Abstract
This study was conducted to compare the anesthetic effects of 2,2,2-tribromoethanol (TBE, Avertin®) in ICR mice obtained from three different sources. TBE (2.5%) was intraperitoneally injected at three doses: high-dose group (500 mg/kg), intermediate-dose group (250 mg/kg), and low-dose group (125 mg/kg). Anesthesia time, recovery time, end-tidal peak CO2 (ETCO2), mean arterial blood pressure, heart rate, oxygen saturation (SpO2), body temperature, pH, PCO2, and PO2 of the arterial blood were measured. Stable anesthesia was induced by all doses of TBE and the anesthesia time was maintained exhibited dose dependency. No significant differences in anesthetic duration were found among the three different strains. However, the anesthesia time was longer in female than in male mice, and the duration of anesthesia was significantly longer in female than in male mice in the high-dose group. The recovery time was significantly longer for female than male mice in the intermediate- and high-dose groups. In the ICR strains tested, there were no significant differences in the mean arterial blood pressure, SPO2, arterial blood PCO2, and PO2, which decreased after TBE anesthesia, or in heart rate and ETCO2, which increased after TBE anesthesia. In addition, body temperature, blood biochemical markers, and histopathological changes of the liver, kidney, and lung were not significantly changed by TBE anesthesia. These results suggested that ICR mice from different sources exhibited similar overall responses to a single exposure to TBE anesthesia. In conclusion, TBE is a useful drug that can induce similar anesthetic effects in three different strains of ICR mice.
Mice are the most commonly used species in experimental studies; during these experiments, many surgical procedures are performed on various mice strains. Following the introduction of Swiss mice into the United States from Lausanne in Switzerland, ICR mice were first established as an outbred stock by Hauschka at the Institute for Cancer Research [1]. ICR mice are now widely used in safety and efficacy testing, cancer research, and biomedical studies [23].
The prevention of pain and distress in experimental animals is essential and can be achieved by the use of anesthetics and analgesics appropriate for biomedical research [4]. General anesthesia is a pharmacologic state involving amnesia, immobility, unconsciousness, and analgesia [5] and is mainly achieved by injection or inhalation anesthetics. 2,2,2-Tribromoethanol (tribromoethanol [TBE]; Avertin®) has been used as a general anesthetic for laboratory animals since 1980s. TBE induces the suppression of the respiratory and cardiovascular centers of the central nervous system [6] and rapidly induces short term anesthesia with rapid recovery [7]. Papaioannou and Fox [8] reported that TBE was an anesthetic agent with low mortality, a morbidity rate less than 1%, and no significant abdominal adhesions or inflammatory responses. However, TBE-induced side effects, such as peritonitis, intestinal ileus, serositis of the abdominal organs, and death, are known to occur in mice [9]. In addition, repeated TBE injection is reported to lead to high mortality [6], and there are differences in the anesthetic effects in some strains of inbred mice [1011]. However, no study has compared the anesthetic effects of TBE in ICR mice obtained from different sources. The present study was conducted to compare the anesthetic effects of the TBE in three strains of ICR mice (Korl:ICR, A:ICR, and B:ICR).
Six-week-old male and female ICR mice were obtained from three difference sources. Korl:ICR mice were kindly provided by the Department of Laboratory Animal Resources in the National Institute of Food and Drug Safety Evaluation (NIFDS, Cheongju, Korea), and the other ICR mice were purchased from two different vendors located in the United States (A:ICR) and Japan (B:ICR). All mice were given ad libitum access to a standard irradiated chow diet (Purina, Seoul, Korea) and filtered tap water. During the experiment, the mice were maintained in a specific pathogen-free environment under strict environmental conditions: light cycle (lights on, 07:00; lights off, 19:00); 22℃±2℃; and 50%±10% relative humidity. After an initial acclimation period (14 days), changes in general condition, mobility, appearance, reflexes, and deaths were monitored in all experimental animals; animals that displayed abnormal responses were excluded from the experiment. The mice were housed in the Kyungpook National University-Laboratory Animal Resources Center. All animal experiments were performed in accordance with the Guidelines for Animal Care and Use by Kyungpook National University.
2,2,2-Tribromoethanol (2%, Sigma-Aldrich, St. Louis, MO, USA), 100% ethanol, and 2-methyl-2-butanol were dissolved in sterile saline. A 40× stock solution of TBE was prepared. Aliquots of 25 µL were placed in 1.5 mL plastic tubes and stored at −20℃. Working solutions of 2.5% TBE were prepared from a fresh aliquot of stock dissolved in 9.75 mL sterile PBS (pH 7.4) by vortex mixing. Working solutions were stored away from light at 4℃ for a maximum of 1 week.
