Abstract
Genetically engineered mouse models are commonly preferred for studying the human disease due to genetic and pathophysiological similarities between mice and humans. In particular, Cre-loxP system is widely used as an integral experimental tool for generating the conditional. This system has enabled researchers to investigate genes of interest in a tissue/cell (spatial control) and/or time (temporal control) specific manner. A various tissue-specific Cre-driver mouse lines have been generated to date, and new Cre lines are still being developed. This review provides a brief overview of Cre-loxP system and a few commonly used promoters for expression of tissue-specific Cre recombinase. Also, we finally introduce some available links to the Web sites that provides detailed information about Cre mouse lines including their characterization.
Cre (Cre recombinase) is one of the tyrosine site-specific recombinases (T-SSRs) including flipase (Flp) and D6 specific recombinase (Dre) [12]. it was discovered as a 38-kDa DNA recombinase produced from cre (cyclization recombinase) gene of bacteriophage P1 [3456]. It recognizes the specific DNA fragment sequences called loxP (locus of x-over, P1) site and mediates site-specific deletion of DNA sequences between two loxP sites [78]. The loxP site is a 34 bp sequences consisting of a two 13 bp inverted and palindromic repeats and 8 bp core sequences (Figure 1A).
Cre-loxP system is a widely used powerful technology for mammalian gene editing. This system has advantages which is very simple manipulation and do not require additional factors for efficient recombination [1]. Concerning the mechanism of Cre-loxP system, a single Cre recombinase recognizes two directly repeated loxP site, then the Cre excises the loxP flanked (floxed) DNA, thus creating two types of DNA with circular, excised and inactivated gene Y (Figure 1A). While the Cre-loxP system is predominantly used in genetic excision, it also induces the inversion and translocation of DNA between two loxP sites depending on the orientation and location of loxP sites [129].
To generate the spatiotemporally controlled mutant mice, two elements are needed in the Cre-loxP system [10111213]. First, Cre-driver strain is generated in which Cre recombinase is expressed by a promoter that specifically targets the cell or tissue of interest. Second, loxP flanked (floxed) DNA containing mouse strain is needed to be generated. Conditional knockout mice are then generated by breeding the Cre-driver strain with a floxed mouse strain (Figure 1B). The specificity and timing of recombination are controlled by used promoter and/or enhancer.
To achieve more accurate genetic functional studies and clinical applications using Cre-loxP system, it was required a more sophisticated technique that controls the Cre activation at the precise time and in a specific cell. An inducible Cre system is controlled by cell-specific regulatory elements (promoters and enhancers) and temporally inducible way by exogenous inducer such as tamoxifen (tam) or tetracycline (tet) [11014151617].
Tamoxifen-inducible Cre system is achieved by modified Cre protein fused with the estrogen receptor containing a mutated ligand binding domain (ER-LBD) [1415]. The fused Cre protein is called CreER recombinase, tamoxifen (also known as CreERT, Cre-ERT) and normally presented in the cytoplasm in a form that binds to heat shock protein 90 (HSP90). Upon binding the synthetic steroids (such tamoxifen or 4-hydoxytamoxifen; 4-OHT), the interaction is disrupted between HSP90 and CreERT. This causes nuclear translocation of CreERT and the interaction of Cre with loxP sites (Figure 2A). In this system, tamoxifen is systemically administered via intraperitoneal injection. To improve the function of targeted mutagenesis using CreERT, a new version of CreER, CreERT2 (Cre-ERT2), that is about ten-folds more sensitive to 4-OHT in vivo than CreERT has been generated [1518]. Thus, the use of CreERT2 is more preferred in several biological fields.
Another temporal and cell specific inducible system is tetracycline (Tet) system, also called doxycycline (Dox; a tetracycline derivative)-inducible Cre system. Dox is much more cost-effective, usable and efficient in controlling the Tet receptor (TetR) than tetracycline. This system is available in two modes, Tet-on and Tet-off, which permit Dox-dependent gene activation [1920] or inactivation [17]. The Tet systems consist of three elements, reverse tetracycline-controlled transactivator (rtTA), tetracycline-controlled transactivator (tTA) and tetracycline responsive element (TRE), also referred to as a tetracycline operon (TetO), which regulates a cre gene expression. When the rtTA binds to Dox, it can bind to the tetO7 (7 repeats of tetO) sequences and activates Cre gene expression (“Tet-on”) (Figure 2B), on the other hand, tTA binds to tetO7 sequences in normally, and when it is coupled with Dox, it can no longer bind to the tetO7 sequences and inactivates Cre expression (“Tet-off”) (Figure 2C). In Tet system, doxycycline is usually administered in feed or drinking water.
Due to the usefulness of the Cre-loxP system, numerous organ-specific Cre and/or inducible Cre transgenic mouse lines have been created and are still being developed. Here, we provide some most commonly used tissue-specific promoter/enhancer of Cre driver lines in Table 1. The various organs were divided into 6 physiological systems: nervous, integumentary, immune, musculoskeletal, digestive and urogenital system. The useful promoters of Cre are selected and summarized based on International Mouse Phenotyping Consortium (IMPC), Mouse Genome Informatics (MGI), The Jackson Laboratory (JAX Cre repository) and National Center for Biotechnology Information (NCBI) databases.
Nervous system consists of two main parts, the central nervous system (CNS), which includes the brain and spinal cord, and the peripheral nervous system (PNS), which includes the all nerves of the entire body. Since the brain is very anatomically and functionally divided, we will divide it into three arbitrary regions; cerebrum, cerebellum and brain stem.
The aldehyde dehydrogenase 1 family, member L1 (Aldh1L1) [21] and glial fibrillary acidic protein (Gfap) [2223] are expressed in astrocytes, but the GFAP is also known to be expressed in adult neural stem cells. Expression of calcium/calmodulin-dependent protein kinase II alpha (CaMK2α) [24] and glutamate receptor, ionotropic, kainate 4 (Grik4) [25] is detected in each CA1 and CA3 pyramidal neurons of hippocampus. Distal-less homeobox 1 (Dlx1) [26], distal-less homeobox 5/6 (Dlx5/6) [272829], glutamic acid decarboxylase 2 (Gad2), synthase 1, neuronal (nNos), parvalbumin (Pv, also called Pva, Parv) [30], somatostatin (Sst) and vasoactive intestinal polypeptide (Vip) promoters are predominantly expressed in inhibitory GABAergic interneurons [29], whereas the solute carrier family 17; sodium-dependent inorganic phosphate cotransporter, member 6 (Slc17a6) is expressed in excitatory glutamatergic, vesicular glutamate transport 2 (VGLUT2)-positive neurons [31]. The nestin (Nes) is also expressed in precursor cells of neuron and glia [32]. Platelet derived growth factor receptor, alpha polypeptide (Pdgfrα) is expressed in inner nuclear layer of the retina [33]. This promoter is useful in studies of retinal degenerative diseases such as age-related macular degeneration (AMD) and retinitis pigmentosa (RP). Expression of the leptin receptor (Lepr) is detected in hypothalamus and amygdala, and the promoter is used in research related to food intake and energy expenditure [34]. In the study of oligodendrocytes, proteolipid protein (myelin) 1 (PLP1) promoter is very useful in the studies of the gene function in myelination of mature oligodendrocytes [35]. Here, we provide the detailed expression regions of cerebral specific promoters in Table 2.
In the cerebellum, Purkinje cells are the most salient cell type and play a major role in the cerebellar circuit. Purkinje cell protein 2 (Pcp2) is expressed in most Purkinje cells and is very useful for cerebellar studies [36].
The brainstem is a region located between cerebrum and spinal cord and connects the two. Brainstem consists of three regions: midbrain, pons and medulla oblongata of the hindbrain. Solute carrier family 6; neurotransmitter transporter, dopamine, member 3 (Slc6a3 also known as DAT; dopamine transporter 1) is expressed in dopaminergic neurons of the midbrain and used for studying drug addiction and Parkinson's disease [37]. FEV; ETS oncogene family (Fev, also known as ePet) is expressed in the serotonin (5HT)-specific neurons of the midbrain, especially in the dorsal ad median raphe regions [38]. Neuropeptide Y receptor Y2 (Npy2r) expression is restricted in subsets of vagal sensory neurons in the lung and brainstem that regulate respiration [39]. In spinal cord, cadherin 3 (Cdh3) and 5-hydroxytryptamine; serotonin, receptor 6 (Htr6) are expressed in a subclass of inhibitory and excitatory interneurons in the dorsal horn of the spinal cord, respectively and these promoters have been used in neuronal functional studies [40].
Unlike the identification of enormous amounts of CNS-specific promoters of Cre, there are not many useful PNS-specific promoters. Actin-binding protein, Advillin (Avil)-driven Cre is expressed in all dorsal root ganglion (DRG) and trigeminal neurons but not in superior cervical ganglia (SCG) and is widely used for the studies of nociception in sensory neurons [4142434445].
