Abstract
The purpose of this animal study is to evaluate, by histomorphometric analysis, bone regeneration in rabbit's maxillary sinuses with blood clots alone, Bio-Oss, β-tricalcium phosphate (β-TCP), and demineralized tooth dentin (DTD) grafting. Bilateral sinus augmentation procedures were performed in 18 adult male rabbits. Rectangular replaceable bony windows were made with a piezoelectric thin saw insert. In the group 1, blood clots were filled; group 2, anorganic bovine graft (Bio-Oss) was grafted; group 3, β-TCP was grafted; group 4, DTD was grafted, and covered by replaceable bony windows. Animals were sacrificed at 2, 4, and 8 weeks after surgical procedure. The augmented sinuses were evaluated by histomorphometric analysis using hematoxylin and eosin and Masson's trichrome stains. Histologically, new bone formation was revealed along the elevated sinus membrane and all graft materials. The new bone area of the group 2 was significantly greater than the group 1, and of the group 3 was significantly greater than the group 2, and of the group 4 was significantly greater than the group 3 at 8 weeks with P<0.05. The bone marrow area of group 1 was significantly greater than other groups at 8 weeks. The DTD area was significantly lesser than Bio-Oss or β-TCP particles area at 8 weeks. This present study suggests that DTD can be effective graft materials for bone regeneration of the maxillary sinus augmentation.
Maxillary sinus augmentation is an established surgical procedure indicated for the improvement of posterior maxillary bone height when enough bone is not present for the installation of endosseous implants [12345]. The new compartment created between the floor of the maxillary sinus and the elevated sinus membrane is typically filled with autografts, allografts, xenografts, alloplasts, or combinations of different graft materials to maintain space for new bone formation [678]. Autograft has been considered to be the gold standard, because of its regenerative osteogenicity, osteoinductivity, and osteoconductivity [9]. However, many clinicians do not prefer autograft due to complications of donor site, limited amount of grafts and increased surgical time [1011]. Other graft materials such as bovine bone or synthetic bone have been an alternative choice over autogenous bone grafting. However, these substitutes are osteoconductive and mainly function as space makers with volume preservation [121314].
Demineralized autologous tooth dentin has been introduced as a new alternative to autogenous bone graft as it has osteoinductivity [151617]. Dentin is known to have similar inorganic and organic components to alveolar bone [18]. Demineralization of particulate dentin is known to release diverse growth factors to induce bone regeneration [19]. An extracted tooth from the patient can be used as noble bone graft material after appropriate decalcification and sterilization process, in block or particulate form, and it has been widely used for ridge and sinus augmentation [20]. However, the histomorphometric comparison of newly formed bone in the maxillary sinus with graft materials in use and demineralized tooth dentin is rare.
The purpose of this study was to compare bone regeneration after maxillary sinus augmentation using graft materials and demineralized tooth dentin by means of a histologic and histomorphometric evaluation.
This study used 18 adult male New Zealand white rabbits that weighed from 2.8 to 3.2 kg (average 3.0 kg) as experimental animals. This study was approved by the Animal Care and Use Committee at Daegu Catholic University Medical Center (DCIAFCR-160703-3-Y).
The rabbit's maxillary sinus filling material was divided into 4 groups, as follows: group 1, control, blood clots; group 2, anorganic bovine graft (Bio-Oss, Geistlich AG, Wolhusen, Switzerland); group 3, β-tricalcium phosphate (β-TCP; Cerasorb M, Curasan, Kleinostheim, Germany); and group 4, demineralized tooth dentin (DTD).
Extracted permanent teeth without caries or fillings were collected and soft tissue attached in teeth was removed with blade or rotary with coolant. After sterilization of teeth with sterilization reagent (peracetic acid ethanol solution) in a vacuum-ultrasonic device (VacuaSonic System, CosmoBio-Medicare Co., Seoul, Korea), the sterilized teeth were stored at −20℃ before preparing tooth bone. Teeth was crushed and into powders of 0.8–1.0 mm in size on experimental day and demineralization using 0.6 N hydrochloride was done for 15 minutes under vacuum compression and ultrasonic vibration. The DTD was then washed with phosphate buffered saline (PBS), sterilized with sterilization reagent and consecutively washed again with PBS and distilled water. All steps, including demineralization, washing, and were processed following manufacturer's instructions.
The 18 rabbits were used and three sinuses were assigned to each group. All rabbits received the same surgical procedures. General anesthesia was induced with a combination of 30 mg/kg ketamin (Ketalar, Yuhan Co., Seoul, Korea) and 10 mg/kg xylazine (Rompun, Bayer Korea, Seoul, Korea) intramuscularly, and 0.5 ml of lidocaine with 1;100,000 epinephrine was injected subcutaneously along the midline of the nasal dorsum. Each rabbit was stabilized on the surgical table and skin and periosteal incisions were made at the middle of the nasal dorsum to expose nasal bone and the nasoincisional suture line. A rectangular replaceable bony window, about 3 mm×10 mm, was made with a thin saw insert (S-Saw, Bukbu Dental Co., Daegu, Korea) connected with a piezoelectric device (Surgybone, Silfradent srl, Sofia, Italy). Two replaceable bony windows were made at both nasal bones and windows were located about 20 mm anterior to the nasofrontal suture line and 5 mm away from the middle suture line. The sinus mucosa was elevated with a blunt-ended curette carefully to avoid membrane perforation, anteroventrally to accommodate the bone graft. In the group 1, blood clot was filled in the new compartment under the elevated sinus membrane and the replaceable bony window was repositioned. In the group 2, approximately 0.25 cc of anorganic bovine graft (Bio-Oss, Geistlich AG) was grafted. In the group 3, β-TCP (Cerasorb M, Curasan) was grafted. In the group 4, DTD was grafted. Group 1 and group 2 were grafted in same rabbit's bilateral sinuses, and groups 3 and 4 were grafted in the other rabbit's bilateral sinuses. The muscular tissue was sutured with a nylon suture 4-0 (Blue nylon, Ailee Co., Busan, Korea). All animals were administered antibiotics intramuscularly with Gentamycin (Donghwa Co., Seoul, Korea), 20 mg/kg for 3 days postoperatively.
