan should be minimized (911).
To appropriately evaluate the complex cardiovascular anatomy in CHD, multiplanar and 3D reformations in addition to axial images should be used for image interpretation. A combination of maximum intensity projection, minimum intensity projection, and volume rendering (VR) visualization techniques is commonly utilized to highlight the target anatomic structures as required. Detailed descriptions of these techniques are beyond the scope of this article. Color-coded merging of multiple 3D segmented datasets is often useful to depict different anatomic substrates and their spatial relationships in a single 3D image; for example, this technique has been demonstrated in subjects with vascular airway compression and major aortopulmonary collateral arteries (5685). Transparent-lumen VR imaging is quite useful for illustrating intracardiac anatomy in children with CHD (86). Ventricular volumes can be quantified by using CT datasets in children with CHD (456458788). Recent studies have demonstrated that useful assessment of the pulmonary vascularity ratio can be provided using end-systolic cardiothoracic CT in patients with CHD (8990). Multi-phase cine 2D or 3D analysis may be used to evaluate not only the global and regional ventricular function, but also the respiratory dynamics of the airways, the lungs, and the diaphragms in patients with CHD (456484950515253869192). Post-processing, as CT scanning, should be performed by or under the supervision of an experienced radiologist, but it should not routinely be performed by a radiology technician along since this may lead to a misinterpretation due to a pseudo-lesion or a missed lesion. Accurate and detailed segmentation of 3D CT dataset is also crucial for developing 3D printed heart models (93).
A dual-source CT scanner is indeed a technical innovation in cardiac CT (93). The most distinguished advantage of this scanner type is the high temporal resolution, which is equivalent to a quarter of the gantry rotation time: 83 msec for a first-generation scanner; 75 msec for a second-generation scanner; and 66 msec for a third-generation scanner (1193), which is exceedingly useful in children with CHD and high heart rates. Another eminent imaging technique useful for pediatric cardiothoracic CT that exclusively available from this manufacturer is high-pitch dual-source spiral or helical scan mode that is available in second- and third-generation dual-source scanners (3194). The high-pitch scan mode considerably reduces cardiac and respiratory motion artifacts in children by drastically reducing the scan time, while maintaining the low radiation dose (9596). There are two types of high-pitch dual-source spiral or helical scan modes applicable to pediatric cardiothoracic CT: non-ECG-synchronized mode, pitch up to 3.2 (3132); and prospectively ECG-triggered mode, pitch up to 3.4 (979899). A recent study using a second-generation dual-source CT scanner (14) disclosed that additional ECG triggering did not substantially reduce cardiac motion artifacts on high-pitch dual-source spiral or helical pediatric cardiothoracic CT in young children with CHD.
A couple of radiation dose reduction techniques are available from this CT manufacturer, and some of them are exclusively available from this manufacturer. Combined longitudinal and transverse tube current modulation (CARE Dose 4D; Siemens Healthineers, Forchheim, Germany) is almost universally available in all types of CT scans. Organ-based tube current modulation may be utilized to reduce radiation exposure to the breasts when available. For retrospectively ECG-gated spiral or helical CT scan, two types of ECG-controlled tube current modulation are available; a conventional one offers 20% of full tube current outside pulsing window as in other CT manufacturers, whereas only 4% of the full tube current is produced outside the pulsing window in a more aggressive type (MinDose; Siemens Healthineers). Overall the radiation dose in dual-source retrospectively ECG-gated spiral or helical cardiothoracic CT can be reduced by approximately 15% by using the "chest pain" protocol, in which, unlike conventional protocol, a half of the radiation dose is utilized outside the heart (100). The study (100) also demonstrated that a tube current modulation curve over the whole scanning range provided in real-time was useful to avoid tube current saturation. Heart-rate-adaptive pitch allows a reduction of the radiation dose and the scan time in retrospectively ECG-gated spiral or helical scan. The availability of 70 kV is quite useful in reducing the radiation dose and increasing the iodine contrast-to-noise ratio in young children with CHD (101). ECG pulsing is also applicable in prospectively ECG-triggered sequential CT scans from this manufacturer as it is in retrospectively ECG-gated spiral or helical scans. Adaptive section collimation, which reduces the effect of z-overranging, is almost routinely available from this manufacturer (6).
The best systolic and diastolic phases can be automatically selected after retrospectively ECG-gated spiral or helical scan by using a 4D motion map (Cardio BestPhase; Siemens Healthineers), and this helps to simplify the workflow (33). Automatic tube voltage selection software (Care kV; Siemens Healthineers) helps imagers to optimize the CT radiation dose (102). The isocenter line displayed on the lateral scout view is very helpful in adjusting the vertical centering of a child before CT scanning. The extent of z-overranging is also indicated on CT scout images (6).
Three versions of iterative reconstruction algorithm are available in dual-source CT scanners for reducing image noise without substantially affecting the anatomic details. The latest version (ADMIRE; Siemens Healthineers) has been recently reported to improve the objective and subjective image quality when a low-dose 70 kV chest CT angiography is performed in young children (67).
A 320-detector-row CT scanner (Aquilion ONE; Canon Medical Systems, Otawara, Japan) with a 16 cm coverage in its z-axis was introduced in 2007. The 16-cm longitudinal coverage has several advantages when performing pediatric cardiac CT. In fact, the longitudinal coverage is usually long enough for the whole heart in older children and for the whole chest in young children. Because the total scan range in pediatric cardiac CT can be acquired in one gantry rotation in most of cases, cardiac and respiratory motion artifacts can be reduced. Moreover, sequential scanning in a single imaging slab can completely eliminate respiratory misregistration artifacts as well as differences in contrast enhancement between adjacent imaging slabs that often occur in several sequential scans during free breathing in other CT scanners with shorter z-axis coverage. For pediatric cardiac CT, four scan modes, which are target scan, prospective scan, and continuous scans with and without ECG modulation, may be utilized according to the heart rate and the required acquisition window in the cardiac cycle. Among them, the single-beat target scan with the minimum acquisition window is usually sufficient for pediatric cardiac CT (68).