The integumentary system consists of the skin and its appendages such as hair, nails, sebaceous glands and sweat glands. Skin has at least three layers: epidermis (outer layer), dermis (middle layer) and hypodermis (deeper fatty layer, known as subcutaneous layer).
Epidermis, the top layer of skin, has five layers: stratum corneum, stratum lucidum, stratum granulosum, stratum spinosum and stratum basale. Each layer is composed of keratinocytes in different states [46]. Keratinocytes is the main cell type (~90%) of the epidermis and produces keratin, an intermediate filaments protein. Keratin 5 (Krt5) and Keratin 14 (Krt14) is expressed in basal keratinocytes of stratum basale [1847]. Keratin 10 (Krt10) is expressed in post-mitotic suprabasal keratinocytes [48]. Keratin 18 (Krt 18) expression is observed in Merkel cells of the basal epidermal layer [49]. Leucine-rich repeat-containing G protein-coupled receptor 6 (Lgr6, also known as GPCR) is expressed in hair placodes at embryonic stage and in follicle bulge of dermis at adult stage [50].
Immune system is a host defense response against foreign invaders, and it protects the body from disease. This system consists of two working modes: innate immunity (also called non-specific, natural or native immunity), which is a first defense mechanism against invaders and initiates the adaptive immunity, and the adaptive immunity (also called specific or acquired immunity), which is systemic response mediating by lymphocytes such as T and B cells [515253]. The leukocytes, three major phagocytes (dendritic cells, macrophages and neutrophils), basophils, eosinophils, mast cells and natural killer cells (NK cells), are included in innate immunity and the helper T, cytotoxic T and B lymphocytes are included in adaptive immunity
Lysozyme 2 (Lyz2) is expressed in various myeloid cells including monocytes, macrophages and granulocytes. It is used for the studies of myeloid cell lineage tracing and the innate immunity [54].
Dendritic cells and mast cells are involved in innate immunity. To study the homeostasis and function of dendritic cells, Integrin alpha X (Itgax, also known as CD11c) promoter is used [55]. Chymase 1 (CMA1) is expressed in mast cells of tissues and can be used for the studying of their function or fate mapping [56].
T and B lymphocytes are representative immune cells involved in adaptive immunity. CD2 molecule (CD2) is expressed in peripheral T cells such as CD4 and CD8 cells [57]. The CD4 antigen (CD4), a membrane glycoprotein, is expressed in CD4-expressing T cells in lymphoid tissues and used for the studies of CD4-expressing T cell maturation and proliferation [5859]. Tumor necrosis factor receptor superfamily, member 4 (OX40, also known as ACT35, Txgp1, TXGP1L and CD134) is also expressed in activated CD4-positive T cells [60]. Expression of CD8 antigen, alpha chain (CD8a) is only observed in peripheral CD8-positive T cells but not CD4-T cells [61]. Forkhead box P3 (Foxp3) is used for studying the lineage stability of regulatory T cells (Treg) [62]. Lymphocyte protein tyrosine kinase (Lck) is mainly expressed in T cells and used for the selection and maturation studies of T cell development in thymus [6364]. CD19 antigen (CD19) is known to B-lymphocyte surface antigen and is expressed throughout B-lymphocyte development [6566].
The musculoskeletal system (also known as the locomoter system) is composed of two systems, muscular and skeletal system. This organ system is made up of the bones (the skeleton), muscles, cartilage, tendon, ligaments, joints and other connective tissues that bind tissues and organs together.
The bone formation (also known as ossification) process is caused by two types of ossification: intramembranous ossification (including skull and clavicle), bone is developed directly from mesenchyme or fibrous connective tissue and endochondral ossification, bone is created from a pre-existing cartilage. A majority of the skeleton is formed by endochondral ossification. In the bone formation, three different types of cells are involved: Osteoblasts, Osteocytes and Osteoclasts.
The bone cells, Osteoblasts (immature, bone-forming cells) and Osteocytes (mature bone cells), are derived from osteochondro progenitor cells arose from mesenchymal stem cells (MSCs). In studying these cells, two twist basic helix-loop-helix transcription factor 2 (Twist2 also known as Dermo1) [7172] and paired related homeobox1, rat (Prrx1 also known as Prx1) [73]-containing promoters are used in osteochondro progenitor cells and mesenchymal cells. Also, for the bone growth and physiological studies, bone gamma-carboxyglutamate protein (BGLAP; OC, also known as HOC, OCN, Osteocalcin) [74], collagen, type I, alpha 1 (Col1a1) [75] and Sp7 transcription factor 7 (Sp7, also known as osterix, Osx1) [76] -promoters are used for osteoblasts and Dmp1 (dentin matrix protein 1) is used for osteocytes [77]. The osteoclasts, which is the bone-resorbing cells, are derived from hematopoietic progenitors. For studying the functional regulation of osteoclasts, cathepsin K (Ctsk) promoter is used [75787980].
The muscular system includes three different types of muscle tissue: skeletal (also called striated), smooth (non-striated) and cardiac muscles. Actin, alpha 1, skeletal muscle (ACTA1 also known as HAS) [84] expressed in striated muscles, myogenic factor 5 (Myf5) [85] and myogenic factor 6 (Myh6 also known as αMHC) expressed in differentiated myocytes [86] are used in studies of skeletal muscle. Creatine kinase, muscle (Ckmm also known as Mck) is expressed in both skeletal and cardiac muscles [87]. Transgelin (Tagln also known as SM22α; smooth muscle protein 22-alpha) is expressed in vascular smooth muscle cells of liver and lung and used in studies of vascular diseases [88].
This system includes digestive tract and various accessory organs to assist digestion by secreting enzymes. The digestive tract (also called gastrointestinal tract) consists of the mouth, esophagus, stomach, small intestine, large intestine and anus. The accessory organs consist of salivary glands, liver, pancreas and so on.
Expression of the ED-L2 promoter of the Epstein-Barr virus, (ED-L2) is reported in squamous epithelial of the tongue, esophagus and forestomach in previous studies [899091]. To study the gastric cancer and diseases of the stomach, the ATPase, H+/K+ exchanging, beta polypeptide (Atp4b) [92], H+,K+-ATPase b-subunit (HK) [93] and trefoil factor 1 (Tff1, also known as PS2 or Bcei) promoters have been used. Atp4b and HK are expressed in progenitors of parietal cells and parietal cells secreting hydrochloric acid (HCl) and intrinsic factor, respectively. Tff1 is expressed in the epithelium of the glandular stomach, a source of secreting gastric enzymes and acid for digestion [94].
The carbonic anhydrase 1 (Car1) is expressed in the large intestinal tissues, including the cecum and colon [95]. This promoter is useful in colon disease research. Villin 1 (Vil1) promoter is most widely used in intestine studies and is expressed in epithelial cells of the small and large intestines from embryonic day 12.5 to adulthood [96].
Achaete-scute family bHLH transcription factor 3 (Ascl3 also known as Sgn1) promoter is expressed in progenitors of both secretory acinar and ductal cells, and thus it is used in developmental studies of salivary gland [97]. Albumin (Alb) promoter is known to exclusively express the Cre recombinase in the liver and are the most commonly used in liver-specific genetic studies [9899].
Pancreatic cells are divided two compartments: alpha, beta, delta, epsilon and PP (pancreatic polypeptide) cells are involved in endocrine function and acinal cells and epithelial cells lining ducts are involved in exocrine function. So far, various pancreatic cell-specific promoters have been identified and used. Glucagon (Gcg, also known as Glu) [100101], ghrelin (Ghrl) [102] and pancreatic polypeptide (Ppy, also known as PP) [101] are used for alpha, epsilon and PP cells, respectively. Insulin I (Ins1, also known as MIP) [103] and insulin 2 (Ins2, also known as RIP) [104] are available in beta cell specific studies. Pancreas specific transcription factor, 1a (Ptf1a) [105] and SRY (sex determining region Y)-box 9 (SOX9) [106] are useful in pancreatic acinal and ductal cell studies, respectively.
The Urogenital system is composed of urinary system including bladder and kidney and reproductive organs including ovary and testis.
Uroplakin 2 (Upk2) is expressed in urothelium of the bladder and used for the bladder cancer and urothelium functional studies [107]. The promoters for the kidney study are as follows. Aquaporin 2 (Aqp2) is expressed in collecting duct of the kidney and used in nephrological studies [108]. Forkhead box D1 (Foxd1) is detected in metanephric mesenchyme and is useful in studies of pericyte differentiation and fibrotic disease of kidney [109]. The expression of glial cell line derived neurotrophic factor (Gdnf) is restricted in renal progenitor cells and this promoter is useful in kidney developmental studies [110]. Gamma-glutamyltransferase (Ggt1) is expressed in the cortical tubular epithelium of the kidney and used for studying polycystic kidney disease and renal fibrosis [111]. Kidney androgen regulated protein (Kap) is expressed in renal proximal tubule of male kidney [112]. Nephrosis 2 (Nphs2, also known as podocin) is exclusively expressed in podocytes within the kidney glomeruli [113].