The experimental protocol and dosages are presented in Table 1. Male and female Korl:ICR, A:ICR, and B:ICR mice (n=126) were allocated into three groups: the low-dose group (125 mg/kg), the intermediate-dose group (250 mg/kg), and the high-dose group (500 mg/kg), with seven male and female mice in each group. The mice were weighed before receiving anesthesia. The anesthetic was injected intraperitoneally (i.p.) and the mice were kept on a thermostatically controlled heating pad maintained at approximately 38℃. The ambient temperature of the laboratory was maintained at 25℃. The onset of anesthesia was judged by a reduced righting reflex and the loss of pedal withdrawal reflex [1213]. Response to noxious stimulation was determined by a negative toe pinch response. Monitoring comprised oxygen saturation (SpO2, saturation of percutaneous oxygen) and heart rate using a pulse oximeter (PhysioSuite® MouseSTAT and CapnoScan, Kent Scientific Corp, Torrington, USA).
Anesthesia time and recovery time were measured based on the degree of relaxation of the jaw, skeletal muscle movement, swallowing reflex, corneal reflex, and presence of stimulus-response in the sole and the ear. The induction time was defined as the time from anesthetic injection to an absent response to noxious stimulation. The anesthetic duration was defined as the period of time without a response to noxious stimulation from anesthesia induction to anesthetic recovery. Anesthetic recovery was defined as the time required for movement, ambulation, and righting ability to return after the initial injection.
The blood pressure and heart rate of the mice were measured at 25℃ by using a tail-cuff pulse detection system (MK-2000 model, Muromachi Kikai Co., Tokyo, Japan) before and during the anesthesia. During the measurement of blood pressure, changes in body core temperature were measured using an electronic thermometer in the anus. Pulse oximetry and end-tidal peak CO2 (ETCO2) were measured during deep anesthesia.
Arterial blood gas analysis was performed by heart sampling during deep anesthesia by using an Epoc® portable blood gas analyzer (Epocal Inc., Canada). A blood sample (0.8 mL) was collected from the retroorbital plexus of each mouse after undergoing deep anesthesia and centrifuged (Centrifuge 5415R, Eppendorf, Germany) at 3,000 rpm for 15 min. The serum was separated and stored at −70℃ until analysis. Serum concentrations of aspartate aminotransferase (AST), alanine aminotransferase (ALT), alkaline phosphatase (ALP), glucose, creatinine, blood urea nitrogen (BUN), uric acid, total protein, albumin, calcium, phosphorus, total cholesterol (TC), and triglyceride (TG), and the albumin to globulin (A/G) ratio were measured by using commercial kits (Asan Pharmaceutical Co., Seoul, Korea) and an automatic chemistry analyzer (Hitachi 7180; Hitachi, Tokyo, Japan).
The animals were sacrificed by CO2 inhalation, and the liver, kidney, and lung tissues were removed, fixed with 4% paraformaldehyde, and embedded in paraffin. Paraffin block samples were cut into 5 µm sections and stained with hematoxylin and eosin (H&E) to enable histological observations.
The data were presented as the mean±standard deviation (SD). Statistical analysis was performed using SAS version 9.1 (SAS Institute Inc., Cary, NC, USA); Student's t-test was used for comparisons between the experimental groups, and a P value of <0.05 was considered to indicate statistical significance.
The anesthesia time and recovery time after the induction of TBE anesthesia in Korl:ICR, A:ICR, and B:ICR mice are shown in Figure 1. Stable anesthesia was induced at all doses of TBE and the time for which anesthesia permissible for surgical treatment was maintained was found to be dose dependent. Most mice reached an acceptable anesthetic state for surgical treatment, when the corneal reflex disappeared, within 2 min of injection in the intermediate- and high-dose groups. In Korl:ICR mice, anesthesia was maintained for 3.82–5.2 min, 13.5–16.8 min, and 21.5–34.5 min in the low-, intermediate-, and high-dose groups, respectively. In A:ICR mice, anesthesia was maintained for 3.1–4.9, 14.2–18.6, and 22.9–32.1 min in the low-, intermediate-, and high-dose groups, respectively. In B:ICR mice, anesthesia was maintained for 3.5–5.6, 14.0–17.9, and 20.3–36.8min in the low-, intermediate-, and high-dose groups, respectively. In all groups, the anesthesia time in female mice was longer than that in male mice, with larger differences observed as the dose increased; statistically significant differences were observed in the high-dose groups of all strains and the low-dose group in B:ICR mice. Anesthesia recovery time in Korl:ICR mice was 17.6–22.1, 36.1–71.5, and 61.0–103.4 min in the low-, intermediate-, and high-dose groups, respectively. The recovery time of female mice was longer than that of male mice, with statistically significant sex difference was observed in the intermediate- and high-dose groups. Anesthesia recovery times in A:ICR and B:ICR mice were 16.1–19.7 and 15.3–20.4 min, 41.2–68.4 and 31.5–64.5 min, and 70.1–98.5 and 64.2–107.2 min in the low-, intermediate-, and high-dose groups, respectively. Similar to the Korl:ICR mice, the recovery time of female mice was significantly longer than that of male mice in the intermediate- and high-dose groups. TBE dose was determined to have a slight, but not significant, effect on anesthesia time and recovery time in all ICR mice strains.
