Abstract
Animal models have been used to elucidate the pathophysiology of varying diseases and to provide insight into potential targets for therapeutic intervention. Although alternatives to animal testing have been proposed to help overcome potential drawbacks related to animal experiments and avoid ethical issues, their use remains vital for the testing of new drug candidates and to identify the most effective strategies for therapeutic intervention. Particularly, the study of metabolic diseases requires the use of animal models to monitor whole-body physiology. In line with this, the National Institute of Food and Drug Safety Evaluation (NIFDS) in Korea has established their own animal strains to help evaluate both efficacy and safety during new drug development. The objective of this study was to characterize the response of C57BL/6NKorl mice from the NIFDS compared with that of other mice originating from the USA and Japan in a chemical-induced diabetic condition. Multiple low-dose treatments with streptozotocin were used to generate a type-1 diabetic animal model which is closely linked to the known clinical pathology of this disease. There were no significantly different responses observed between the varying streptozotocin-induced type-1 diabetic models tested in this study. When comparing control and diabetic mice, increases in liver weight and disturbances in serum amino acids levels of diabetic mice were most remarkable. Although the relationship between type-1 diabetes and BCAA has not been elucidated in this study, the results, which reveal a characteristic increase in diabetic mice of all origins are considered worthy of further study.
Diabetes mellitus is a metabolic disorder resulting from impaired insulin secretion of pancreatic β-cells and disrupted insulin action in target organs (eg, liver, muscle, adipose tissue), followed by hyperglycemia and secretion or lack of action of endogenous insulin [12]. Diabetes mellitus is classified into two types, insulin-dependent (type-1) and ii) non-insulin-dependent (type-2). Type-1 diabetes currently accounts for roughly 5–10% of all cases of diabetes, however, its incidence is constantly increasing worldwide [3]. Type-1 diabetes primarily occurs in individuals who experience destruction of the insulin-secreting pancreatic β-cell by a genetic defect-induced chronic immune response, and its symptoms are caused by hyperglycemia resulting from insufficient function insulin [456]. Currently, it is considered that insulin supplementation is an essential therapy in type-1 diabetes [7]. One important challenge facing patients with diabetes is that lasting hyperglycemia increases the risk of kidney disease, heart disease, retinal injury, nerve damage, and vascular damage [8]. Therefore, it is necessary to establish suitable animal models for research investigating novel prevention and treatment approaches for diabetes mellitus.
Streptozotocin (2-deoxy-2-(3-methyl-3-nitrosourea)-1-D-glucopyranose), a naturally occurring compound produced by soil bacterial streptomyces achromogenes, is a highly selective cytotoxic reagent in the pancreatic β cells. It induces an inflammatory response of the insulin-producing β cells followed by insulitis and absolute insulin deficiency [9]. Based on these mechanisms, streptozotocin is widely used to induce experimental type-1 diabetic animal models [101112]. Initially, it was administered at a high single dosage to induce complete death of pancreatic β cells within 2 days [13]. However, Like and Rossini noted that multiple challenges with low dose streptozotocin results in the slowly progressive type-1 diabetes and more closely resembles the clinical pathogenesis [141516]; this approach is now widely used to establish type-1 diabetes in animals.
The National Institute of Food and Drug Safety Evaluation (NIFDS, Cheongju, Korea) has established its own animal strains to evaluate both efficacy and safety during new drug development. The objective of this study was to characterize the response of the C57BL/6N mice from the NIFDS treated with streptozotocin compared with that of other mice originating from the USA and Japan. In this study, multiple low dose treatments with streptozotocin were used to generate type-1 diabetic animal models which closely relate to clinical pathology. Thus, a study comparing well-established animal disease model using streptozotocin can provide insight into the validity and usefulness of NIFDS-derived mice in preclinical testing of antidiabetic substances and elucidating the mechanism of the progression of type-1 diabetes in humans.
Male C57BL/6N mice were obtained from three different sources. C57BL/6NKorl were kindly provided by the Department of Laboratory Animal Resources in the National Institute of Food and Drug Safety Evaluation (NIFDS, Cheongju, Korea). The other two groups of C57BL/6N strain were purchased from different vendors located in the USA (referred C57BL/6NA) and Japan (referred C57BL/6NB). Animal protocol of this study was approved by the Institutional Animal Care and Use Committees at Pusan National University (PNU-2017-1632). Mice were acclimated to temperature (22±2) and humidity (55±5%)-controlled rooms with a 12 h light/dark cycle for seven days prior to use. They were randomly divided into two groups and the streptozotocin-treated group was given daily intraperitoneal injection with 50 mg/kg body weight of streptozotocin (Sigma, St. Louis, MO, USA) freshly dissolved in 0.1 M citrate buffer (pH 4.5) for 5 days. Body weight, water and food consumption were measured at least once a week after last dosing of streptozotocin. Liver and kidney tissues were harvested 4 weeks after the final administration.
Serum sample was obtained from blood of each mouse using BD Microtainer Serum Collection Tube (BD Life Sciences, Franklin Lakes, NJ, USA). The sera were stored at −80℃ until the biochemical analyses. Serum glucose level was determined using commercial Glucose CII-test Wako (Wako Pure Chemical Industries, Ltd., Tokyo, Japan). Activities of alanine aminotransferase (ALT) and aspartate aminotransferase (AST) were measured using the protocol of Reitman and Frankel [17]. They were quantified spectrophotometrically using an MULTISKAN GO reader (Thermo Scientific, Waltham, MA, USA). The serum levels of total cholesterol, HDL-cholesterol, and LDL-cholesterol were determined by using an Automated Chemistry Analyzer (Prestige 24I, Tokyo Boeki Medical System, Tokyo, Japan).
Metabolites in deproteinized serum were derivatized with O-phthalaldehyde/2-mercaptoethanol and quantified by HPLC with fluorescence detection (FLD-3100: Thermo Scientific, Waltham, MA, USA) on a HECTOR-T C18 column (3 µm×4.6 mm×100 mm; Chiral Technology Korea, Daejeon, Korea) at wavelengths of excitation 338 nm and emission 425 nm.