The fastest gantry rotation time available from this vendor is 275 msec, and the temporal resolution of half reconstruction, which is approximately 140 msec, may be insufficient in patients with high heart rates. Notably, the vendor provides a unique CT imaging technique, called "variable helical pitch" scanning, which allows a seamless change of pitch during one continuous acquisition and enables the combination of ECG-gated and non-ECG-gated acquisition within one scan (103). The scan mode appears particularly useful in reducing the radiation exposure of pediatric cardiac CT requiring longer scan ranges such as the evaluation of the anomalous pulmonary venous connection and the Fontan pathway. Multiple wide volume scans may be used to acquire such longer scan ranges with low radiation dose, but the artifacts caused by the difference in contrast enhancement between the volumes are inevitable.
In planning the scan parameters of pediatric cardiothoracic CT, a user may be guided by the automatic software (SURE Cardio and SURE kV; Canon Medical Systems). In addition, the workflow can be simplified by utilizing automatic selection of the best cardiac phase instead of performing manual selection (PhaseXact; Canon Medical Systems). To reduce the radiation dose of pediatric cardiac CT, ECG-controlled tube current modulation in retrospectively ECG-gated spiral or helical scan and organ-based tube current modulation are available as in other vendors. The most recent version (FIRST; Canon Medical Systems) of iterative reconstruction algorithm can improve image quality of a low-dose ECG-gated pediatric cardiothoracic CT, compared with those an older version (68).
The most recent CT scanner (Revolution; GE Healthcare, Waukesha, WI, USA) can also provide a wide longitudinal coverage of up to 16 cm. As previously described, the scanner also has the advantages of one beat or single rotation sequential scanning; these advantages include the reduction of cardiac and respiratory motion artifacts as well as absence of respiratory misregistration and different-enhancement artifacts. The scan coverage of axial or sequential scanning can be adjusted by using variable collimation (4, 8, 10, 12, 14, and 16 cm), and this can reduce unnecessary radiation exposure to small children. The determination of the optimal CT scan parameters may be guided by utilizing software (Auto gating and kV Assist; GE Healthcare) as it is with other vendors. In addition, the best cardiac phase may be automatically selected (Smart Phase; GE Healthcare). Tube current modulation based on noise index and ECG-controlled tube current modulation are available to reduce the radiation dose of pediatric cardiac CT. Several versions of iterative reconstruction algorithm can be used to reduce image noise while maintaining anatomic details.
Of note, motion artifacts of the coronary arteries can be reduced by utilizing an intracycle motion correction algorithm (Snapshot Freeze; GE Healthcare), especially in the evaluation of the right coronary artery (104). To compensate for coronary motion, cardiac phases adjacent to the target phase within a single cardiac cycle are used in the algorithm. This technique is less vulnerable to beat-to-beat variability than multi-segment reconstruction is because the former operates on a single cycle (104). However, it remains to be determined whether the motion correction algorithm is also useful in pediatric cardiac CT.
A single-source dual-layer CT scanner with a unique spectral detector was recently introduced and allows dual-energy CT evaluation (105). Because basic ECG-synchronized scan options are available in Philips CT scanners, cardiovascular structures–including the coronary arteries–can be evaluated using end-systolic ECG-synchronized cardiac CT even in newborns with CHD (3715). Nevertheless, beta-blockers seem to be helpful to compensate for the limited temporal resolution (the gantry rotation of 0.27 second) of cardiac CT and to reduce the cardiac motion artifacts on pediatric cardiac CT images acquired at high heart rates. On the other hand, an adaptive multi-segment or multi-cycle reconstruction algorithm is not really effective in increasing the temporal resolution and is susceptible to variable heart rates or arrhythmias. The longitudinal coverage of the modern CT scanners produced by the vendor is in the range of 4.0–8.0 cm; therefore, the whole scan range of pediatric cardiac CT examination cannot be covered in one heartbeat or single gantry rotation with these scanners. A recently developed knowledge-based iterative reconstruction algorithm (IMR; Philips Healthcare, Cleveland, OH, USA) can improve the image quality of cardiac CT in infants with CHD; however, small structures, such as a small patent ductus arteriosus and coronary arteries, are blurred or missed (106).
We have provided in this article serves a comprehensive vendor-specific guideline with respect to the imaging techniques for cardiothoracic CT in children with CHD based on the consensus opinions of the experts the Asian Society of Cardiovascular Imaging. The first part of the guideline includes recommendations on patient preparation, scan techniques, radiation dose reduction techniques, intravenous iodinated contrast agent injection protocols, and post-processing techniques. Therefore, the guideline may be used to shape each user's own protocol, especially with reference to the same vendor-specific descriptions. Selecting a CT scanner for cardiac imaging is the heart of the matter (107) because it is really technically demanding to obtain excellent image quality of pediatric cardiothoracic CT. In this respect, the detailed comparative information of the most recent CT scanners described in this article may help a user to select a new CT scanner for pediatric cardiothoracic CT based on the specific institutional requirements.
References
1. Han BK, Lesser AM, Vezmar M, Rosenthal K, Rutten-Ramos S, Lindberg J, et al. Cardiovascular imaging trends in congenital heart disease: a single center experience. J Cardiovasc Comput Tomogr. 2013; 7:361–366. PMID: 24331931.