The changes in the mean arterial blood pressure (A), heart rate (B), ETCO2 (C), SpO2 (D) after the induction of TBE anesthesia in Korl:ICR, A:ICR, and B:ICR mice are presented in Figure 2. The mean arterial blood pressure tended to decrease as the dose of TBE increased. Although there was no significant difference between each ICR mice strain, the blood pressure of male mice was higher than that of female mice. Blood pressure before the induction of TBE anesthesia was highest Korl:ICR mice, followed by A:ICR and B:ICR mice, but the differences were not significant. Heart rate was higher after the induction of TBE anesthesia than before induction in all groups, although there were no significant differences between dose, sex, and ICR strain. ETCO2 increased rapidly in a dose-dependent manner after TBE anesthesia compared with before anesthesia and statistical significance observed in the group intermediate- and high-dose groups. SPO2 were reduced after the induction of TBE anesthesia in all ICR strains, but no dose- or sex-dependent differences were observed. The induction of TBE anesthesia did not result in statistically significant differences in body temperature by dose, sex, or ICR strain. The body temperature was between 37.5 and 38.0℃ before and after TBE anesthesia (data not shown).
The arterial blood PCO2, PO2, and pH after the induction of TBE anesthesia in Korl:ICR, A:ICR, and B:ICR are shown in Figure 3. The arterial blood PCO2 was decreased in a dose-dependent manner in all ICR strains after the induction of TBE anesthesia. Korl:ICR mice had the lowest PCO2, but the difference was not significance; B:ICR mice had the highest PCO2, but there was no significant difference between ICR strains or sex. The arterial blood PO2 tended to decrease in dose dependent manner after the induction of TBE anesthesia in all ICR strains, but there was no significant difference between strain or sex. The arterial blood pH was between 7.2 and 7.3 before and after TBE anesthesia, with no significant difference observed between the experimental groups (data not shown).
The changes in blood biochemical markers at the recommended anesthetic dose of 250 mg/kg TBE in Korl:ICR, A:ICR, and B:ICR mice are shown in Tables 1, 2, and 3, respectively. No significant differences in in blood biochemical markers were observed following the induction of TBE anesthesia in all experimental groups of ICR mice.
The histopathological changes in the liver, kidney, and lungs following anesthesia induced by low-, intermediate-, and high-doses of TBE in Korl:ICR, A:ICR, and B:ICR mice are shown in Figure 4. No significant differences in the effects of TBE anesthesia were observed in any experimental group.
In this study, the anesthesia time, recovery time, mean arterial blood pressure, heart rate, body temperature, ETCO2, SpO2, and PCO2, PO2, and pH of the arterial blood following the induction of TBE anesthesia were compared in Korl:ICR, A:ICR, and B:ICR mice.
TBE is a modest cardiodepressive injectable anesthetic used in biomedical research for short-term surgical procedures (5–30 min) for laboratory rodents and in embryo transfer procedures to produce transgenic mice [814]. TBE is metabolized in the liver by glucuronic acid conjugation [15], is generally administered by the intraperitoneal route, and is reported to produce good surgical anesthesia and muscle relaxation with rapid induction and recovery within 80 min [16]. The minimum cut-off dose for TBE is reported to be 200–250 mg/kg [17181920] and a dose of 240 mg/kg i.p. produces surgical anesthesia lasting 16–20 min, with good skeletal muscle relaxation, moderate respiratory depression, and full recovery within 40–90 min [212223]. In this study, 125 mg/kg was used as the lowest dose of TBE, 250 mg/kg was used as the intermediate dose, and 500 mg/kg was used as the highest dose; all doses were administered i.p. and all mice displayed clear signs of light anesthesia with a strong pedal withdrawal reflex. Gardner et al. [24] reported that 400 mg/kg of 2.5% TBE i.p. induced reduced righting reflex within 3 min and a mean time of adequate anesthesia of 6.9 min in male HSD:ICR mice. In this study, anesthesia time of 21.5–34.5 min was observed for male Korl:ICR mice in the high-dose group, which was comparatively long, despite the higher dose used. Voipio et al. reported that there were some differences in anesthetic effects on different strains of mice [10]. Mulder's experiment indicated strain differences, but not sex differences, of anesthetic duration in inbred mice [11]. Koizumi et al. evaluated sleep-time variation in outbred Jcl:ICR and MCH (ICR) mice administered TBE (400 mg/kg i.p.) [25]. The susceptibility of Jcl:ICR mice to TBE anesthesia was equivalent between experimental groups, but differed widely between male and female mice, with females more susceptible than males [25]. In the present study, there were no significant differences between the three ICR strains with regard to anesthesia time and recovery time, although anesthesia and recovery time were longer in female mice than in male mice. The results of our study confirmed differences in the response of male and female mice to TBE anesthesia.