In mice treated with streptozotocin, the changes of body weight showed no significant difference compared with those of respective vehicle-treated mice during experimental period (Figure 1A). Significant increase in water intake were observed from 10 days after last dose of streptozotocin in C57BL/6NKorl and C57BL/6NB mice, and from 14 days in C57BL/6NA mice (Figure 1B). Food consumption of mice were slightly increased in streptozotocin-treated mice compared with those of respective vehicle-treated mice (Figure 1C).
Mice with greater than 300 mg/dL of blood glucose levels were considered diabetic in streptozotocin-induced model [18]. As shown in Figure 2A, serum glucose concentration of streptozotocin-treated mice was more than 300 mg/dL and it was successfully established in mice of all origins. The liver-to-body weight ratios (Figure 2B) were significantly increased 29, 42, and 21% in streptozotocin-treated C57BL/6NKorl, C57BL/6NA, C57BL/6NB, respectively. The weight of the kidney (Figure 2C) was not changed in the streptozotocin-treated mice.
Serum activity of ALT (Figure 3A) was significantly increased by the treatment of streptozotocin (C57BL/6NKorl, 1.74 fold; C57BL/6NA, 1.98 fold; C57BL/6NB, 1.60 fold). AST activity in serum showed slight increase with significance only in C57BL/6NA mice treated with streptozotocin (Figure 3B). There was no difference among the source of mice in the activities of both ALT and AST, suggesting that streptozotocin treatment affects liver function of diabetic mice.
As shown Figure 5, many kinds of metabolites in serum were changed by streptozotocin treatment. The concentration of aspartate, citrulline, methionine, serine, threonine, glutamate, taurine, lysine, glycine, and tryptophan were decreased in streptozotocin-induced diabetic mice. Whereas, phenylalanine, ornithine, leucine, valine, and isoleucine were increased in streptozotocin-induced diabetic mice. There was no changes in the serum levels of tyrosine, histidine, alanine, asparagine, and arginine streptozotocin treatment. The changed pattern of serum metabolites was no significant difference among the source of mice.
A number of animal models have been used to elucidate the pathophysiology of varying diseases and to provide insight into potential targets and approaches for therapeutic intervention. Although various alternatives to animal testing have been proposed to help overcome potential drawbacks related to animal experiments and avoid ethical issues, they are still considered critical to the drug development process for treating human diseases. In particular, the study of metabolic diseases (eg, diabetes and obesity), requires the use of animal models to monitor whole-body physiology [19]. One of the most widely used laboratory mouse strains in metabolic research is the inbred C57BL/6 mouse which is commonly referred to as “Black 6”, “B6”, or “C57 Black” [192021]. Inbred stains including the C57BL6 mouse are commonly used for biomedical and behavioral experiments, and data resulting from these studies have contributed substantially to the field's understanding of complicated biological processes [222324]. In accordance with this, NIFDS has established its own inbred line of C57BL6/N named “C57BL6/NKorl” in the Guidelines for Nomenclature of Mouse Strains and been providing the characteristic information for animal researchers [25].
A diabetogenic effect of streptozotocin was first reported by Junod et al. who found that its effect resulted from selective destruction of pancreatic islet β-cells [2627]. Several animal species are sensitive to the cytotoxic effect of streptozotocin and it is currently most often used to induce diabetes in rats and mice [28]. Specifically, multiple, lose-dose treatments of streptozotocin is increasingly being used to produce type-1 diabetic animal models which closely mimic symptoms seen in humans. In this study, a type-1 diabetic model of mice was induced by chronic injection of low dose streptozotocin and the response of C57BL/6NKorl mice was characterized by changes in basic physiology and serum metabolites. Furthermore, it was compared with the response of other mice obtained from two different commercially available sources.
No significantly different responses between the streptozotocin-induced type-1 diabetic mouse models used in this study were observed. When comparing control and diabetic mice, increases in liver weight and disturbances in serum amino acids levels of diabetic mice were most remarkable.
It has been suggested that increased triglyceride accumulation leads to an increase in the weight of livers from diabetic mouse model organisms because of the increased import of fatty acids into the liver induced by hypoinsulinemia and the low excretion of lipoprotein from the liver due to deficiency in the synthesis of apolipoprotein B [29303132]. In agreement with these reports, an important physiological change in this study is a significant increase in liver weight of streptozotocin-induced diabetic mice. This change is accompanied by increased activities of both ALT and AST suggesting that type-1 diabetes may induce abnormal liver functions.
Insulin leads to a decrease in serum amino acid levels through the stimulus of protein synthesis and the inhibition of proteolysis, which would explain the negative correlation between insulin dosage and plasma level of the amino acids in patients with diabetes [33]. It has been experimentally proven that insulin deficiency causes functional changes in the liver and muscle, which subsequently inhibits protein synthesis and promotes protein degradation. Interestingly, evidence is accumulating that there is a positive association between type-2 diabetes and circulating concentrations of BCAAs (eg, valine, leucine, isoleucine) [343536]. Of note, the serum levels of many amino acids in the type-1 diabetic mice used in this study were decreased, but the serum concentration of BCAA was increased, which is similar to that observed in type-2 diabetes. Although the relationship between type-1 diabetes and BCAA has not been elucidated in this study, the results revealing a characteristic increase in diabetic mice of all origin are considered to be worthy of further study.
In conclusion, the responses of C57BL/6NKorl mice following streptozotocin treatment (eg, physiological, biochemical, metabolic) are not significantly different among mice of different orgins. Thus, our results suggest the usefulness of C57BL/6NKorl mice in preclinical testing of antidiabetic substances and those aiming to better elucidate the mechanisms of type-1 diabetes progression in humans.
Acknowledgments
This project was supported by a grant of NLAR (National Laboratory Animal Resources) from Ministry of Food and Drug Safety in 2017.
References
1. Maritim AC, Sanders RA, Watkins JB 3rd. Diabetes, oxidative stress, and antioxidants: a review. J Biochem Mol Toxicol. 2003; 17(1):24–38. PMID: 12616644.