2. Yang JC, Lin MT, Jaw FS, Chen SJ, Wang JK, Shih TT, et al. Trends in the utilization of computed tomography and cardiac catheterization among children with congenital heart disease. J Formos Med Assoc. 2015; 114:1061–1068. PMID: 25241602.


3. Lee T, Tsai IC, Fu YC, Jan SL, Wang CC, Chang Y, et al. Using multidetector-row CT in neonates with complex congenital heart disease to replace diagnostic cardiac catheterization for anatomical investigation: initial experiences in technical and clinical feasibility. Pediatr Radiol. 2006; 36:1273–1282. PMID: 17036235.


4. Goo HW. State-of-the-art CT imaging techniques for congenital heart disease. Korean J Radiol. 2010; 11:4–18. PMID: 20046490.


5. Goo HW. Cardiac MDCT in children: CT technology overview and interpretation. Radiol Clin North Am. 2011; 49:997–1010. PMID: 21889018.


6. Goo HW. Current trends in cardiac CT in children. Acta Radiol. 2013; 54:1055–1062. PMID: 23104372.


7. Tsai IC, Chen MC, Jan SL, Wang CC, Fu YC, Lin PC, et al. Neonatal cardiac multidetector row CT: why and how we do it. Pediatr Radiol. 2008; 38:438–451. PMID: 18259739.


8. Han BK, Rigsby CK, Hlavacek A, Leipsic J, Nicol ED, Siegel MJ, et al. Computed tomography imaging in patients with congenital heart disease Part I: rationale and utility. An expert consensus document of the Society of Cardiovascular Computed Tomography (SCCT): endorsed by the Society of Pediatric Radiology (SPR) and the North American Society of Cardiac Imaging (NASCI). J Cardiovasc Comput Tomogr. 2015; 9:475–492. PMID: 26272851.
9. Han BK, Rigsby CK, Leipsic J, Bardo D, Abbara S, Ghoshhajra B, et al. Computed tomography imaging in patients with congenital heart disease, Part 2: technical recommendations. An expert consensus document of the Society of Cardiovascular Computed Tomography (SCCT): endorsed by the Society of Pediatric Radiology (SPR) and the North American Society of Cardiac Imaging (NASCI). J Cardiovasc Comput Tomogr. 2015; 9:493–513. PMID: 26679548.
10. Tsai IC, Goo HW. Cardiac CT and MRI for congenital heart disease in Asian countries: recent trends in publication based on a scientific database. Int J Cardiovasc Imaging. 2013; 29(Suppl 1):1–5. PMID: 23344910.


11. Booij R, Dijkshoorn ML, van Straten M, du Plessis FA, Budde RP, Moelker A, et al. Cardiovascular imaging in pediatric patients using dual source CT. J Cardiovasc Comput Tomogr. 2016; 10:13–21. PMID: 26524989.


12. Kaasalainen T, Palmu K, Reijonen V, Kortesniemi M. Effect of patient centering on patient dose and image noise in chest CT. AJR Am J Roentgenol. 2014; 203:123–130. PMID: 24951205.


13. Goo HW. Is it better to enter a volume CT dose index value before or after scan range adjustment for radiation dose optimization of pediatric cardiothoracic CT with tube current modulation? Korean J Radiol. 2018; 19:692–703. PMID: 29962875.


14. Goo HW. Image quality and radiation dose of high-pitch dual-source spiral cardiothoracic computed tomography in young children with congenital heart disease: comparison of non-electrocardiography synchronization and prospective electrocardiography triggering. Korean J Radiol. 2018; 19:1031–1041. PMID: 30386135.


15. Tsai IC, Lee T, Chen MC, Fu YC, Jan SL, Wang CC, et al. Visualization of neonatal coronary arteries on multidetector row CT: ECG-gated versus non-ECG-gated technique. Pediatr Radiol. 2007; 37:818–825. PMID: 17562037.


16. Kuo F, Plaza M, Saigal G. Inappropriate arm positioning during scout image acquisition resulting in increased radiation dose while performing a chest CT. Pediatr Radiol. 2012; 42:508–509. PMID: 22322628.


17. Diaz LK, Jones L. Sedating the child with congenital heart disease. Anesthesiol Clin. 2009; 27:301–319. PMID: 19703678.