The rabbits were sacrificed at 2, 4, and 8 weeks under general anesthesia after all intramuscular injection. The augmented sinus was segmented with a microsaw from the cranium and fixed with neutral buffered formalin for 24 hours and washed with 0.1 M phosphate buffer solution and 10% formic acid for 10 days. The specimen was embedded in paraffin (Paraplast, Oxford, St. Louis, MO, USA) and sliced coronally into serial sections 5-µm thick. Both augmented sinuses were included in the specimen to compare at the same time. The specimens were stained with hematoxylin and eosin (H&E) and Masson's trichrome (MT) stains, and examined, under light microscopy, for newly formed bone and soft tissue changes in the new compartment of the maxillary sinus.
Ten randomly selected fields from each group were photographed using the AxioCam MRc5 (Carl Zeiss, Jena, Germany) interfaced with the Axiophot Photomicroscope (Carl Zeiss), and the AxioVision SE64 (Carl Zeiss) program was used for analysis. The following histomorphometric measurements were made: total augmented area, graft material (Bio-Oss, β-TCP, or DTD) area, newly formed bone area, mature lamella bone area, bone marrow area, and connective tissue area. In the group 1, the percentage of newly formed bone was analyzed as the percentage of newly formed bone area to the total augmented area. In the groups 2, 3, and 4, the percentage of newly formed bone was analyzed as the percentage of the newly formed bone area to the total augmented area, except for the Bio-Oss, β-TCP, or DTD particle area. The mature lamella bone was defined as a red color structure containing osteocytes in MT stain.
For the data processing and statistical evaluation, appropriate validated software was used (version 25.0, IBM Corp., Armonk, NY, USA). The statistical significance of differences between intra- and intergroup was evaluated by one-way analysis of variance (ANOVA) with Tukey method. The quantitative results were expressed as means±standard deviation and the P-value of <0.05 indicated statistical significance.
Bio-Oss and β-TCP particles were lightly stained, and DTD was stained with various colors, and all graft materials were well differentiated from surrounding tissue in H&E and MT stains. Host bone or lamella bone was stained red, and newly formed bone or woven bone was stained blue in MT stain. No signs of inflammation were shown in all groups by H&E and MT stains under light microscopy. New bone formation changed in the new compartment under the elevated sinus membrane throughout the experimental period in all groups (Fig. 1).
At 2 weeks, central areas of the new compartment under the elevated sinus membrane were filled with blood clots, and many blood vessels were revealed in the connective tissue around the blood clots. Active new bone formation was observed under the elevated sinus membrane and the floor of replaced bony window, and many osteoblasts were observed there (Figs. 1A, 2A). At 4 weeks, abundant new bone formation was seen in the new compartment of the maxillary sinus, and many osteocytes were seen in the newly formed bone. Blood vessels and some adipose tissue were observed around newly formed bone. Many osteoblasts and some osteoclasts were observed on the surface of the newly formed bone (Figs. 1E, 2B, C). At 8 weeks, more thickened new bone was found than at 4 weeks. Bone marrow around new bone was more prominent, and many active osteoblasts were revealed on the surface of newly formed bone. The density of the bone marrow was highly increased compared with that at 4 weeks. Most of newly formed woven bone was replaced by mature lamellar bone. The area of the maxillary sinus was decreased compared with that of 4-week group (Figs. 1I, 2D).
At 2 weeks postoperatively, new bone formation was revealed the floor of replaced bony window, under the surface of the elevated sinus membrane and around the lateral wall of the maxillary sinus. Newly formed bone was revealed partially on the surface of the Bio-Oss particles, and many osteoblasts and some osteoclasts were observed on the surface of newly formed bone (Figs. 1B, 3A). At 4 weeks, new bone on the floor of replaced bony window was thicker, and new bone formation increased along the Bio-Oss particles on the surface of the elevated sinus membrane and around the lateral wall of the maxillary sinus. Newly formed bone on the surface of the Bio-Oss particles increased compared with those of 2 weeks, and more soft tissue and blood vessels were evident around the Bio-Oss particles (Figs. 1F, 3B). Eight weeks after surgery, newly formed bone was thicker and observed on the most of Bio-Oss surfaces. A lot of mature lamellar bone was revealed inside the newly formed bone and space of bone marrow containing adipose tissue was observed on the floor of replaceable bone. The density of Bio-Oss particles was similar to that of the 4 weeks (Figs. 1J, 3C, D).
At 2 weeks, new bone formation was revealed the floor of replaced bony window and under the surface of the elevated sinus membrane. Central areas of the new compartment under the elevated sinus membrane were filled with blood coats, β-TCP particles and connective tissue.
Newly formed bone was revealed partially on the surface and inside of the β-TCP particles, and soft tissue with osteoblasts and blood vessels were observed around the newly formed bone (Figs. 1C, 4A). Four weeks after surgery, new bone formation increased along the β-TCP particles on the floor of replaced bony window and on the surface of the elevated sinus membrane. Newly formed bone on the surface and inside of the β-TCP particles increased compared with those of 2 weeks, and more soft tissue and blood vessels were evident around the β-TCP particles. Some mature lamellar bone was revealed inside the newly formed bone (Figs. 1G, 4B). At 8 weeks, the thickness and the density of new bone were highly increased, and abundant bone marrow tissues were observed around the newly formed bone. Most of newly formed woven bone in the new compartment of the maxillary sinus was replaced by mature lamellar bone. Many osteoblasts and some osteoclasts were observed on the surface of the newly formed bone and β-TCP particles. The size and the density of β-TCP particles were decreased compared with that at 4 weeks (Figs. 1K, 4C, D).