Parameters such as oxygen saturation, heart rate, and respiratory rate have been reported to relate directly to anesthesia [26]. Anesthesia depresses the heart function and blood flow, which may affect both surface temperature and, potentially, blood flow to the peripheral nervous system. This study showed that the mean arterial blood pressure before anesthesia was higher in males than in females in all ICR stains. Male Korl:ICR had the highest blood pressure in non-anesthetic conditions and after the induction of TBE anesthesia; blood pressure was lower after TBE anesthesia, but there was no significant difference. In Korl:ICR mice, heart rate was higher during deep anesthesia with a dose of TBE 125 mg/kg and more than in non-anesthetic conditions, but there was no significant difference, and A:ICR and B:ICR mice exhibited the same pattern (Figure 2). Hart et al. [27] examined cardiac performance in male Swiss Webster mice receiving TBE (375 mg/kg), and found less bradycardia with smaller effects on cardiac loading and ventricular function. However, our results indicated that changes in blood pressure and heart rate were the major cardiovascular changes following a single induction of TBE anesthesia and that other factors were not major risk factors for ICR mice. Capnography is the measurement and display of the ETCO2 near the airway opening and has been performed in non-intubated patients by using a device that samples exhaled air through a nasal cannula [2829]. ETCO2 is used for a noninvasive estimate of PaCO2 [3031] and the continuous assessment of ETCO2 may help to optimize mechanical ventilation [32]. Our results showed that the ETCO2 increased with an increase in the dose of TBE, that PCO2 gas in the arterial blood increased, and that the arterial blood PO2 decreased. However, the pH of the arterial blood remained within the normal range, confirming that the balance of acid and base in the blood was controlled for the single induction of TBE anesthesia at doses of 125–500mg/kg. O2 saturation is one of the parameters used to estimate anesthetic depth and condition of laboratory animals under anesthesia [26]. Our results showed that SPO2 was reduced by approximately 15% in deep anesthesia, regardless of the TBE dose, ICR strain, or sex. There was almost no change in body temperature due to TBE anesthesia; it was presumed that normal body temperature was maintained by the thermostatic heat pad. The arterial blood PCO2 and PO2 were decreased in dose-dependent manner after the induction of TBE anesthesia in all ICR strains. These results showed that the single exposure to TBE anesthesia reduced arterial blood PCO2 as well as PO2, but that it was not sufficient to cause fatal heart and respiratory problems. Although anesthesia is generally known to affect cardiopulmonary function [1433], we concluded that cardiopulmonary function in the ICR strains tested were not significantly altered by a single exposure to TBE anesthesia. Christine et al. [34] reported the variability of anesthetic effectiveness, pathology, morbidity, and mortality associated with the use of TBE and did not recommend the use of TBE in female ICR mice. However, in this study, the safety of the anesthetic method for female ICR mice was observed, because no adverse events or deaths occurred. Our results showed that the most commonly recommended dose, 250 mg/kg, was capable of inducing deep anesthesia within 13–19 min, with greater differences between the anesthesia time in male and female mice observed in the high-dose groups. These sex differences after TBE administration may be affected by pharmacokinetic factors such as differences in drug metabolism and body composition of male and female mice.