18. Char D, Ramamoorthy C, Wise-Faberowski L. Cognitive dysfunction in children with heart disease: the role of anesthesia and sedation. Congenit Heart Dis. 2016; 11:221–229. PMID: 27228360.


19. Mahabadi AA, Achenbach S, Burgstahler C, Dill T, Fischbach R, Knez A, et al. Working group "Cardiac CT" of the German Cardiac Society. Safety, efficacy, and indications of beta-adrenergic receptor blockade to reduce heart rate prior to coronary CT angiography. Radiology. 2010; 257:614–623. PMID: 21084413.
20. Decramer I, Vanhoenacker PK, Sarno G, Van Hoe L, Bladt O, Wijns W, et al. Effects of sublingual nitroglycerin on coronary lumen diameter and number of visualized septal branches on 64-MDCT angiography. AJR Am J Roentgenol. 2008; 190:219–225. PMID: 18094315.


22. Kim JW, Goo HW. Coronary artery abnormalities in Kawasaki disease: comparison between CT and MR coronary angiography. Acta Radiol. 2013; 54:156–163. PMID: 23482350.


23. Goo HW, Park IS, Ko JK, Kim YH, Seo DM, Yun TJ, et al. Visibility of the origin and proximal course of coronary arteries on non-ECG-gated heart CT in patients with congenital heart disease. Pediatr Radiol. 2005; 35:792–798. PMID: 15886981.


24. Goo HW, Seo DM, Yun TJ, Park JJ, Park IS, Ko JK, et al. Coronary artery anomalies and clinically important anatomy in patients with congenital heart disease: multislice CT findings. Pediatr Radiol. 2009; 39:265–273. PMID: 19159923.


25. Ben Saad M, Rohnean A, Sigal-Cinqualbre A, Adler G, Paul JF. Evaluation of image quality and radiation dose of thoracic and coronary dual-source CT in 110 infants with congenital heart disease. Pediatr Radiol. 2009; 39:668–676. PMID: 19319514.


26. Goo HW, Yang DH. Coronary artery visibility in free-breathing young children with congenital heart disease on cardiac 64-slice CT: dual-source ECG-triggered sequential scan vs. single-source non-ECG-synchronized spiral scan. Pediatr Radiol. 2010; 40:1670–1680. PMID: 20464385.


27. Goo HW. Identification of coronary artery anatomy on dual-source cardiac computed tomography before arterial switch operation in newborns and young infants: comparison with transthoracic echocardiography. Pediatr Radiol. 2018; 48:176–185. PMID: 29032431.


28. Rigsby CK, deFreitas RA, Nicholas AC, Leidecker C, Johanek AJ, Anley P, et al. Safety and efficacy of a drug regimen to control heart rate during 64-slice ECG-gated coronary CTA in children. Pediatr Radiol. 2010; 40:1880–1889. PMID: 20499055.


29. Goo HW, Park IS, Ko JK, Kim YH, Seo DM, Yun TJ, et al. CT of congenital heart disease: normal anatomy and typical pathologic conditions. Radiographics. 2003; 23 Spec No:S147–S165. PMID: 14557509.


30. Goo HW, Park IS, Ko JK, Kim YH, Seo DM, Park JJ. Computed tomography for the diagnosis of congenital heart disease in pediatric and adult patients. Int J Cardiovasc Imaging. 2005; 21:347–365. discussion 367. PMID: 16015453.


31. Flohr TG, Leng S, Yu L, Aiimendinger T, Bruder H, Petersilka M, et al. Dual-source spiral CT with pitch up to 3.2 and 75 ms temporal resolution: image reconstruction and assessment of image quality. Med Phys. 2009; 36:5641–5653. PMID: 20095277.


32. Sriharan M, Lazoura O, Pavitt CW, Castellano I, Owens CM, Rubens MB, et al. Evaluation of high-pitch ungated pediatric cardiovascular computed tomography for the assessment of cardiac structures in neonates. J Thorac Imaging. 2016; 31:177–182. PMID: 27007667.


33. Kanie Y, Sato S, Tada A, Kanazawa S. Image quality of coronary arteries on non-electrocardiography-gated high-pitch dual-source computed tomography in children with congenital heart disease. Pediatr Cardiol. 2017; 38:1393–1399. PMID: 28689328.


34. Ruzsics B, Gebregziabher M, Lee H, Brothers RL, Allmendinger T, Vogt S, et al. Coronary CT angiography: automatic cardiac-phase selection for image reconstruction. Eur Radiol. 2009; 19:1906–1913. PMID: 19277670.


35. Nakanishi T, Ito K, Imazu M, Yamakido M. Evaluation of coronary artery stenoses using electron-beam CT and multiplanar reformation. J Comput Assist Tomogr. 1997; 21:121–127. PMID: 9022783.


36. Choi BW, Park YH, Choi JY, Choi BI, Kim MJ, Ryu SJ, et al. Using electron beam CT to evaluate conotruncal anomalies in pediatric and adult patients. AJR Am J Roentgenol. 2001; 177:1045–1049. PMID: 11641166.


37. Achenbach S, Ulzheimer S, Baum U, Kachelriess M, Ropers D, Giesler T, et al. Noninvasive coronary angiography by retrospectively ECG-gated multislice spiral CT. Circulation. 2000; 102:2823–2828. PMID: 11104739.