Two weeks after surgery, new bone formation was revealed the floor of replaced bony window and around the lateral wall of the maxillary sinus. Newly formed bone was expanded from the replaceable bone and revealed partially on the surface of the DTD. Many osteoblasts were observed on the surface of newly formed bone (Figs. 1D, 5A). At 4 weeks, new bone formation increased along the DTD on the floor of replaced bony window and in the central areas of the new compartment. Newly formed bone on the surface of the DTD was thicker and increased compared with those of 2 weeks, and more connective tissue and blood vessels were revealed around the DTD and the newly formed bone. Some mature lamellar bone was revealed inside the newly formed bone. The density of DTD was decreased compared with that at 2-week group (Figs. 1H, 5B). Eight weeks after the operation, the thickness and the density of new bone were highly increased at 4-week group. A lot of mature lamellar bone was revealed inside the newly formed bone and space of bone marrow containing adipose tissue was observed on the floor of replaceable bone and nasal bone. Many osteoblasts were observed on the surface of the newly formed bone and some osteoclasts were observed on the surface and around of the DTD. The size and the density of DTD were decreased compared with that at 4-week group (Figs. 1L, 5C, D).
In the group 1, the percentage of newly formed bone to the area of the augmented sinus at 2, 4, and 8 weeks was 8.46±1.88, 17.80±2.63, and 12.10±2.71%, respectively. One-way ANOVA and post hoc comparisons showed that the new bone area at 4 weeks was significantly greater than at 2 weeks, and 8 weeks was significantly lesser than at 4 weeks with P<0.05. In the groups 2, 3, and 4, the percentage of newly formed bone to the area of the augmented sinus at 2, 4, and 8 weeks was 16.09±1.52%, 18.91±1.96%, 19.65±1.81%; 15.05±1.21%, 20.13±2.30%, 20.73±1.99%; and 17.71±2.20%, 20.73±1.99%, 28.09±1.51%, respectively. In the group 2, the new bone area at 8 weeks was no significant different than at 2 weeks and 4 weeks with P<0.05. In the groups 3 and 4, the new bone area at 8 weeks was significantly greater than at 2 weeks and 4 weeks with P<0.05. The new bone area of the group 1 was significantly lesser than other groups at 2 weeks. The new bone area of the all groups was no significant different at 4 weeks. The new bone area of the group 2 was significantly greater than the group 1, and of the group 3 was significantly greater than the group 2, and of the group 4 was significantly greater than the group 3 at 8 weeks with P<0.05 (Fig. 6A).
In the groups 1, 2, 3, and 4, the percentage of mature lamella bone to the area of the augmented sinus at 4 and 8 weeks was 2.21±0.69%, 10.45±2.10%; 1.48±0.30%, 4.85±0.47%; 1.46±0.40%, 4.04±0.52%; and 1.31±0.30%, 4.70±0.59%, respectively. The mature lamella bone area of group 1 was significantly greater than other groups at 8 weeks with P<0.05 (Fig. 6B).
The total bone is the sum of the newly formed bone and the mature lamellar bone. In the group 1, the percentage of total bone to the area of the augmented sinus at 2, 4, and 8 weeks was 8.46±1.88%, 20.01±2.73%, and 22.55±3.07%, respectively. The total bone area at 4 weeks was significantly greater than at 2 weeks, and 8 weeks was no significant difference than at 4 weeks. In the groups 2, 3, and 4, the percentage of total bone to the area of the augmented sinus at 2, 4, and 8 weeks was 16.09±1.52%, 20.38±2.23%, 24.50±1.99%; 15.05±1.21%, 21.58±2.40%, 27.94±1.51%; and 17.71±2.20%, 22.04±2.25%, 32.79±1.54%, respectively. The total bone area at 8 weeks was significantly greater than at 2 weeks and 4 weeks with P<0.05. The total bone area of the group 1 was significantly lesser than other groups at 2 weeks. The total bone area of the all groups was no significant different at 4 weeks. The total bone area of the group 2 was no significant difference than the group 1, and of the group 3 was significantly greater than the group 2, and of the group 4 was significantly greater than the group 3 at 8 weeks with P<0.05 (Fig. 6C).
In the groups 1, 2, 3, and 4, the percentage of the bone marrow area of the augmented sinus at 4 and 8 weeks was 9.88±2.17%, 42.72±2.93%; 7.71±3.28%, 22.08±2.45%; 7.40±1.55%, 21.96±3.52%; and 7.35±3.15%, 29.67±3.22%, respectively. The bone marrow area of group 1 was significantly greater than other groups at 8 weeks. The bone marrow area of group 4 was significantly greater than groups 2 and 3 at 8 weeks with P<0.05 (Fig. 7A).
In the group 1, the percentage of the connective tissue area to the area of the augmented sinus at 2, 4, and 8 weeks was 91.54±1.88%, 70.11±3.84%, and 34.72±4.01%, respectively. In the groups 2, 3, and 4, the percentage of the connective tissue area to the area of the augmented sinus at 2, 4, and 8 weeks was 83.91±1.52%, 71.90±5.38%, 53.42±4.01%; 84.95±1.21%, 71.01±3.31%, 50.10±4.47%; and 82.29±2.20%, 70.61±5.11%, 37.54±3.66%, respectively. The connective tissue area of group 1 was significantly greater than other groups at 2 weeks. The connective tissue area of groups 1 and 4 were significantly lesser than groups 2 and 3 at 8 weeks with P<0.05 (Fig. 7B).
In the group 2, the percentage of augmented Bio-Oss to the area of the augmented sinus at 2, 4, and 8 weeks was 36.74±3.94%, 32.97±2.59%, and 35.21±3.16%, respectively, and no significant difference was found. In the group 3, the percentage of augmented β-TCP to the area of the augmented sinus at 2, 4, and 8 weeks was 37.19±3.08%, 29.43±3.20%, and 21.01±3.40%, respectively. The β-TCP area at 4 weeks was significantly lesser than at 2 weeks, and 8 weeks was significantly lesser than at 4 weeks. In the group 4, the percentage of augmented DTD to the area of the augmented sinus at 2, 4, and 8 weeks was 44.41±5.26%, 28.25±3.68%, and 14.04±3.17%, respectively. The DTD area at 4 weeks was significantly lesser than at 2 weeks, and 8 weeks was significantly lesser than at 4 weeks. The DTD area was significantly lesser than Bio-Oss or β-TCP area at 8 weeks with P<0.05 (Fig. 7C).