Growth differentiation factor 9 (Gdf9) is expressed in oocytes of the primordial follicles and used in folliculogenesis and oocyte developmental studies [114]. Expression of the zona pellucida glycoprotein 3 (Zp3) is detected in female germ line, and thus this promoter is useful for generating the deletion of the desired gene in the germ line [114115116]. Expression of the anti-Mullerian hormone (Amh) [117] is restricted in Sertoli cells and used in studies of the male embryonic sexual differentiation and spermatogenesis. Heat shock protein 2 (Hspa2) is expressed in spermatocytes and spermatids within the testis and this promoter is useful in assessing the gene functional studies during and after meiotic prophase in pachytene spermatocytes [118].
To date, a huge numerous Cre transgenic mouse lines have been established and being generated continuously. Here we introduce a free, publicly accessible web site that can be used to provide more accurate and versatile information on the Cre mouse lines required by the researchers in Table 3.
Unfortunately, there is still no worldwide database site where researchers can find all the information about the desired Cre mouse lines in one place. The scientific community makes a constant effort for efficiency in collecting and sharing this information. Coordination of resources for conditional expression of mutated mouse alleles (CREATE) consortium web site (http://creline.org/) had integrated and provided information on Cre mouse lines from four international partner databases: Cre portal at MGI (http://www.creportal.org), Cre-X-Mice (http://nagy.mshri.on.ca/cre_new/index.php), CreZoo (http://bioit.fleming.gr/crezoo) and CreERT2Zoo (http://www.ics-mci.fr/crezoo.html) [119120121122123124125126]. But now information of CREATE has integrated into Cre portal at International Mouse Phenotyping Consortium (IMPC) (https://www.mousephenotype.org/data/order/creline). Cre portal sites of MGI and CreZOO are available, but Cre-X-Mice and CreERT2Zoo has integrated into Mouse Resource Browse (MRB) (http://bioit.fleming.gr/mrb) and MouseCre (Mouse Cre and CreERT2 zoo) (http://mousecre.phenomin.fr/), respectively. The Jackson Laboratory's Cre Repository (http://www.Jax.org/research-and-faculty/resources/cre-repository), Neuroscience Blueprint Cre Driver Network of NIH (National Institutes of Health) (http://www.credrivermice.org/) and Gene Expression Nervous System Atlas (GENSAT) (http://www.gensat.org/cre.jsp) can also be used to find information about desired Cre mouse lines. In particular, GENSAT [21127] and Neuroscience Blueprint Cre Driver Network [29] provide useful information in neuroscience.
Technology using Cre-loxp system provides the sophistication to study the gene functions. To date, a tremendous amount of Cre-driver lines has been developed by researchers and are being shared through international networks. Recently, Cre-loxp system for more precise control has been continuously developed. Various types of Cre plasmids are being developed such as fluorescent Cre fused to fluorescent reporter, optimized Cre with high expression ratio and split Cre having the different promoters in N- and C-terminal regions. In addition, Cre-loxp system approaches has been continually being developed using Crispr/Cas9 technology and viral system. Using more sophisticated control techniques, researchers will be able to understand more precise gene functions by studying the function of specific genes at desired time (temporal) and tissue (spatial). The useful Cre-related portal sites and databases will enhance the efficiency of research by allowing researchers to find and obtain the suitable Cre-driver lines for research.
Figures and Tables
Figure 1
Mechanism of Cre-loxP system. (A) An overview of Cre-loxP system. 38 kDa Cre recombinase recognizes the loxP sites of specific 34 bp DNA sequences. (B) General breeding strategy for conditional mutation using loxP and Cre driving mouse line. In principle, one mouse must have a tissue-specific driven cre gene and another mouse have loxP flanked (floxed) alleles of interest gene Y. Expression of Cre recombinase excises floxed loci and inactivates the gene Y.
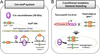
Figure 2
Principles of Inducible Cre-loxP mutation system. (A) Tamoxifen (Tam)-inducible System of estrogen receptor fused to Cre (CreER). In the absence of tamoxifen, expressed fusion protein, CreER, interacts with heat shock protein 90 (HSP90) and exists in cytoplasm (1). Administration of Tam disrupts the interaction of HSP90 with CreER (2). Interaction of ER with Tam induces nuclear translocation of Cre (3). In the nucleus, the CreER recognizes the loxP sites (4) and inactivates the gene Y in tissue X (5). (B and C) Doxycycline (Dox)-induced Tetracyclin (Tet)-on and -off systems. (B) In Tet-on system, ubiquitous or tissue-specific promoter driven rtTA is expressed. In the absence of Dox, inactivated rtTA is unable to bind tetO7 (7 repeats of a 19 nuclotide tetO minimal promoter, also referred to as TRE) sequence of cre gene. Cre is not expressed. Following Dox administration, Dox interacts with the rtTA and allows to activate. Activated rtTA binds to tetO7 promoter of cre and induces the Cre expression. (C) In Tet-off system, in the absence of Dox, activated tTA is able to bind tetO7 (TRE) sequence of cre and induces the Cre expression. Upon Dox administration, tTA interacted with Dox is inactivated. Inactivated rTA is not able to bind to tetO7 promoter and therefore Cre expression is inhibited.
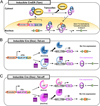
Table 1
Summary of the commonly used Cre promoters for studying specific tissues
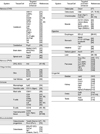
System | Tissue/Cell | Targeted promoter/enhancer | References |
---|---|---|---|
Nervous (CNS) | Aldh1l1 | [21] | |
Cerebrum | CaMIIα | [24] | |
Dlx1 | [26] | ||
Dlx5/6 | [272829] | ||
Gad2 | [30] | ||
GFAP | [2223] | ||
Grik4 | [25] | ||
Lepr | [34] | ||
Nes | [32] | ||
nNOS | [30] | ||
Pdgfrα | [33] | ||
PLP1 | [35] | ||
Pv (Pvalb) | [30] | ||
Slc17a6 | [31] | ||
Sst | [29] | ||
Vip | [29] | ||
Cerebellum | Pcp2 | [36] | |
Brain stem | Slc6a3 (DAT) | [37] | |
ePet (Fev) | [38] | ||
Npy2r | [39] | ||
Spinal cord | Cdh3 | [40] | |
Htr6 | [40] | ||
Nervous (PNS) | |||
DRG, SCG | Avil | [4142434445] | |
Integumentary | |||
Skin | Krt5 | [18] | |
Krt10 | [48] | ||
Krt14 | [47] | ||
Krt18 | [49] | ||
Hair follicles | Lgr6 | [50] | |
Immune | |||
Macrophage | Lyz2 | [54] | |
Dendritic cells | CD11c (Itgax) | [55] | |
Mast cells | CMA1 | [56] | |
T-cells | CD2 | [57] | |
CD4 | [5859] | ||
CD8a | [61] | ||
Foxp3 | [62] | ||
Lck | [6364] | ||
OX40 | [60] | ||
B-cells | CD19 | [6566] | |
Haematopoietic tissue | CD45 (Ly5, Ptprc) | [70] | |
Vav | [676869] | ||
Musculoskeletal | |||
Osteochondro progenitors | Twist2 (Dermo1) | [7172] | |
Prrx1 (Prx1) | [73] | ||
Osteoblasts | BGLAP (OC) | [74] | |
Col1a1 | [75] | ||
Sp7 (Osterix, Osx1) | [76] | ||
Osteocytes | Dmp1 | [77] | |
Osteoclasts | Ctsk | [75787980] | |
Chondrocytes | Acan (Agc1) | [81] | |
Col10a1 | [8283] | ||
Muscle | ACTA1 (HSA) | [84] | |
Ckmm (Mck) | [87] | ||
Myf5 | [85] | ||
Myh6 (αMHC) | [86] | ||
Tagln (SM22α) | [88] | ||
Digestive | |||
Esophagus | ED-L2 | [899091] | |
Stomach | Atp4b | [92] | |
H+,K+-ATPase | [93] | ||
b-subunit | [94] | ||
Tff1 | |||
Intestine | Car1 | [95] | |
Vil1 | [96] | ||
Salivary gland | Ascl3 | [97] | |
Liver | Alb | [9899] | |
Pancreas | Gcg (Glu) | [100101] | |
Ghrl | [102] | ||
Ins1 (MIP) | [103] | ||
Ins2 (RIP) | [104] | ||
Ppy (PP) | [101] | ||
Ptf1a | [105] | ||
SOX9 | [106] | ||
Urogenital | |||
Bladder | Upk2 | [107] | |
Kidney | Aqp2 | [108] | |
Foxd1 | [109] | ||
Gdnf | [110] | ||
Ggt1 | [111] | ||
Kap | [112] | ||
Nphs2 (Podocin) | [113] | ||
Ovary | Gdf9 | [114] | |
Zp3 | [114115116] | ||
Testis | Amh | [117] | |
Hspa2 | [118] |
Table 2
Expression regions of cerebral specific promoters
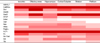
Isocortex | Olfactory areas | Hippocampus | Cortical Subplate | Striatum | Pallidum | |
---|---|---|---|---|---|---|
![]() |
*Table 2 is summarized by quoting data from ALLEN BRAIN ATLAS (http://mouse.brain-map.org/).
*Table 2 is based on the in situ hybridization data of the adult mouse brain (postnatal 56 day).
*Expression levels above the threshold (0.15 of raw expression value) are considered significant value.
*Raw expression values are expressed as arbitrary units with different colors; <0.15,
0.15~2.0,
2.0~4.0,
4.0~6.0,
6.0~8.0 and
8.0<
Table 3
Useful portal site related to databases of Cre driver line
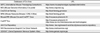
Databases of Cre lines | URL |
---|---|
IMPC (International Mouse Phenotyping Consortium) | https://www.mousephenotype.org/data/order/creline |
MGI (Mouse Genome Informatics) Cre portal | http://www.creportal.org |
CreZOO at Fleming | http://bioit.fleming.gr/crezoo |
MRB (Mouse Resource Browse / CRE-X-Mice) | http://bioit.fleming.gr/mrb |
MouseCRE (Mouse Cre and CreERT2 Zoo) | http://mousecre.phenomin.fr/ |
CreERT2Zoo | http://mousecre.phenomin.fr/ |
(http://www.ics-mci.fr/en/resources-and-technologies) | |
JAX Cre Repository | http://www.Jax.org/research-and-faculty/resources/cre-repository |
NIH Neuroscience blueprint Cre Driver Network | http://www.credrivermice.org/ |
GENSAT (Gene Expression Nervous System Atlas) | http://www.gensat.org/cre,jsp |
Acknowledgments
This research was supported by Basic Science Research Program through the National Research Foundation of Korea (NRF-2018R1A2B6003447) and faculty research grant of Yonsei University College of Medicine (6-2017-0099).
References
1. Nagy A. Cre recombinase: the universal reagent for genome tailoring. Genesis. 2000; 26(2):99–109.