Previous studies have shown that TBE elevates blood glucose [17], increases apoptosis and peritonitis, and that TBE toxicity was associated with the breakdown product, dibromoacetaldehyde [1735]. The liver enzymes, AST and ALT, were within the normal range for mice, which indicated that TBE anesthesia did not exert harmful effects on liver function. In addition, no mortality or adverse events were observed in Korl:ICR, A:ICR, B:ICR mice administered 500 mg/kg TBE. Some researchers have reported high rates of post-anesthesia mortality, peritonitis, intestinal ileus, and abdominal adhesions [36], whereas others have used TBE extensively with few adverse effects [37]. Thompson et al. [38] investigated the histopathological changes to the liver, spleen, heart, and kidney at 3 and 6 h after the onset of anesthesia (160 mg/kg TBE i.p.) in mice and also reported no detectable pathologic changes in the tissue. However, Goelz [39] concluded that TBE produced an anesthetic state in CD-1 mice, but that it was inconsistent and often variable. With regard to the safety of TBE in this study, all mice injected with TBE (125–500 mg/kg) recovered perfectly from anesthesia, with normal values for blood biochemical parameters and histological appearances. Therefore, we suggest that a single dose range of 125–500 mg/kg TBE is a safe and proper dosage to allow mice to recover quickly from anesthesia. In conclusion, the anesthetic response to TBE in three different strains of ICR mice was similar, but with some sex differences. However, in general, TBE was safe and resulted in stable anesthetic responses.
Acknowledgments
This project was supported by a grant of NLAR (National Laboratory Animal Resources) from Ministry of Food and Drug Safety in 2017.
References
1. Cui S, Chesson C, Hope R. Genetic variation within and between strains of outbred Swiss mice. Lab Anim. 1993; 27(2):116–123. PMID: 8501892.


2. Lehoczky JA, Cai WW, Douglas JA, Moran JL, Beier DR, Innis JW. Description and genetic mapping of Polypodia: an X-linked dominant mouse mutant with ectopic caudal limbs and other malformations. Mamm Genome. 2006; 17(9):903–913. PMID: 16964440.


3. Al-Awar A, Kupai K, Veszelka M, Szûcs G, Attieh Z, Murlasits Z, Török S, Pósa A, Varga C. Experimental Diabetes Mellitus in Different Animal Models. J Diabetes Res. 2016; 2016:9051426. PMID: 27595114.


4. Richardson CA, Flecknell PA. Anaesthesia and post-operative analgesia following experimental surgery in laboratory rodents: are we making progress? Altern Lab Anim. 2005; 33(2):119–127. PMID: 16180987.


5. Naguib M, Gottumukkala V, Goldstein PA. Melatonin and anesthesia: a clinical perspective. J Pineal Res. 2007; 42(1):12–21. PMID: 17198534.


6. Meyer RE, Fish RE. A review of tribromoethanol anesthesia for production of genetically engineered mice and rats. Lab Anim (NY). 2005; 34(10):47–52. PMID: 16261153.


7. Hill WA, Tubbs JT, Carter CL, Czarra JA, Newkirk KM, Sparer TE, Rohrbach B, Egger CM. Repeated administration of tribromoethanol in C57BL/6NHsd mice. J Am Assoc Lab Anim Sci. 2013; 52(2):176–179. PMID: 23562101.
8. Papaioannou VE, Fox JG. Efficacy of tribromoethanol anesthesia in mice. Lab Anim Sci. 1993; 43(2):189–192. PMID: 8320967.
9. Lieggi CC, Artwohl JE, Leszczynski JK, Rodriguez NA, Fickbohm BL, Fortman JD. Efficacy and safety of stored and newly prepared tribromoethanol in ICR mice. Contemp Top Lab Anim Sci. 2005; 44(1):17–22.
10. Voipio HM, Nevalainen T, Virtanen R. Evaluation of anaesthetic potency of medetomidine-ketamine combination in mice. In : IXth ICLAS International Symposium on Laboratory Animal Science Proceedings; Bangkok. 1988. p. 298–299.
11. Mulder JB. Anesthesia in the mouse using a combination of ketamine and promazine. Lab Anim Sci. 1978; 28:70–71. PMID: 633840.
12. Arras M, Autenried P, Rettich A, Spaeni D, Rülicke T. Optimization of intraperitoneal injection anesthesia in mice: drugs, dosages, adverse effects, and anesthesia depth. Comp Med. 2001; 51(5):443–456. PMID: 11924805.
13. Wixson SK, White WJ, Hughes HC Jr, Lang CM, Marshall WK. The effects of pentobarbital, fentanyl-droperidol, ketamine-xylazine and ketamine-diazepam on arterial blood pH, blood gases, mean arterial blood pressure and heart rate in adult male rats. Lab Anim Sci. 1987; 37(6):736–742. PMID: 3125387.
14. Chu DK, Jordan MC, Kim JK, Couto MA, Roos KP. Comparing isoflurane with tribromoethanol anesthesia for echocardiographic phenotyping of transgenic mice. J Am Assoc Lab Anim Sci. 2006; 45(4):8–13.