The grafting material is an important determinant of the success or failure of bone augmentation procedures. The present study, we evaluated the new bone formation in rabbit's maxillary sinuses using histologic and histomorphometric analysis with blood clots, Bio-Oss, β-TCP, and DTD.
Blood clots have been shown to contain endogenous growth factors, such as platelet-derived growth factor, fibroblast growth factor, insulin like growth factor, and transforming growth factor beta [21]. In the previous studies, good bone regeneration has been obtained with blood clots alone, without additional bone graft materials [222324]. However, our study showed that most of the newly formed bone in augmented sinus spaces had disappeared 8 weeks after blood-clots implantation. The space of augmented maxillary sinus remarkably decreased from 2 to 8 weeks. The air pressure moves the maxillary sinus membrane and affects the augmented bone structure [25]. The blood clots might not withstand the sinus pressure. In this study, blood clots alone showed a faster bone regeneration process, but we concluded that reduction of maxillary sinus space is insufficient to obtain sufficient amount of bone.
It requires the use of grafts to maintain the augmented space and to promote new bone formation [526]. The present study, we observed the process of new bone formation in reconstructed maxillary sinuses using two bioactive materials, Bio-Oss and β-TCP. Bio-Oss is a low-resorbable deproteinized bovine xenograft, chemically and physically identical to human bone, in the form of cortical granules, presenting 75%–80% porosity and a large-mesh interconnecting macroand micropore system that facilitates angiogenesis and osteoblast migration [13]. β-TCP consists of 1–2-mm-diameter spherical granules of >99% of pure β tricalcium phosphate with 5–20 mm interconnecting micropores and 40%–50% porosity, being absorbed by a combination of hydrolytic and cellular degradation processes [27]. However, both materials have a slight difference in bone regeneration process. The Bio-Oss particle is not replaced by new bone for a long time [2829], on the other hand, β-TCP particle has a faster absorption rate [30]. In this study, new bone formation was revealed on the surface of Bio-Oss and β-TCP particles and bone density was significantly increased from 2 to 8 weeks. However, the percentage of bone formation was significantly increased in β-TCP grafted group than in Bio-Oss grafted group at 8 weeks, and the amount of graft materials were significantly decreased in β-TCP grafted group from 4 to 8 weeks. These results indicate that β-TCP showed better bone regeneration results in rabbit's maxillary sinus augmentation.
The DTD is known to show faster bone regeneration and higher bone reformation because it underwent slow resorption, and osteogenic properties of diverse growth factors are released from tooth dentin [182031323334]. In this study, significantly higher new bone formation was revealed in the DTD grafted group at 8 weeks because demineralized tooth dentin revealed gradual resorption by osteoclasts around tooth dentin, and the volume of the tooth graft reduced over time during the bone regeneration process. Compared with β-TCP, the amount of total bone containing mature lamellar bone was significantly increased and the amount of demineralized tooth dentin was significantly decreased. Since the allogenic use of demineralized dentin for bone regeneration in 1975, demineralized tooth dentin has been used as a novel bone graft as an alternative to the autogenous bone in block and particulate form [353637383940]. A tooth is composed of an organic matrix and an inorganic component as similar as the alveolar bone. Among the organic components in dentin, 90% type I collagens and diverse growth factors such as bone morphogenetic proteins are included [18414243]. Among the inorganic components, hydroxyapatite and tricalcium phosphate show osteoconductive property and can be remodeled to bone when grafted [36]. Because noncollagenous proteins are known to trigger bone resorption and generation processes, phosphophoryn, sialoprotein, glycoprotein, proteoglycan, osteopontin, osteocalcin, and dentin matrix protein-1 are expressed in dentin, and these noncollagenous proteins in dentin and bone are known to play an important role in the mineralization of these tissues [44454647]. Demineralized dentin is known to be more active in bone induction than mineralized dentin and decreases its antigenicity [1948]. In addition, the demineralization process lowers the high crystallinity of hydroxyapatite, which enhances osteoblast adhesion and increases the resorption rate of dentin material to allow bone remodeling after bone graft [49]. Among the various decalcification methods of dentin introduced by several researchers, demineralization of dentin using 0.6 N HCl revealed favorable osteoinduction and led to bone formation in muscle and skin connective tissues [4850515253545556]. Reducing crystallinity of hydroxyapatite is known to promote osteoblast adhesion [57]. High crystallinity of hydroxyapatite has osteoconductive property because complete bone remodeling is not achieved. Therefore, demineralization time of dentin is an important factor when reducing the crystallinity of hydroxyapatite. According to previous research on demineralization using the vacuum-ultrasonic device, 15-minute demineralization time for dentin was required because 15-minute decalcification maintains appropriate mineral content, crystallinity of hydroxyapatite, and organic structure for partially mineralized organic graft material [49].
The present study used rabbits' maxillary sinuses, known to be similar to human sinuses, to compare new bone formation for various graft materials histologically in the sinus. This study suggests that demineralized tooth dentin can be effective graft materials for bone regeneration of the maxillary sinus augmentation.
Figures and Tables
Fig. 1
Low magnification images of the augmented maxillary sinus in the rabbit at 2 weeks (A–D), 4 weeks (E–H), and 8 weeks (I–L) after surgery. Group 1 (A, E, I) was filled blood clots; and group 2 (B, F, J), group 3 (C, G, K), and group 4 (D, H, L) was grafted Bio-Oss particles, β-TCP particles and demineralized tooth dentin in the augmented maxillary sinus, respectively. β-TCP, β-tricalcium phosphate; NS, nasal septum. Masson trichrome stain (×12.5).