2. Meinke G, Bohm A, Hauber J, Pisabarro MT, Buchholz F. Cre Recombinase and Other Tyrosine Recombinases. Chem Rev. 2016; 116(20):12785–12820.


3. Sternberg N, Austin S, Hamilton D, Yarmolinsky M. Analysis of bacteriophage P1 immunity by using lambda-P1 recombinants constructed in vitro. Proc Natl Acad Sci USA. 1978; 75(11):5594–5598.


4. Sternberg N, Hamilton D. Bacteriophage P1 site-specific recombination. I. Recombination between loxP sites. J Mol Biol. 1981; 150(4):467–486.
5. Sternberg N, Sauer B, Hoess R, Abremski K. Bacteriophage P1 cre gene and its regulatory region. Evidence for multiple promoters and for regulation by DNA methylation. J Mol Biol. 1986; 187(2):197–212.
6. Sauer B. Inducible gene targeting in mice using the Cre/lox system. Methods. 1998; 14(4):381–392.
7. O'Neil KT, Hoess RH, DeGrado WF. Design of DNA-binding peptides based on the leucine zipper motif. Science. 1990; 249(4970):774–778.
8. Sauer B, Henderson N. Site-specific DNA recombination in mammalian cells by the Cre recombinase of bacteriophage P1. Proc Natl Acad Sci USA. 1988; 85(14):5166–5170.