15. Fish RE. Pharmacology of injectable anesthetics. Anesthesia and Analgesia in Laboratory Animals. New York: Academic Pressv;1997. p. 1–28.
16. Weiss J, Zimmermann F. Tribromoethanol (Avertin) as an anaesthetic in mice. Lab Anim. 1999; 33(2):192–193. PMID: 10780824.


17. Brown ET, Umino Y, Loi T, Solessio E, Barlow R. Anesthesia can cause sustained hyperglycemia in C57/BL6J mice. Vis Neurosci. 2005; 22(5):615–618. PMID: 16332272.


18. Kubo Y, Tahara Y, Hirao A, Shibata S. 2,2,2-Tribromoethanol phase-shifts the circadian rhythm of the liver clock in Per2::Luciferase knockin mice: lack of dependence on anesthetic activity. J Pharmacol Exp Ther. 2012; 340(2):698–705. PMID: 22171092.


19. Norton WB, Scavizzi F, Smith CN, Dong W, Raspa M, Parker-Thornburg JV. Refinements for embryo implantation surgery in the mouse: comparison of injectable and inhalant anesthesias - tribromoethanol, ketamine and isoflurane - on pregnancy and pup survival. Lab Anim. 2016; 50(50):335–343. PMID: 26566637.


20. Oh SS, Hayes JM, Sims-Robinson C, Sullivan KA, Feldman EL. The effects of anesthesia on measures of nerve conduction velocity in male C57Bl6/J mice. Neurosci Lett. 2010; 483(2):127–131. PMID: 20691755.


21. Hogan B, Costantini F, Lacy E. Manipulating the mouse embryo: A laboratory manual. Cold Spring Harbor, NY: Cold Spring Harbor Laboratory;1986.
22. Buetow BS, Chen LI, Maggio-Price L, Swisshelm K. Peritonitis in Nude Mice in a Xenograft Study. Contemp Top Lab Anim Sci. 1999; 38(6):47–49.
23. Kohn DF, Wixson SK, White WJ, Benson GJ. Anesthesia and Analgesia in Laboratory Animals. San Diego: Academic Press;1997.
24. Gardner DJ, Davis JA, Weina PJ, Theune B. Comparison of tribromoethanol, ketamine/acetylpromazine, Telazol/xylazine, pentobarbital, and methoxyflurane anesthesia in HSD:ICR mice. Lab Anim Sci. 1995; 45(2):199–204. PMID: 7603025.
25. Koizumi T, Maeda H, Hioki K. Sleep-time variation for ethanol and the hypnotic drugs tribromoethanol, urethane, pentobarbital, and propofol within outbred ICR mice. Exp Anim. 2002; 51(2):119–124. PMID: 12012718.


26. Flecknell PA. Chapter 3, Analgesic management. Laboratory Animal Anaesthesia. 3rd ed. London: Academic Press;2009.
27. Hart CY, Burnett JC Jr, Redfield MM. Effects of avertin versus xylazine-ketamine anesthesia on cardiac function in normal mice. Am J Physiol Heart Circ Physiol. 2001; 281(5):H1938–H1945. PMID: 11668054.


28. Rousselon S, Coat M, Nguyen BV, Gouny P, Nowak E, Wargnier JP, Arvieux CC, Gueret G. [Comparison between ETCO2 values measured by the Smart Capnoline™ and the PACO2 in intubated then extubated postoperative cardiac surgery patients]. Ann Fr Anesth Reanim. 2011; 30(1):13–16. PMID: 21190808.
29. American Heart Association. 2005 American Heart Association (AHA) guidelines for cardiopulmonary resuscitation (CPR) and emergency cardiovascular care (ECC) of pediatric and neonatal patients: pediatric advanced life support. Pediatrics. 2006; 117(5):e1005–e1028. PMID: 16651281.
30. Benallal H, Busso T. Analysis of end-tidal and arterial PCO2 gradients using a breathing model. Eur J Appl Physiol. 2000; 83(4-5):402–408. PMID: 11138582.
31. Wasserman K, Hansen JE, Sue DY, Stringer WW, Whipp BJ. Normal values. Arterial and end-tidal carbon dioxide tensions. Principles of exercise testing and interpretation. 4th ed. Philadelphia: Lippincott Williams & Wilkins;2005.
32. Enghoff H. Volumen inefficax: Bemerkungen zur frage des schädlichen raumes. Upsala Lakareforen Forh. 1938; 44:191–218.