Fig. 2
Photomicrograph showing the bone formation of the group 1 at 2 weeks (A), 4 weeks (B, C), and 8 weeks (D). (A) Newly formed bone was found below the replaceable bony window and capillaries were found in the connective tissue at 2 weeks. (B) Abundant new bone formation was seen in the new compartment of the maxillary sinus, and many osteocytes were seen in the newly formed bone. (C) Many osteoblasts (arrows) and some osteoclasts (arrowheads) were observed on the surface of the newly formed bone at 4 weeks. (D) BM around new bone was more prominent, and many active osteoblasts were revealed on the surface of newly formed bone. Most of newly formed woven bone was replaced by mature lamellar bone at 8 weeks. BM, bone marrow; LB, lamella bone; N, newly formed bone; rB, replaceable bone. A, B, D, Masson trichrome stain (×100); C, hematoxylin and eosin stain (×200).
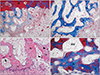
Fig. 3
Photomicrograph showing the bone formation of the group 2 at 2 weeks (A), 4 weeks (B), and 8 weeks (C, D). (A) New bone formation was revealed the floor of replaced bony window and newly formed bone was revealed partially on the surface of the Bio-Oss particles. Many osteoblasts and some osteoclasts were observed on the surface of newly formed bone at 2 weeks. (B) Newly formed bone was thicker and more soft tissue and blood vessels were evident around the Bio-Oss particles at 4 weeks. (C) At 8 weeks the density of transplant did not change significantly. Newly formed bone on the most of Bio-Oss surfaces was thicker and the density of Bio-Oss particles did not change significantly at 8 weeks. (D) A lot of mature lamellar bone was revealed inside the newly formed bone and abundant BM tissues were observed around the newly formed bone at 8 weeks. BM, bone marrow; BP, Bio-Oss particles; LB, lamella bone; N, newly formed bone; rB, replaceable bone. A, B, D, Masson trichrome stain (×100); C, hematoxylin and eosin stain (×50).
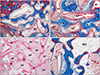
Fig. 4
Photomicrograph showing the bone formation of the group 3 at 2 weeks (A), 4 weeks (B), and 8 weeks (C, D). (A) New bone formation was revealed the floor of replaced bony window and newly formed bone was revealed partially on the surface and inside of the β-TCP particles at 2 weeks. (B) Newly formed bone on the surface and inside of the β-TCP particles increased and more soft tissue and blood vessels were evident around the β-TCP particles at 4 weeks. (C) The thickness and the density of new bone were highly increased, and abundant BM tissues were observed around the newly formed bone at 8 weeks. (D) Many osteoblasts (arrows) and some osteoclasts (arrowheads) were observed on the surface of the newly formed bone and β-TCP particles at 8 weeks. BM, bone marrow; β-TCP, β-tricalcium phosphate; LB, lamella bone; N, newly formed bone; rB, replaceable bone; TP, β-TCP particles. A–C, Masson trichrome stain (×100); D, hematoxylin and eosin stain (×200).
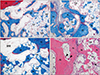
Fig. 5
Photomicrograph showing the bone formation of the group 4 at 2 weeks (A), 4 weeks (B), and 8 weeks (C, D). (A) New bone formation was revealed the floor of replaced bony window and newly formed bone was revealed on the surface of the DTD at 2 weeks. (B) Newly formed bone on the surface of the DTD was thicker and increased and more connective tissue and blood vessels were revealed around the DTD and the newly formed bone at 4 weeks. (C) The thickness and the density of new bone were highly increased. A lot of mature lamellar bone was revealed inside the newly formed bone and space of BM containing adipose tissue was observed at 8 weeks. (D) Some osteoclasts (arrowheads) were observed on the surface and around of the DTD and many osteoblasts (arrows) were observed on the surface of the newly formed bone at 8 weeks. BM, bone marrow; DTD, demineralized tooth dentin; LB, lamella bone; N, newly formed bone; rB, replaceable bone. A, C, Masson trichrome stain (×100); B, hematoxylin and eosin (H&E) stain (×100); D, H&E stain (×200).
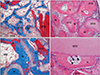
Fig. 6
(A) Histomorphometric measurement of the area of newly formed bone to the area of the augmented sinus. (B) Histomorphometric measurement of the area of mature lamellar bone to the area of newly formed woven bone at 4 and 8 weeks. (C) Histomorphometric measurement of the area of total bone to the area of the augmented sinus. The total bone is the sum of the newly formed bone and the lamellar bone (*P<0.05).
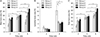
Fig. 7
(A) Histomorphometric measurement of the area of bone marrow to the area of the augmented sinus at 4 and 8 weeks. (B) Histomorphometric measurement of the area of connective tissue to the area of the augmented sinus. (C) Histomorphometric measurement of the area of Bio-Oss particle, β-TCP particle or demineralized tooth dentin to the area of the augmented sinus (*P<0.05). β-TCP, β-tricalcium phosphate.
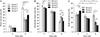
References
1. Boyne PJ, James RA. Grafting of the maxillary sinus floor with autogenous marrow and bone. J Oral Surg. 1980; 38:613–616.
2. Tatum H Jr. Maxillary and sinus implant reconstructions. Dent Clin North Am. 1986; 30:207–229.
3. Misch CE. Maxillary sinus augmentation for endosteal implants: organized alternative treatment plans. Int J Oral Implantol. 1987; 4:49–58.
4. Chanavaz M. Maxillary sinus: anatomy, physiology, surgery, and bone grafting related to implantology: eleven years of surgical experience (1979–1990). J Oral Implantol. 1990; 16:199–209.
5. Smiler DG, Johnson PW, Lozada JL, Misch C, Rosenlicht JL, Tatum OH Jr, Wagner JR. Sinus lift grafts and endosseous implants. Treatment of the atrophic posterior maxilla. Dent Clin North Am. 1992; 36:151–186.
6. Hürzeler MB, Kirsch A, Ackermann KL, Quiñones CR. Reconstruction of the severely resorbed maxilla with dental implants in the augmented maxillary sinus: a 5-year clinical investigation. Int J Oral Maxillofac Implants. 1996; 11:466–475.
7. Cordioli G, Mazzocco C, Schepers E, Brugnolo E, Majzoub Z. Maxillary sinus floor augmentation using bioactive glass granules and autogenous bone with simultaneous implant placement. Clinical and histological findings. Clin Oral Implants Res. 2001; 12:270–278.
8. Aghaloo TL, Moy PK. Which hard tissue augmentation techniques are the most successful in furnishing bony support for implant placement? Int J Oral Maxillofac Implants. 2007; 22:Suppl. 49–70.
9. Tadic D, Epple M. A thorough physicochemical characterisation of 14 calcium phosphate-based bone substitution materials in comparison to natural bone. Biomaterials. 2004; 25:987–994.