9. Branda CS, Dymecki SM. Talking about a revolution: The impact of site-specific recombinases on genetic analyses in mice. Dev Cell. 2004; 6(1):7–28.
10. McLellan MA, Rosenthal NA, Pinto AR. Cre-loxP-Mediated Recombination: General Principles and Experimental Considerations. Curr Protoc Mouse Biol. 2017; 7(1):1–12.


11. Gu H, Zou YR, Rajewsky K. Independent control of immunoglobulin switch recombination at individual switch regions evidenced through Cre-loxP-mediated gene targeting. Cell. 1993; 73(6):1155–1164.


12. Gu H, Marth JD, Orban PC, Mossmann H, Rajewsky K. Deletion of a DNA polymerase beta gene segment in T cells using cell type-specific gene targeting. Science. 1994; 265(5168):103–106.


13. Rajewsky K, Gu H, Kühn R, Betz UA, Müller W, Roes J, Schwenk F. Conditional gene targeting. J Clin Invest. 1996; 98(3):600–603.


14. Metzger D, Chambon P. Site- and time-specific gene targeting in the mouse. Methods. 2001; 24(1):71–80.


15. Brocard J, Warot X, Wendling O, Messaddeq N, Vonesch JL, Chambon P, Metzger D. Spatio-temporally controlled site-specific somatic mutagenesis in the mouse. Proc Natl Acad Sci USA. 1997; 94(26):14559–14563.


16. Kühn R, Schwenk F, Aguet M, Rajewsky K. Inducible gene targeting in mice. Science. 1995; 269(5229):1427–1429.


17. Gossen M, Bujard H. Tight control of gene expression in mammalian cells by tetracycline-responsive promoters. Proc Natl Acad Sci USA. 1992; 89(12):5547–5551.


18. Indra AK, Warot X, Brocard J, Bornert JM, Xiao JH, Chambon P, Metzger D. Temporally-controlled site-specific mutagenesis in the basal layer of the epidermis: comparison of the recombinase activity of the tamoxifen-inducible Cre-ER(T) and Cre-ER(T2) recombinases. Nucleic Acids Res. 1999; 27(22):4324–4327.


19. Gossen M, Freundlieb S, Bender G, Müller G, Hillen W, Bujard H. Transcriptional activation by tetracyclines in mammalian cells. Science. 1995; 268(5218):1766–1769.


20. Kistner A, Gossen M, Zimmermann F, Jerecic J, Ullmer C, Lübbert H, Bujard H. Doxycycline-mediated quantitative and tissue-specific control of gene expression in transgenic mice. Proc Natl Acad Sci USA. 1996; 93(20):10933–10938.


21. Gong S, Doughty M, Harbaugh CR, Cummins A, Hatten ME, Heintz N, Gerfen CR. Targeting Cre recombinase to specific neuron populations with bacterial artificial chromosome constructs. J Neurosci. 2007; 27(37):9817–9823.


22. Garcia AD, Doan NB, Imura T, Bush TG, Sofroniew MV. GFAPexpressing progenitors are the principal source of constitutive neurogenesis in adult mouse forebrain. Nat Neurosci. 2004; 7(11):1233–1241.


23. Brenner M, Kisseberth WC, Su Y, Besnard F, Messing A. GFAP promoter directs astrocyte-specific expression in transgenic mice. J Neurosci. 1994; 14(3 Pt 1):1030–1037.


24. Dragatsis I, Zeitlin S. CaMKIIalpha-Cre transgene expression and recombination patterns in the mouse brain. Genesis. 2000; 26(2):133–135.
25. Nakazawa K, Quirk MC, Chitwood RA, Watanabe M, Yeckel MF, Sun LD, Kato A, Carr CA, Johnston D, Wilson MA, Tonegawa S. Requirement for hippocampal CA3 NMDA receptors in associative memory recall. Science. 2002; 297(5579):211–218.


26. Potter GB, Petryniak MA, Shevchenko E, McKinsey GL, Ekker M, Rubenstein JL. Generation of Cre-transgenic mice using Dlx1/ Dlx2 enhancers and their characterization in GABAergic interneurons. Mol Cell Neurosci. 2009; 40(2):167–186.
27. Zerucha T, Stühmer T, Hatch G, Park BK, Long Q, Yu G, Gambarotta A, Schultz JR, Rubenstein JL, Ekker M. A highly conserved enhancer in the Dlx5/Dlx6 intergenic region is the site of cross-regulatory interactions between Dlx genes in the embryonic forebrain. J Neurosci. 2000; 20(2):709–721.
28. Monory K, Massa F, Egertová M, Eder M, Blaudzun H, Westenbroek R, Kelsch W, Jacob W, Marsch R, Ekker M, Long J, Rubenstein JL, Goebbels S, Nave KA, During M, Klugmann M, Wölfel B, Dodt HU, Zieglgänsberger W, Wotjak CT, Mackie K, Elphick MR, Marsicano G, Lutz B. The endocannabinoid system controls key epileptogenic circuits in the hippocampus. Neuron. 2006; 51(4):455–466.


29. Taniguchi H, He M, Wu P, Kim S, Paik R, Sugino K, Kvitsiani D, Fu Y, Lu J, Lin Y, Miyoshi G, Shima Y, Fishell G, Nelson SB, Huang ZJ. A resource of Cre driver lines for genetic targeting of GABAergic neurons in cerebral cortex. Neuron. 2011; 71(6):995–1013.


30. Hippenmeyer S, Vrieseling E, Sigrist M, Portmann T, Laengle C, Ladle DR, Arber S. A developmental switch in the response of DRG neurons to ETS transcription factor signaling. PLoS Biol. 2005; 3(5):e159.


31. Vong L, Ye C, Yang Z, Choi B, Chua S Jr, Lowell BB. Leptin action on GABAergic neurons prevents obesity and reduces inhibitory tone to POMC neurons. Neuron. 2011; 71(1):142–154.


32. Tronche F, Kellendonk C, Kretz O, Gass P, Anlag K, Orban PC, Bock R, Klein R, Schütz G. Disruption of the glucocorticoid receptor gene in the nervous system results in reduced anxiety. Nat Genet. 1999; 23(1):99–103.


33. Roesch K, Jadhav AP, Trimarchi JM, Stadler MB, Roska B, Sun BB, Cepko CL. The transcriptome of retinal Müller glial cells. J Comp Neurol. 2008; 509(2):225–238.


34. DeFalco J, Tomishima M, Liu H, Zhao C, Cai X, Marth JD, Enquist L, Friedman JM. Virus-assisted mapping of neural inputs to a feeding center in the hypothalamus. Science. 2001; 291(5513):2608–2613.


35. Doerflinger NH, Macklin WB, Popko B. Inducible site-specific recombination in myelinating cells. Genesis. 2003; 35(1):63–72.


36. Zhang XM, Ng AH, Tanner JA, Wu WT, Copeland NG, Jenkins NA, Huang JD. Highly restricted expression of Cre recombinase in cerebellar Purkinje cells. Genesis. 2004; 40(1):45–51.


37. Zhuang X, Masson J, Gingrich JA, Rayport S, Hen R. Targeted gene expression in dopamine and serotonin neurons of the mouse brain. J Neurosci Methods. 2005; 143(1):27–32.