38. Jin KN, Park EA, Shin CI, Lee W, Chung JW, Park JH. Retrospective versus prospective ECG-gated dual-source CT in pediatric patients with congenital heart diseases: comparison of image quality and radiation dose. Int J Cardiovasc Imaging. 2010; 26(Suppl 1):63–73. PMID: 20044793.


39. Hsieh J, Londt J, Vass M, Li J, Tang X, Okerlund D. Step-and-shoot data acquisition and reconstruction for cardiac x-ray computed tomography. Med Phys. 2006; 33:4236–4248. PMID: 17153402.


40. Husmann L, Valenta I, Gaemperli O, Adda O, Treyer V, Wyss CA, et al. Feasibility of low-dose coronary CT angiography: first experience with prospective ECG-gating. Eur Heart J. 2008; 29:191–197. PMID: 18089704.


41. Al-Mousily F, Shifrin RY, Fricker FJ, Feranec N, Quinn NS, Chandran A. Use of 320-detector computed tomographic angiography for infants and young children with congenital heart disease. Pediatr Cardiol. 2011; 32:426–432. PMID: 21210093.


42. Hausleiter J, Meyer TS, Martuscelli E, Spagnolo P, Yamamoto H, Carrascosa P, et al. Image quality and radiation exposure with prospectively ECG-triggered axial scanning for coronary CT angiography: the multicenter, multivendor, randomized PROTECTION-III study. JACC Cardiovasc Imaging. 2012; 5:484–493. PMID: 22595156.
43. Labounty TM, Leipsic J, Min JK, Heilbron B, Mancini GB, Lin FY, et al. Effect of padding duration on radiation dose and image interpretation in prospectively ECG-triggered coronary CT angiography. AJR Am J Roentgenol. 2010; 194:933–937. PMID: 20308494.


44. Feuchtner G, Goetti R, Plass A, Baumueller S, Stolzmann P, Scheffel H, et al. Dual-step prospective ECG-triggered 128-slice dual-source CT for evaluation of coronary arteries and cardiac function without heart rate control: a technical note. Eur Radiol. 2010; 20:2092–2099. PMID: 20407896.


45. Goo HW. Serial changes in anatomy and ventricular function on dual-source cardiac computed tomography after the Norwood procedure for hypoplastic left heart syndrome. Pediatr Radiol. 2017; 47:1776–1786. PMID: 28879411.


46. Koyama Y, Matsuoka H, Mochizuki T, Higashino H, Kawakami H, Nakata S, et al. Assessment of reperfused acute myocardial infarction with two-phase contrast-enhanced helical CT: prediction of left ventricular function and wall thickness. Radiology. 2005; 235:804–811. PMID: 15833978.


47. Goo HW. Myocardial delayed-enhancement CT: initial experience in children and young adults. Pediatr Radiol. 2017; 47:1452–1462. PMID: 28534155.


48. Greenberg SB. Dynamic pulmonary CT of children. AJR Am J Roentgenol. 2012; 199:435–440. PMID: 22826409.


49. Goo HW. Advanced functional thoracic imaging in children: from basic concepts to clinical applications. Pediatr Radiol. 2013; 43:262–268. PMID: 23417252.


50. Goo HW, Drubach L, Lee EY. Imaging techniques. In : Coley BD, editor. Caffey's pediatric diagnostic imaging. 12th ed. Philadelphia, PA: Elsevier;2013. p. 506–518.
51. Tan JZ, Crossett M, Ditchfield M. Dynamic volumetric computed tomographic assessment of the young paediatric airway: initial experience of rapid, non-invasive, four-dimensional technique. J Med Imaging Radiat Oncol. 2013; 57:141–148. PMID: 23551770.


52. Greenberg SB, Dyamenahalli U. Dynamic pulmonary computed tomography angiography: a new standard for evaluation of combined airway and vascular abnormalities in infants. Int J Cardiovasc Imaging. 2014; 30:407–414. PMID: 24322888.


53. Goo HW. Four-dimensional CT of the diaphragm in children: initial experience. Korean J Radiol. 2018; 19:111–118. PMID: 29354007.


54. Goo HW, Goo JM. Dual-energy CT: new horizon in medical imaging. Korean J Radiol. 2017; 18:555–569. PMID: 28670151.


55. Goo HW. Initial experience of dual-energy lung perfusion CT using a dual-source CT system in children. Pediatr Radiol. 2010; 40:1536–1544. PMID: 20596701.


56. Goo HW. Dual-energy lung perfusion and ventilation CT in children. Pediatr Radiol. 2013; 43:298–307. PMID: 23417255.


57. Grewal J, Al Hussein M, Feldstein J, Kiess M, Ellis J, Human D, et al. Evaluation of silent thrombus after the Fontan operation. Congenit Heart Dis. 2013; 8:40–47. PMID: 22897869.


58. Goo HW. CT radiation dose optimization and estimation: an update for radiologists. Korean J Radiol. 2012; 13:1–11. PMID: 22247630.


59. Goo HW. Individualized volume CT dose index determined by cross-sectional area and mean density of the body to achieve uniform image noise of contrast-enhanced pediatric chest CT obtained at variable kV levels and with combined tube current modulation. Pediatr Radiol. 2011; 41:839–847. PMID: 21656275.