11. De Menezes Oliveira MA, Torres CP, Gomes-Silva JM, Chinelatti MA, De Menezes FC, Palma-Dibb RG, Borsatto MC. Microstructure and mineral composition of dental enamel of permanent and deciduous teeth. Microsc Res Tech. 2010; 73:572–577.


12. Ozyuvaci H, Bilgic B, Firatli E. Radiologic and histomorphometric evaluation of maxillary sinus grafting with alloplastic graft materials. J Periodontol. 2003; 74:909–915.


13. Orsini G, Traini T, Scarano A, Degidi M, Perrotti V, Piccirilli M, Piattelli A. Maxillary sinus augmentation with Bio-Oss particles: a light, scanning, and transmission electron microscopy study in man. J Biomed Mater Res B Appl Biomater. 2005; 74:448–457.


14. Zijderveld SA, Zerbo IR, van den Bergh JP, Schulten EA, ten Bruggenkate CM. Maxillary sinus floor augmentation using a beta-tricalcium phosphate (Cerasorb) alone compared to autogenous bone grafts. Int J Oral Maxillofac Implants. 2005; 20:432–440.
15. Chesmel KD, Branger J, Wertheim H, Scarborough N. Healing response to various forms of human demineralized bone matrix in athymic rat cranial defects. J Oral Maxillofac Surg. 1998; 56:857–863.