38. Scott MM, Wylie CJ, Lerch JK, Murphy R, Lobur K, Herlitze S, Jiang W, Conlon RA, Strowbridge BW, Deneris ES. A genetic approach to access serotonin neurons for in vivo and in vitro studies. Proc Natl Acad Sci USA. 2005; 102(45):16472–16477.


39. Chang RB, Strochlic DE, Williams EK, Umans BD, Liberles SD. Vagal Sensory Neuron Subtypes that Differentially Control Breathing. Cell. 2015; 161(3):622–633.


40. Abraira VE, Kuehn ED, Chirila AM, Springel MW, Toliver AA, Zimmerman AL, Orefice LL, Boyle KA, Bai L, Song BJ, Bashista KA, O'Neill TG, Zhuo J, Tsan C, Hoynoski J, Rutlin M, Kus L, Niederkofler V, Watanabe M, Dymecki SM, Nelson SB, Heintz N, Hughes DI, Ginty DD. The Cellular and Synaptic Architecture of the Mechanosensory Dorsal Horn. Cell. 2017; 168(1-2):295–310.


41. Hasegawa H, Abbott S, Han BX, Qi Y, Wang F. Analyzing somatosensory axon projections with the sensory neuron-specific Advillin gene. J Neurosci. 2007; 27(52):14404–14414.


42. Lau J, Minett MS, Zhao J, Dennehy U, Wang F, Wood JN, Bogdanov YD. Temporal control of gene deletion in sensory ganglia using a tamoxifen-inducible Advillin-Cre-ERT2 recombinase mouse. Mol Pain. 2011; 7:100.


43. Lewis AE, Vasudevan HN, O'Neill AK, Soriano P, Bush JO. The widely used Wnt1-Cre transgene causes developmental phenotypes by ectopic activation of Wnt signaling. Dev Biol. 2013; 379(2):229–234.


44. Minett MS, Nassar MA, Clark AK, Passmore G, Dickenson AH, Wang F, Malcangio M, Wood JN. Distinct Nav1.7-dependent pain sensations require different sets of sensory and sympathetic neurons. Nat Commun. 2012; 3(1):791.


45. Zhou X, Wang L, Hasegawa H, Amin P, Han BX, Kaneko S, He Y, Wang F. Deletion of PIK3C3/Vps34 in sensory neurons causes rapid neurodegeneration by disrupting the endosomal but not the autophagic pathway. Proc Natl Acad Sci USA. 2010; 107(20):9424–9429.


46. Simpson CL, Patel DM, Green KJ. Deconstructing the skin: cytoarchitectural determinants of epidermal morphogenesis. Nat Rev Mol Cell Biol. 2011; 12(9):565–580.


47. Dassule HR, Lewis P, Bei M, Maas R, McMahon AP. Sonic hedgehog regulates growth and morphogenesis of the tooth. Development. 2000; 127(22):4775–4785.


48. Calléja C, Messaddeq N, Chapellier B, Yang H, Krezel W, Li M, Metzger D, Mascrez B, Ohta K, Kagechika H, Endo Y, Mark M, Ghyselinck NB, Chambon P. Genetic and pharmacological evidence that a retinoic acid cannot be the RXR-activating ligand in mouse epidermis keratinocytes. Genes Dev. 2006; 20(11):1525–1538.
49. Van Keymeulen A, Mascre G, Youseff KK, Harel I, Michaux C, De Geest N, Szpalski C, Achouri Y, Bloch W, Hassan BA, Blanpain C. Epidermal progenitors give rise to Merkel cells during embryonic development and adult homeostasis. J Cell Biol. 2009; 187(1):91–100.


50. Snippert HJ, Haegebarth A, Kasper M, Jaks V, van Es JH, Barker N, van de Wetering M, van den Born M, Begthel H, Vries RG, Stange DE, Toftgård R, Clevers H. Lgr6 marks stem cells in the hair follicle that generate all cell lineages of the skin. Science. 2010; 327(5971):1385–1389.


51. Gasteiger G, Rudensky AY. Interactions between innate and adaptive lymphocytes. Nat Rev Immunol. 2014; 14(9):631–639.


54. Clausen BE, Burkhardt C, Reith W, Renkawitz R, Förster I. Conditional gene targeting in macrophages and granulocytes using LysMcre mice. Transgenic Res. 1999; 8(4):265–277.
55. Caton ML, Smith-Raska MR, Reizis B. Notch-RBP-J signaling controls the homeostasis of CD8- dendritic cells in the spleen. J Exp Med. 2007; 204(7):1653–1664.


56. Müsch W, Wege AK, Männel DN, Hehlgans T. Generation and characterization of alpha-chymase-Cre transgenic mice. Genesis. 2008; 46(3):163–166.


57. Vacchio MS, Wang L, Bouladoux N, Carpenter AC, Xiong Y, Williams LC, Wohlfert E, Song KD, Belkaid Y, Love PE, Bosselut R. A ThPOK-LRF transcriptional node maintains the integrity and effector potential of post-thymic CD4+ T cells. Nat Immunol. 2014; 15(10):947–956.


58. Sawada S, Scarborough JD, Killeen N, Littman DR. A lineagespecific transcriptional silencer regulates CD4 gene expression during T lymphocyte development. Cell. 1994; 77(6):917–929.


59. Lee PP, Fitzpatrick DR, Beard C, Jessup HK, Lehar S, Makar KW, Pérez-Melgosa M, Sweetser MT, Schlissel MS, Nguyen S, Cherry SR, Tsai JH, Tucker SM, Weaver WM, Kelso A, Jaenisch R, Wilson CB. A critical role for Dnmt1 and DNA methylation in T cell development, function, and survival. Immunity. 2001; 15(5):763–774.


60. Klinger M, Kim JK, Chmura SA, Barczak A, Erle DJ, Killeen N. Thymic OX40 expression discriminates cells undergoing strong responses to selection ligands. J Immunol. 2009; 182(8):4581–4589.


61. Maekawa Y, Minato Y, Ishifune C, Kurihara T, Kitamura A, Kojima H, Yagita H, Sakata-Yanagimoto M, Saito T, Taniuchi I, Chiba S, Sone S, Yasutomo K. Notch2 integrates signaling by the transcription factors RBP-J and CREB1 to promote T cell cytotoxicity. Nat Immunol. 2008; 9(10):1140–1147.


62. Rubtsov YP, Niec RE, Josefowicz S, Li L, Darce J, Mathis D, Benoist C, Rudensky AY. Stability of the regulatory T cell lineage in vivo. Science. 2010; 329(5999):1667–1671.


63. Hennet T, Hagen FK, Tabak LA, Marth JD. T-cell-specific deletion of a polypeptide N-acetylgalactosaminyl-transferase gene by site-directed recombination. Proc Natl Acad Sci USA. 1995; 92(26):12070–12074.


64. Wang Q, Strong J, Killeen N. Homeostatic competition among T cells revealed by conditional inactivation of the mouse Cd4 gene. J Exp Med. 2001; 194(12):1721–1730.


65. Rickert RC, Roes J, Rajewsky K. B lymphocyte-specific, Cremediated mutagenesis in mice. Nucleic Acids Res. 1997; 25(6):1317–1318.


66. Yasuda T, Wirtz T, Zhang B, Wunderlich T, Schmidt-Supprian M, Sommermann T, Rajewsky K. Studying Epstein-Barr virus pathologies and immune surveillance by reconstructing EBV infection in mice. Cold Spring Harb Symp Quant Biol. 2013; 78:259–263.