60. Kleinman PL, Strauss KJ, Zurakowski D, Buckley KS, Taylor GA. Patient size measured on CT images as a function of age at a tertiary care children's hospital. AJR Am J Roentgenol. 2010; 194:1611–1619. PMID: 20489103.


61. Goo HW, Suh DS. Tube current reduction in pediatric non-ECG-gated heart CT by combined tube current modulation. Pediatr Radiol. 2006; 36:344–351. PMID: 16501970.


62. Lee CH, Goo JM, Ye HJ, Ye SJ, Park CM, Chun EJ, et al. Radiation dose modulation techniques in the multidetector CT era: from basics to practice. Radiographics. 2008; 28:1451–1459. PMID: 18794318.


63. Goo HW, Suh DS. The influences of tube voltage and scan direction on combined tube current modulation: a phantom study. Pediatr Radiol. 2006; 36:833–840. PMID: 16642311.


64. Israel GM, Herlihy S, Rubinowitz AN, Cornfeld D, Brink J. Does a combination of dose modulation with fast gantry rotation time limit CT image quality? AJR Am J Roentgenol. 2008; 191:140–144. PMID: 18562737.


65. Kordolaimi SD, Argentos S, Pantos I, Kelekis NL, Efstathopoulos EP. A new era in computed tomographic dose optimization: the impact of iterative reconstruction on image quality and radiation dose. J Comput Assist Tomogr. 2013; 37:924–931. PMID: 24270114.
66. Han BK, Grant KL, Garberich R, Sedlmair M, Lindberg J, Lesser JR. Assessment of an iterative reconstruction algorithm (SAFIRE) on image quality in pediatric cardiac CT datasets. J Cardiovasc Comput Tomogr. 2012; 6:200–204. PMID: 22682262.


67. Rompel O, Glöckler M, Janka R, Dittrich S, Cesnjevar R, Lell MM, et al. Third-generation dual-source 70-kVp chest CT angiography with advanced iterative reconstruction in young children: image quality and radiation dose reduction. Pediatr Radiol. 2016; 46:462–472. PMID: 26739141.


68. Shirota G, Maeda E, Namiki Y, Bari R, Ino K, Torigoe R, et al. Pediatric 320-row cardiac computed tomography using electrocardiogram-gated model-based full iterative reconstruction. Pediatr Radiol. 2017; 47:1463–1470. PMID: 28667349.


69. Infante JC, Liu Y, Rigsby CK. CT image quality in sinogram affirmed iterative reconstruction phantom study - is there a point of diminishing returns? Pediatr Radiol. 2017; 47:333–341. PMID: 27891546.


70. Lee KB, Goo HW. Quantitative image quality and histogram-based evaluations of an iterative reconstruction algorithm at low-to-ultralow radiation dose levels: a phantom study in chest CT. Korean J Radiol. 2018; 19:119–129. PMID: 29354008.


71. Deak PD, Langner O, Lell M, Kalender WA. Effects of adaptive section collimation on patient radiation dose in multisection spiral CT. Radiology. 2009; 252:140–147. PMID: 19561253.


72. Kim YK, Sung YM, Choi JH, Kim EY, Kim HS. Reduced radiation exposure of the female breast during low-dose chest CT using organ-based tube current modulation and a bismuth shield: comparison of image quality and radiation dose. AJR Am J Roentgenol. 2013; 200:537–544. PMID: 23436842.


73. Taylor S, Litmanovich DE, Shahrzad M, Bankier AA, Gevenois PA, Tack D. Organ-based tube current modulation: are women’s breasts positioned in the reduced-dose zone? Radiology. 2015; 274:260–266. PMID: 25153159.


74. Lee E, Goo HW, Lee JY. Age- and gender-specific estimates of cumulative CT dose over 5 years using real radiation dose tracking data in children. Pediatr Radiol. 2015; 45:1282–1292. PMID: 25801905.
75. Hui PKT, Goo HW, Du J, Ip JJK, Kanzaki S, Kim YJ, et al. Asian consortium on radiation dose of pediatric cardiac CT (ASCI-REDCARD). Pediatr Radiol. 2017; 47:899–910. PMID: 28435986.


76. Litmanovich D, Zamboni GA, Hauser TH, Lin PJ, Clouse ME, Raptopoulos V. ECG-gated chest CT angiography with 64-MDCT and tri-phasic IV contrast administration regimen in patients with acute non-specific chest pain. Eur Radiol. 2008; 18:308–317. PMID: 17763855.


77. Goo HW. Haemodynamic findings on cardiac CT in children with congenital heart disease. Pediatr Radiol. 2011; 41:250–261. PMID: 21127855.


78. Ghadimi Mahani M, Agarwal PP, Rigsby CK, Lu JC, Fazeli Dehkordy S, Wright RA, et al. CT for assessment of thrombosis and pulmonary embolism in multiple stages of single-ventricle palliation: challenges and suggested protocols. Radiographics. 2016; 36:1273–1284. PMID: 27618316.


79. Greenberg SB, Bhutta ST. A dual contrast injection technique for multidetector computed tomography angiography of Fontan procedures. Int J Cardiovasc Imaging. 2008; 24:345–348. PMID: 17823851.