33. Roth DM, Swaney JS, Dalton ND, Gilpin EA, Ross J Jr. Impact of anesthesia on cardiac function during echocardiography in mice. Am J Physiol Heart Circ Physiol. 2002; 282(6):H2134–H2140. PMID: 12003821.
34. Lieggi CC, Artwohl JE, Leszczynski JK, Rodriguez NA, Fickbohm BL, Fortman JD. Efficacy and safety of stored and newly prepared tribromoethanol in ICR mice. Contemp Top Lab Anim Sci. 2005; 44(1):17–22.
35. Zeller W, Meier G, Bürki K, Panoussis B. Adverse effects of tribromoethanol as used in the production of transgenic mice. Lab Anim. 1998; 32(4):407–413. PMID: 9807753.


36. Flecknell PA. Chapter 2, Anesthesia. Laboratory Animal Anaesthesia. 3rd ed. London: Academic Press;2009.
37. Boyd RL, Halderman LW, Harris JO, Mangos JA. Strain differences in pulmonary function of laboratory rats. Lab Anim Sci. 1982; 32(1):42–43. PMID: 7078071.
38. Thompson JS, Brown SA, Khurdayan V, Zeynalzadedan A, Sullivan PG, Scheff SW. Early effects of tribromoethanol, ketamine/xylazine, pentobarbitol, and isoflurane anesthesia on hepatic and lymphoid tissue in ICR mice. Comp Med. 2002; 52(1):63–67. PMID: 11900415.
39. Goelz MF. Anesthetic and pathologic effects of tribromoethanol in mice. Toxicology Bibliographic Information (Toxline);1994.
Figure 1
Anesthesia time and recovery time in male and female ICR mice administered the TBE. The data shown represent the means±SD (n=7 per group). A P value less than 0.05 was considered to be statistically significant. *P<0.05 significant difference vs. male Korl:ICR group. #P<0.05 significant difference vs. male A:ICR group. §P<0.05 significant difference vs. male B:ICR group.
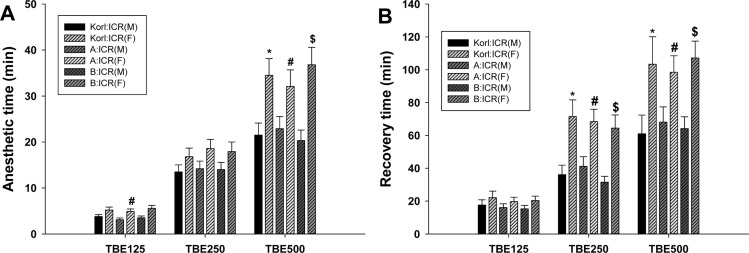
Figure 2
Mean arterial blood pressure, heart rate, end-tidal peak CO2, and SpO2 in male and female ICR mice administered the TBE. Data are presented as means±SD. A P value less than 0.05 was considered to be statistically significant. There were no significant differences between dose, sex, and ICR strain.
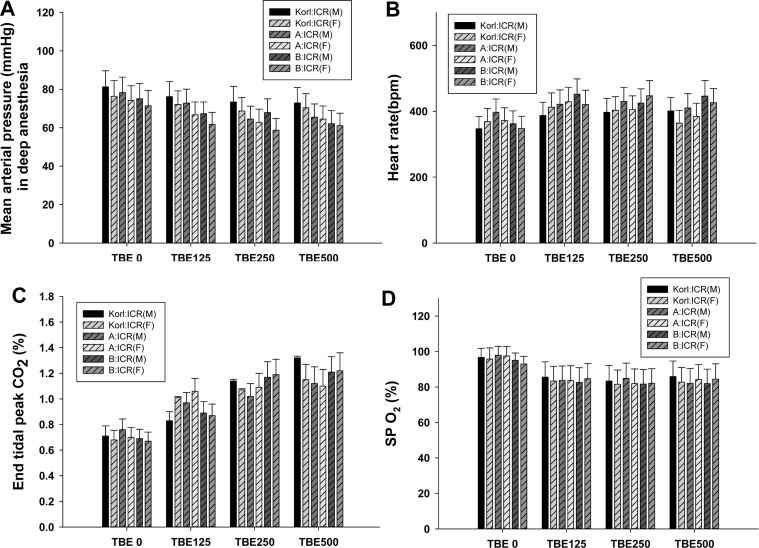
Figure 3
Arterial blood PCO2, and PO2 in male and female ICR mice administered the TBE. Data are presented as means±SD. A P value less than 0.05 was considered to be statistically significant. There were no significant differences between dose, sex, and ICR strain.