67. Ogilvy S, Elefanty AG, Visvader J, Bath ML, Harris AW, Adams JM. Transcriptional regulation of vav, a gene expressed throughout the hematopoietic compartment. Blood. 1998; 91(2):419–430.


68. Ogilvy S, Metcalf D, Gibson L, Bath ML, Harris AW, Adams JM. Promoter elements of vav drive transgene expression in vivo throughout the hematopoietic compartment. Blood. 1999; 94(6):1855–1863.


69. Shimshek DR, Kim J, Hübner MR, Spergel DJ, Buchholz F, Casanova E, Stewart AF, Seeburg PH, Sprengel R. Codonimproved Cre recombinase (iCre) expression in the mouse. Genesis. 2002; 32(1):19–26.


70. Yang J, Hills D, Taylor E, Pfeffer K, Ure J, Medvinsky A. Transgenic tools for analysis of the haematopoietic system: knock-in CD45 reporter and deletor mice. J Immunol Methods. 2008; 337(2):81–87.


71. Šošiæ D, Richardson JA, Yu K, Ornitz DM, Olson EN. Twist regulates cytokine gene expression through a negative feedback loop that represses NF-kappaB activity. Cell. 2003; 112(2):169–180.
72. Yu K, Xu J, Liu Z, Sosic D, Shao J, Olson EN, Towler DA, Ornitz DM. Conditional inactivation of FGF receptor 2 reveals an essential role for FGF signaling in the regulation of osteoblast function and bone growth. Development. 2003; 130(13):3063–3074.


73. Logan M, Martin JF, Nagy A, Lobe C, Olson EN, Tabin CJ. Expression of Cre Recombinase in the developing mouse limb bud driven by a Prxl enhancer. Genesis. 2002; 33(2):77–80.
74. Zhang M, Xuan S, Bouxsein ML, von Stechow D, Akeno N, Faugere MC, Malluche H, Zhao G, Rosen CJ, Efstratiadis A, Clemens TL. Osteoblast-specific knockout of the insulin-like growth factor (IGF) receptor gene reveals an essential role of IGF signaling in bone matrix mineralization. J Biol Chem. 2002; 277(46):44005–44012.


75. Kim JE, Nakashima K, de Crombrugghe B. Transgenic mice expressing a ligand-inducible cre recombinase in osteoblasts and odontoblasts: a new tool to examine physiology and disease of postnatal bone and tooth. Am J Pathol. 2004; 165(6):1875–1882.
76. Rodda SJ, McMahon AP. Distinct roles for Hedgehog and canonical Wnt signaling in specification, differentiation and maintenance of osteoblast progenitors. Development. 2006; 133(16):3231–3244.
77. Lu Y, Xie Y, Zhang S, Dusevich V, Bonewald LF, Feng JQ. DMP1-targeted Cre expression in odontoblasts and osteocytes. J Dent Res. 2007; 86(4):320–325.


78. Baek WY, de Crombrugghe B, Kim JE. Postnatally induced inactivation of Osterix in osteoblasts results in the reduction of bone formation and maintenance. Bone. 2010; 46(4):920–928.


79. Sanchez-Fernandez MA, Sbacchi S, Correa-Tapia M, Naumann R, Klemm J, Chambon P, Al-Robaiy S, Blessing M, Hoflack B. Transgenic mice for a tamoxifen-induced, conditional expression of the Cre recombinase in osteoclasts. PLoS One. 2012; 7(5):e37592.


80. Tonna S, Takyar FM, Vrahnas C, Crimeen-Irwin B, Ho PW, Poulton IJ, Brennan HJ, McGregor NE, Allan EH, Nguyen H, Forwood MR, Tatarczuch L, Mackie EJ, Martin TJ, Sims NA. EphrinB2 signaling in osteoblasts promotes bone mineralization by preventing apoptosis. FASEB J. 2014; 28(10):4482–4496.


81. Henry SP, Jang CW, Deng JM, Zhang Z, Behringer RR, de Crombrugghe B. Generation of aggrecan-CreERT2 knockin mice for inducible Cre activity in adult cartilage. Genesis. 2009; 47(12):805–814.


82. Gebhard S, Hattori T, Bauer E, Schlund B, Bösl MR, de Crombrugghe B, von der Mark K. Specific expression of Cre recombinase in hypertrophic cartilage under the control of a BACCol10a1 promoter. Matrix Biol. 2008; 27(8):693–699.


83. Maye P, Fu Y, Butler DL, Chokalingam K, Liu Y, Floret J, Stover ML, Wenstrup R, Jiang X, Gooch C, Rowe D. Generation and characterization of Col10a1-mcherry reporter mice. Genesis. 2011; 49(5):410–418.


84. Miniou P, Tiziano D, Frugier T, Roblot N, Le Meur M, Melki J. Gene targeting restricted to mouse striated muscle lineage. Nucleic Acids Res. 1999; 27(19):e27.


85. Tallquist MD, Weismann KE, Hellström M, Soriano P. Early myotome specification regulates PDGFA expression and axial skeleton development. Development. 2000; 127(23):5059–5070.


86. Keller C, Arenkiel BR, Coffin CM, El-Bardeesy N, DePinho RA, Capecchi MR. Alveolar rhabdomyosarcomas in conditional Pax3:Fkhr mice: cooperativity of Ink4a/ARF and Trp53 loss of function. Genes Dev. 2004; 18(21):2614–2626.


87. Brüning JC, Michael MD, Winnay JN, Hayashi T, Hörsch D, Accili D, Goodyear LJ, Kahn CR. A muscle-specific insulin receptor knockout exhibits features of the metabolic syndrome of NIDDM without altering glucose tolerance. Mol Cell. 1998; 2(5):559–569.


88. Boucher P, Gotthardt M, Li WP, Anderson RG, Herz J. LRP: role in vascular wall integrity and protection from atherosclerosis. Science. 2003; 300(5617):329–332.


89. Nakagawa H, Wang TC, Zukerberg L, Odze R, Togawa K, May GH, Wilson J, Rustgi AK. The targeting of the cyclin D1 oncogene by an Epstein-Barr virus promoter in transgenic mice causes dysplasia in the tongue, esophagus and forestomach. Oncogene. 1997; 14(10):1185–1190.


90. Andl CD, Mizushima T, Nakagawa H, Oyama K, Harada H, Chruma K, Herlyn M, Rustgi AK. Epidermal growth factor receptor mediates increased cell proliferation, migration, and aggregation in esophageal keratinocytes in vitro and in vivo. J Biol Chem. 2003; 278(3):1824–1830.


91. Tetreault MP, Yang Y, Travis J, Yu QC, Klein-Szanto A, Tobias JW, Katz JP. Esophageal squamous cell dysplasia and delayed differentiation with deletion of krüppel-like factor 4 in murine esophagus. Gastroenterology. 2010; 139(1):171–181.


92. Syder AJ, Karam SM, Mills JC, Ippolito JE, Ansari HR, Farook V, Gordon JI. A transgenic mouse model of metastatic carcinoma involving transdifferentiation of a gastric epithelial lineage progenitor to a neuroendocrine phenotype. Proc Natl Acad Sci USA. 2004; 101(13):4471–4476.


93. Engevik AC, Feng R, Yang L, Zavros Y. The acid-secreting parietal cell as an endocrine source of Sonic Hedgehog during gastric repair. Endocrinology. 2013; 154(12):4627–4639.