80. Prabhu SP, Mahmood S, Sena L, Lee EY. MDCT evaluation of pulmonary embolism in children and young adults following a lateral tunnel Fontan procedure: optimizing contrast-enhancement techniques. Pediatr Radiol. 2009; 39:938–944. PMID: 19471914.


81. Sandler KL, Markham LW, Mah ML, Byrum EP, Williams JR. Optimizing CT angiography in patients with Fontan physiology: single-center experience of dual-site power injection. Clin Radiol. 2014; 69:e562–e567. PMID: 25446326.


82. Park EA, Lee W, Chung SY, Yin YH, Chung JW, Park JH. Optimal scan timing and intravenous route for contrast-enhanced computed tomography in patients after Fontan operation. J Comput Assist Tomogr. 2010; 34:75–81. PMID: 20118726.


83. Johnson PT, Christensen GM, Fishman EK. I.v. contrast administration with dual source 128-MDCT: a randomized controlled study comparing 18-gauge nonfenestrated and 20-gauge fenestrated catheters for catheter placement success, infusion rate, image quality, and complications. AJR Am J Roentgenol. 2014; 202:1166–1170. PMID: 24848812.


84. Tamura A, Kato K, Kamata M, Suzuki T, Suzuki M, Nakayama M, et al. Selection of peripheral intravenous catheters with 24-gauge side-holes versus those with 22-gauge end-hole for MDCT: a prospective randomized study. Eur J Radiol. 2017; 87:8–12. PMID: 28065379.


85. Choo KS, Lee HD, Ban JE, Sung SC, Chang YH, Kim CW, et al. Evaluation of obstructive airway lesions in complex congenital heart disease using composite volume-rendered images from multislice CT. Pediatr Radiol. 2006; 36:219–223. PMID: 16391927.


86. Hong SH, Kim YM, Lee HJ. Three-dimensional endo-cardiovascular volume-rendered cine computed tomography of isolated left ventricular apical hypoplasia: a case report and literature review. Korean J Radiol. 2016; 17:79–82. PMID: 26798219.


87. Kim HJ, Goo HW, Park SH, Yun TJ. Left ventricle volume measured by cardiac CT in an infant with a small left ventricle: a new and accurate method in determining uni- or biventricular repair. Pediatr Radiol. 2013; 43:243–246. PMID: 22875206.


88. Goo HW, Park SH. Semiautomatic three-dimensional CT ventricular volumetry in patients with congenital heart disease: agreement between two methods with different user interaction. Int J Cardiovasc Imaging. 2015; 31(Suppl 2):223–232. PMID: 26319216.


89. Goo HW, Park SH. Pulmonary vascular volume ratio measured by cardiac computed tomography in children and young adults with congenital heart disease: comparison with lung perfusion scintigraphy. Pediatr Radiol. 2017; 47:1580–1587. PMID: 28646273.


90. Goo HW. Computed tomography pulmonary vascular volume ratio in children and young adults with congenital heart disease: the effect of cardiac phase. Pediatr Radiol. 2018; 48:915–922. PMID: 29572746.


91. Goo HW. Free-breathing cine CT for the diagnosis of tracheomalacia in young children. Pediatr Radiol. 2013; 43:922–928. PMID: 23417231.


92. Goo HW, Kim HJ. Detection of air trapping on inspiratory and expiratory phase images obtained by 0.3-second cine CT in the lungs of free-breathing young children. AJR Am J Roentgenol. 2006; 187:1019–1023. PMID: 16985151.


93. Yoo SJ, Spray T, Austin EH 3rd, Yun TJ, van Arsdell GS. Hands-on surgical training of congenital heart surgery using 3-dimensional print models. J Thorac Cardiovasc Surg. 2017; 153:1530–1540. PMID: 28268011.


94. Flohr TG, McCollough CH, Bruder H, Petersilka M, Gruber K, Süss C, et al. First performance evaluation of a dual-source CT (DSCT) system. Eur Radiol. 2006; 16:256–268. PMID: 16341833.


95. Bodelle B, Fischbach C, Booz C, Yel I, Frellesen C, Beeres M, et al. Free-breathing high-pitch 80kVp dual-source computed tomography of the pediatric chest: image quality, presence of motion artifacts and radiation dose. Eur J Radiol. 2017; 89:208–214. PMID: 28267541.
96. Lell MM, May M, Deak P, Alibek S, Kuefner M, Kuettner A, et al. High-pitch spiral computed tomography: effect on image quality and radiation dose in pediatric chest computed tomography. Invest Radiol. 2011; 46:116–123. PMID: 20856124.
97. Nie P, Wang X, Cheng Z, Ji X, Duan Y, Chen J. Accuracy, image quality and radiation dose comparison of high-pitch spiral and sequential acquisition on 128-slice dual-source CT angiography in children with congenital heart disease. Eur Radiol. 2012; 22:2057–2066. PMID: 22592808.


98. Zheng M, Zhao H, Xu J, Wu Y, Li J. Image quality of ultra-low-dose dual-source CT angiography using high-pitch spiral acquisition and iterative reconstruction in young children with congenital heart disease. J Cardiovasc Comput Tomogr. 2013; 7:376–382. PMID: 24331933.