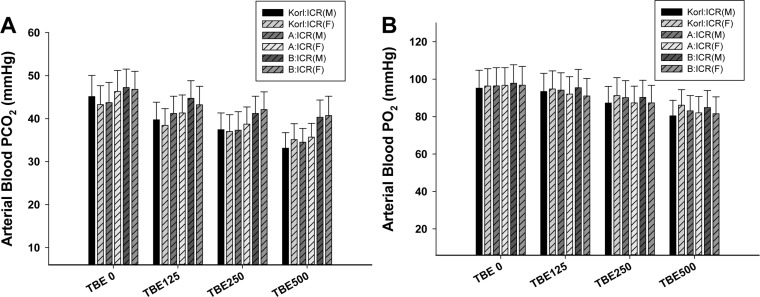
Figure 4
The histological appearances of liver, kidney, and lung in male and female ICR mice administered the TBE. The section were stained using hematoxylin and eosin. Magnification ×200.
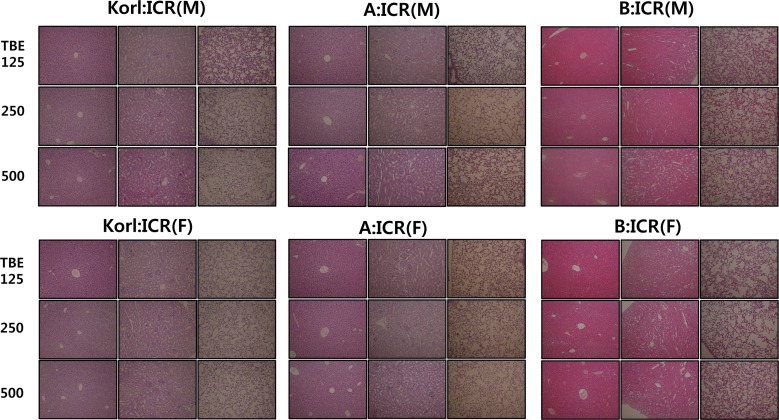
Table 1
Blood biochemical markers in male and female Korl:ICR mice administered the 250 mg/kg TBE
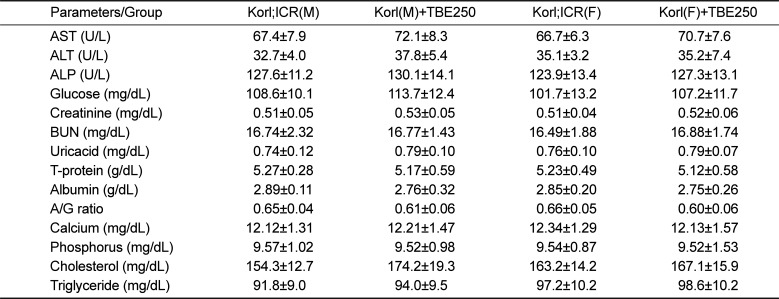
Table 2
Blood biochemical markers in male and female A:ICR mice administered the 250 mg/kg TBE
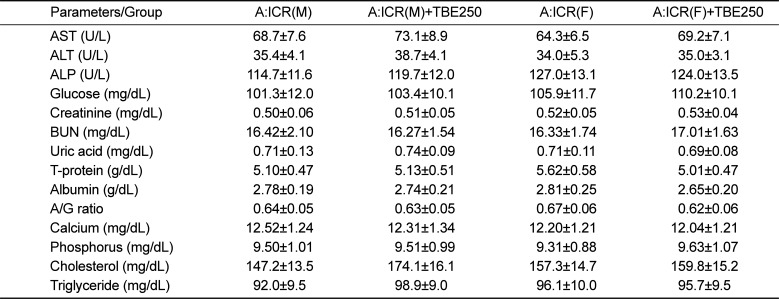
Table 3
Blood biochemical markers in male and female B:ICR mice administered the 250 mg/kg TBE
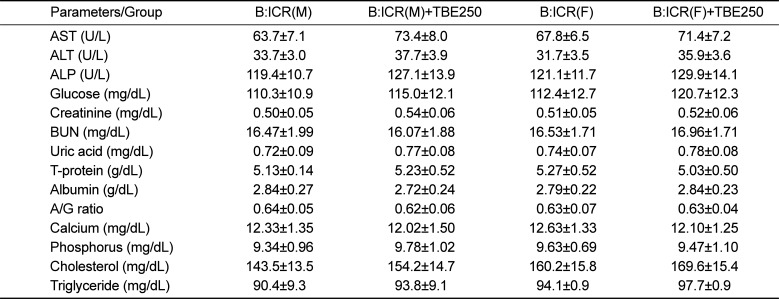