94. Thiem S, Eissmann MF, Stuart E, Elzer J, Jonas A, Buchert M, Ernst M. Inducible gene modification in the gastric epithelium of Tff1-CreERT2, Tff2-rtTA, Tff3-luc mice. Genesis. 2016; 54(12):626–635.
95. Xue Y, Johnson R, Desmet M, Snyder PW, Fleet JC. Generation of a transgenic mouse for colorectal cancer research with intestinal cre expression limited to the large intestine. Mol Cancer Res. 2010; 8(8):1095–1104.


96. Madison BB, Dunbar L, Qiao XT, Braunstein K, Braunstein E, Gumucio DL. Cis elements of the villin gene control expression in restricted domains of the vertical (crypt) and horizontal (duodenum, cecum) axes of the intestine. J Biol Chem. 2002; 277(36):33275–33283.


97. Bullard T, Koek L, Roztocil E, Kingsley PD, Mirels L, Ovitt CE. Ascl3 expression marks a progenitor population of both acinar and ductal cells in mouse salivary glands. Dev Biol. 2008; 320(1):72–78.


98. Yakar S, Liu JL, Stannard B, Butler A, Accili D, Sauer B, LeRoith D. Normal growth and development in the absence of hepatic insulin-like growth factor I. Proc Natl Acad Sci USA. 1999; 96(13):7324–7329.


99. Stratikopoulos E, Szabolcs M, Dragatsis I, Klinakis A, Efstratiadis A. The hormonal action of IGF1 in postnatal mouse growth. Proc Natl Acad Sci USA. 2008; 105(49):19378–19383.


100. Shiota C, Prasadan K, Guo P, Fusco J, Xiao X, Gittes GK. GcgCreERT2 knockin mice as a tool for genetic manipulation in pancreatic alpha cells. Diabetologia. 2017; 60(12):2399–2408.
101. Herrera PL, Orci L, Vassalli JD. Two transgenic approaches to define the cell lineages in endocrine pancreas development. Mol Cell Endocrinol. 1998; 140(1-2):45–50.


102. Arnes L, Hill JT, Gross S, Magnuson MA, Sussel L. Ghrelin expression in the mouse pancreas defines a unique multipotent progenitor population. PLoS One. 2012; 7(12):e52026.


103. Thorens B, Tarussio D, Maestro MA, Rovira M, Heikkilä E, Ferrer J. Ins1(Cre) knock-in mice for beta cell-specific gene recombination. Diabetologia. 2015; 58(3):558–565.
104. Postic C, Shiota M, Niswender KD, Jetton TL, Chen Y, Moates JM, Shelton KD, Lindner J, Cherrington AD, Magnuson MA. Dual roles for glucokinase in glucose homeostasis as determined by liver and pancreatic beta cell-specific gene knock-outs using Cre recombinase. J Biol Chem. 1999; 274(1):305–315.
105. Kopinke D, Brailsford M, Pan FC, Magnuson MA, Wright CV, Murtaugh LC. Ongoing Notch signaling maintains phenotypic fidelity in the adult exocrine pancreas. Dev Biol. 2012; 362(1):57–64.


106. Kopp JL, Dubois CL, Schaffer AE, Hao E, Shih HP, Seymour PA, Ma J, Sander M. Sox9+ ductal cells are multipotent progenitors throughout development but do not produce new endocrine cells in the normal or injured adult pancreas. Development. 2011; 138(4):653–665.


107. Ayala de la Peña F, Kanasaki K, Kanasaki M, Tangirala N, Maeda G, Kalluri R. Loss of p53 and acquisition of angiogenic microRNA profile are insufficient to facilitate progression of bladder urothelial carcinoma in situ to invasive carcinoma. J Biol Chem. 2011; 286(23):20778–20787.


108. Ge Y, Ahn D, Stricklett PK, Hughes AK, Yanagisawa M, Verbalis JG, Kohan DE. Collecting duct-specific knockout of endothelin-1 alters vasopressin regulation of urine osmolality. Am J Physiol Renal Physiol. 2005; 288(5):F912–F920.


109. Humphreys BD, Lin SL, Kobayashi A, Hudson TE, Nowlin BT, Bonventre JV, Valerius MT, McMahon AP, Duffield JS. Fate tracing reveals the pericyte and not epithelial origin of myofibroblasts in kidney fibrosis. Am J Pathol. 2010; 176(1):85–97.


110. Cebrian C, Asai N, D'Agati V, Costantini F. The number of fetal nephron progenitor cells limits ureteric branching and adult nephron endowment. Cell Rep. 2014; 7(1):127–137.


111. Iwano M, Plieth D, Danoff TM, Xue C, Okada H, Neilson EG. Evidence that fibroblasts derive from epithelium during tissue fibrosis. J Clin Invest. 2002; 110(3):341–350.


112. Li H, Zhou X, Davis DR, Xu D, Sigmund CD. An androgeninducible proximal tubule-specific Cre recombinase transgenic model. Am J Physiol Renal Physiol. 2008; 294(6):F1481–F1486.


113. Moeller MJ, Sanden SK, Soofi A, Wiggins RC, Holzman LB. Podocyte-specific expression of cre recombinase in transgenic mice. Genesis. 2003; 35(1):39–42.


114. Lan ZJ, Xu X, Cooney AJ. Differential oocyte-specific expression of Cre recombinase activity in GDF-9-iCre, Zp3cre, and Msx2Cre transgenic mice. Biol Reprod. 2004; 71(5):1469–1474.
115. Lewandoski M, Wassarman KM, Martin GR. Zp3-cre, a transgenic mouse line for the activation or inactivation of loxPflanked target genes specifically in the female germ line. Curr Biol. 1997; 7(2):148–151.


116. de Vries WN, Binns LT, Fancher KS, Dean J, Moore R, Kemler R, Knowles BB. Expression of Cre recombinase in mouse oocytes: a means to study maternal effect genes. Genesis. 2000; 26(2):110–112.


117. Holdcraft RW, Braun RE. Androgen receptor function is required in Sertoli cells for the terminal differentiation of haploid spermatids. Development. 2004; 131(2):459–467.


118. Inselman AL, Nakamura N, Brown PR, Willis WD, Goulding EH, Eddy EM. Heat shock protein 2 promoter drives Cre expression in spermatocytes of transgenic mice. Genesis. 2010; 48(2):114–120.


119. Smedley D, Salimova E, Rosenthal N. Cre recombinase resources for conditional mouse mutagenesis. Methods. 2011; 53(4):411–416.


120. Chandras C, Zouberakis M, Salimova E, Smedley D, Rosenthal N, Aidinis V. CreZOO--the European virtual repository of Cre and other targeted conditional driver strains. Database (Oxford). 2012; 2012:bas029.


121. Murray SA, Eppig JT, Smedley D, Simpson EM, Rosenthal N. Beyond knockouts: cre resources for conditional mutagenesis. Mamm Genome. 2012; 23(9-10):587–599.


122. Schofield PN, Hoehndorf R, Gkoutos GV. Mouse genetic and phenotypic resources for human genetics. Hum Mutat. 2012; 33(5):826–836.


123. Sung YH, Baek IJ, Seong JK, Kim JS, Lee HW. Mouse genetics: catalogue and scissors. BMB Rep. 2012; 45(12):686–692.


124. Sharma S, Zhu J. Immunologic applications of conditional gene modification technology in the mouse. Curr Protoc Immunol. 2014; 105:1–13.


125. Tsien JZ. Cre-Lox Neurogenetics: 20 Years of Versatile Applications in Brain Research and Counting. Front Genet. 2016; 7:19.