99. Xu J, Zhao H, Wang X, Bai Y, Liu L, Liu Y, et al. Accuracy, image quality, and radiation dose of prospectively ECG-triggered high-pitch dual-source CT angiography in infants and children with complex coarctation of the aorta. Acad Radiol. 2014; 21:1248–1254. PMID: 25097011.


100. Goo HW. Comparison of chest pain protocols for electrocardiography-gated dual-source cardiothoracic CT in children and adults: the effect of tube current saturation on radiation dose reduction. Korean J Radiol. 2018; 19:23–31. PMID: 29353996.


101. Nakagawa M, Ozawa Y, Sakurai K, Shimohira M, Ohashi K, Asano M, et al. Image quality at low tube voltage (70 kV) and sinogram-affirmed iterative reconstruction for computed tomography in infants with congenital heart disease. Pediatr Radiol. 2015; 45:1472–1479. PMID: 26115723.


102. Niemann T, Henry S, Faivre JB, Yasunaga K, Bendaoud S, Simeone A, et al. Clinical evaluation of automatic tube voltage selection in chest CT angiography. Eur Radiol. 2013; 23:2643–2651. PMID: 23828227.


103. Matsumoto S, Yamada Y, Hashimoto M, Okamura T, Yamada M, Yashima F, et al. CT imaging before transcatheter aortic valve implantation (TAVI) using variable helical pitch scanning and its diagnostic performance for coronary artery disease. Eur Radiol. 2017; 27:1963–1970. PMID: 27562479.


104. Leipsic J, Labounty TM, Hague CJ, Mancini GB, O'Brien JM, Wood DA, et al. Effect of a novel vendor-specific motion-correction algorithm on image quality and diagnostic accuracy in persons undergoing coronary CT angiography without rate-control medications. J Cardiovasc Comput Tomogr. 2012; 6:164–171. PMID: 22551593.


105. Rajiah P, Abbara S, Halliburton SS. Spectral detector CT for cardiovascular applications. Diagn Interv Radiol. 2017; 23:187–193. PMID: 28302592.


106. Jia Q, Zhuang J, Jiang J, Li J, Huang M, Liang C. Image quality of CT angiography using model-based iterative reconstruction in infants with congenital heart disease: comparison with filtered back projection and hybrid iterative reconstruction. Eur J Radiol. 2017; 86:190–197. PMID: 28027746.


107. Lewis MA, Pascoal A, Keevil SF, Lewis CA. Selecting a CT scanner for cardiac imaging: the heart of the matter. Br J Radiol. 2016; 89:20160376. PMID: 27302494.


Table 1
Vendor-Neutral Check Points for Optimal Pediatric Cardiothoracic CT
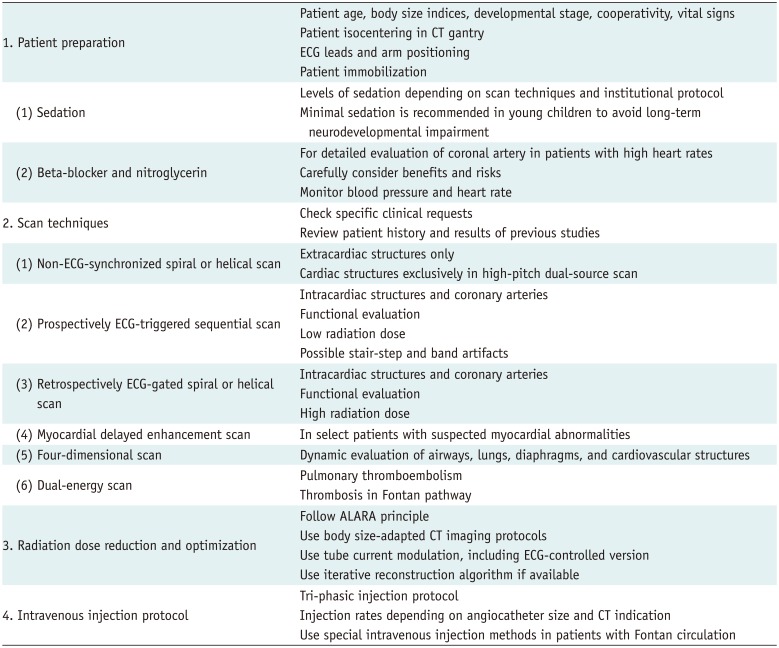
Table 2
Vendor-Specific Features for Pediatric Cardiothoracic CT
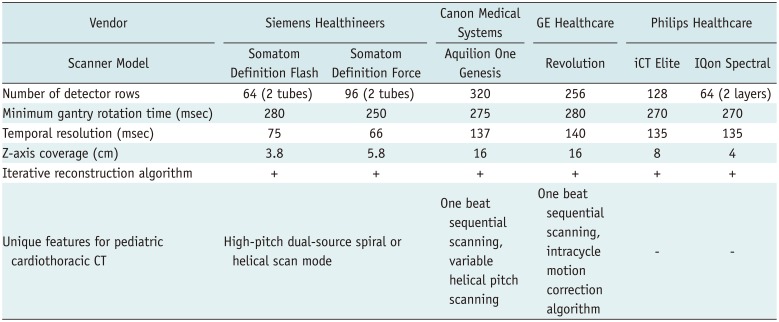