16. Nampo T, Watahiki J, Enomoto A, Taguchi T, Ono M, Nakano H, Yamamoto G, Irie T, Tachikawa T, Maki K. A new method for alveolar bone repair using extracted teeth for the graft material. J Periodontol. 2010; 81:1264–1272.


17. Kim YK, Kim SG, Byeon JH, Lee HJ, Um IU, Lim SC, Kim SY. Development of a novel bone grafting material using autogenous teeth. Oral Surg Oral Med Oral Pathol Oral Radiol Endod. 2010; 109:496–503.


18. Yeomans JD, Urist MR. Bone induction by decalcified dentine implanted into oral, osseous and muscle tissues. Arch Oral Biol. 1967; 12:999–1008.


19. Murata M, Kawai T, Kawakami T, Akazawa T, Tazaki J, Ito K, Kusano K, Arisue M. Human acid-insoluble dentin with BMP-2 accelerates bone induction in subcutaneous and intramuscular tissues. J Ceram Soc Jpn. 2010; 118:438–441.


20. Kim YK, Lee J, Um IW, Kim KW, Murata M, Akazawa T, Mitsugi M. Tooth-derived bone graft material. J Korean Assoc Oral Maxillofac Surg. 2013; 39:103–111.


21. Lynch SE, Colvin RB, Antoniades HN. Growth factors in wound healing: single and synergistic effects on partial thickness porcine skin wounds. J Clin Invest. 1989; 84:640–646.


22. Jensen OT, Greer RO Jr, Johnson L, Kassebaum D. Vertical guided bone-graft augmentation in a new canine mandibular model. Int J Oral Maxillofac Implants. 1995; 10:335–344.


23. Smukler H, Barboza EP, Burliss C. A new approach to regeneration of surgically reduced alveolar ridges in dogs: a clinical and histologic study. Int J Oral Maxillofac Implants. 1995; 10:537–551.
24. Leghissa GC, Zaffe D, Assenza B, Botticelli AR. Guided bone regeneration using titanium grids: report of 10 cases. Clin Oral Implants Res. 1999; 10:62–68.


25. Asai S, Shimizu Y, Ooya K. Maxillary sinus augmentation model in rabbits: effect of occluded nasal ostium on new bone formation. Clin Oral Implants Res. 2002; 13:405–409.


26. Small SA, Zinner ID, Panno FV, Shapiro HJ, Stein JI. Augmenting the maxillary sinus for implants: report of 27 patients. Int J Oral Maxillofac Implants. 1993; 8:523–528.
27. Wiltfang J, Merten HA, Schlegel KA, Schultze-Mosgau S, Kloss FR, Rupprecht S, Kessler P. Degradation characteristics of alpha and beta tri-calcium-phosphate (TCP) in minipigs. J Biomed Mater Res. 2002; 63:115–121.
28. Berglundh T, Lindhe J. Healing around implants placed in bone defects treated with Bio-Oss. An experimental study in the dog. Clin Oral Implants Res. 1997; 8:117–124.


29. Shin HI, Sohn DS. A method of sealing perforated sinus membrane and histologic finding of bone substitutes: a case report. Implant Dent. 2005; 14:328–333.


30. Walsh WR, Vizesi F, Michael D, Auld J, Langdown A, Oliver R, Yu Y, Irie H, Bruce W. Beta-TCP bone graft substitutes in a bilateral rabbit tibial defect model. Biomaterials. 2008; 29:266–271.
31. Butler WT, Mikulski A, Urist MR, Bridges G, Uyeno S. Noncollagenous proteins of a rat dentin matrix possessing bone morphogenetic activity. J Dent Res. 1977; 56:228–232.


32. Catanzaro-Guimarães SA, Catanzaro Guimarães BP, Garcia RB, Alle N. Osteogenic potential of autogenic demineralized dentin implanted in bony defects in dogs. Int J Oral Maxillofac Surg. 1986; 15:160–169.


33. Conover MA, Urist MR. Transmembrane bone morphogenesis by implants of dentin matrix. J Dent Res. 1979; 58:1911.


34. Gomes MF, dos Anjos MJ, Nogueira TO, Guimarães SA. Histologic evaluation of the osteoinductive property of autogenous demineralized dentin matrix on surgical bone defects in rabbit skulls using human amniotic membrane for guided bone regeneration. Int J Oral Maxillofac Implants. 2001; 16:563–571.
35. Nordenram A, Bang G, Bernhoft CH. A clinical-radiographic study of allogenic demineralized dentin implants in cystic jaw cavities. Int J Oral Surg. 1975; 4:61–64.


36. Kim ES. Autogenous fresh demineralized tooth graft prepared at chairside for dental implant. Maxillofac Plast Reconstr Surg. 2015; 37:8.


37. Lee KH, Kim YK, Cho WJ, Um IW, Murata M, Mitsugi M. Autogenous tooth bone graft block for sinus augmentation with simultaneous implant installation: a technical note. J Korean Assoc Oral Maxillofac Surg. 2015; 41:284–289.


38. Kim YK, Lee JH, Um IW, Cho WJ. Guided bone regeneration using demineralized dentin matrix: long-term follow-up. J Oral Maxillofac Surg. 2016; 74:515.e1–515.e9.


39. Kim YK, Pang KM, Yun PY, Leem DH, Um IW. Long-term follow-up of autogenous tooth bone graft blocks with dental implants. Clin Case Rep. 2017; 5:108–118.


40. Lee J, Lee EY, Park EJ, Kim ES. An alternative treatment option for a bony defect from large odontoma using recycled demineralization at chairside. J Korean Assoc Oral Maxillofac Surg. 2015; 41:109–115.


41. Robinson C, Weatherell JA, Hallsworth AS. Variatoon in composition of dental enamel within thin ground tooth sections. Caries Res. 1971; 5:44–57.
42. Bessho K, Tagawa T, Murata M. Comparison of bone matrix-derived bone morphogenetic proteins from various animals. J Oral Maxillofac Surg. 1992; 50:496–501.


43. Hoeppner LH, Secreto F, Jensen ED, Li X, Kahler RA, Westendorf JJ. Runx2 and bone morphogenic protein 2 regulate the expression of an alternative Lef1 transcript during osteoblast maturation. J Cell Physiol. 2009; 221:480–489.


44. Feng JQ, Luan X, Wallace J, Jing D, Ohshima T, Kulkarni AB, D'Souza RN, Kozak CA, MacDougall M. Genomic organization, chromosomal mapping, and promoter analysis of the mouse dentin sialophosphoprotein (Dspp) gene, which codes for both dentin sialoprotein and dentin phosphoprotein. J Biol Chem. 1998; 273:9457–9464.


45. Ritchie HH, Ritchie DG, Wang LH. Six decades of dentinogenesis research: historical and prospective views on phosphophoryn and dentin sialoprotein. Eur J Oral Sci. 1998; 106:Suppl 1. 211–220.
46. Handschin AE, Egermann M, Trentz O, Wanner GA, Kock HJ, Zund G, Trentz OA. Cbfa-1 (Runx-2) and osteocalcin expression by human osteoblasts in heparin osteoporosis in vitro. Clin Appl Thromb Hemost. 2006; 12:465–472.
47. Ye L, MacDougall M, Zhang S, Xie Y, Zhang J, Li Z, Lu Y, Mishina Y, Feng JQ. Deletion of dentin matrix protein-1 leads to a partial failure of maturation of predentin into dentin, hypomineralization, and expanded cavities of pulp and root canal during postnatal tooth development. J Biol Chem. 2004; 279:19141–19148.


48. Huggins CB, Urist MR. Dentin matrix transformation: rapid induction of alkaline phosphatase and cartilage. Science. 1970; 167:896–898.


49. Park M, Mah YJ, Kim DH, Kim ES, Park EJ. Demineralized deciduous tooth as a source of bone graft material: its biological and physicochemical characteristics. Oral Surg Oral Med Oral Pathol Oral Radiol. 2015; 120:307–314.


50. Urist MR. Bone histogenesis and morphogenesis in implants of demineralized enamel and dentin. J Oral Surg. 1971; 29:88–102.
51. Inoue T, Deporter DA, Melcher AH. Induction of cartilage and bone by dentin demineralized in citric acid. J Periodontal Res. 1986; 21:243–255.


52. Bang G. Induction of heterotopic bone formation by demineralized dentin in guinea pigs: antigenicity of the dentin matrix. J Oral Pathol. 1972; 1:172–185.


53. Bang G. Induction of heterotopic bone formation by demineralized dentin: an experimental model in guinea pigs. Scand J Dent Res. 1973; 81:240–250.


54. Urist MR, Dowell TA, Hay PH, Strates BS. Inductive substrates for bone formation. Clin Orthop Relat Res. 1968; 59:59–96.


55. Reddi AH, Anderson WA. Collagenous bone matrix-induced endochondral ossification hemopoiesis. J Cell Biol. 1976; 69:557–572.