2. American Diabetes Association. Diagnosis and Classification of Diabetes Mellitus. Diabetes Care. 2014; 37(Suppl 1):S81–S90. PMID: 24357215.
3. Chao KC, Chao KF, Fu YS, Liu SH. Islet-like clusters derived from mesenchymal stem cells in Wharton's Jelly of the human umbilical cord for transplantation to control type 1 diabetes. PLoS One. 2008; 3(1):e1451. PMID: 18197261.


4. Diabetes Prevention Trial--Type 1 Diabetes Study Group. Effects of insulin in relatives of patients with type 1 diabetes mellitus. N Engl J Med. 2002; 346(22):1685–1691. PMID: 12037147.
5. van Belle TL, Coppieters KT, von Herrath MG. Type 1 diabetes: etiology, immunology, and therapeutic strategies. Physiol Rev. 2011; 91(1):79–118. PMID: 21248163.


6. Knip M, Veijola R, Virtanen SM, Hyöty H, Vaarala O, Akerblom HK. Environmental triggers and determinants of type 1 diabetes. Diabetes. 2005; 54(Suppl 2):S125–S136. PMID: 16306330.


7. Skyler JS, Cefalu WT, Kourides IA, Landschulz WH, Balagtas CC, Cheng SL, Gelfand RA. Efficacy of inhaled human insulin in type 1 diabetes mellitus: a randomised proof-of-concept study. Lancet. 2001; 357(9253):331–335. PMID: 11210993.


8. Polat K, Güneş S. An expert system approach based on principal component analysis and adaptive neuro-fuzzy inference system to diagnosis of diabetes disease. Digital Signal Processing. 2007; 17(4):702–710.


9. Oelze M, Knorr M, Schuhmacher S, Heeren T, Otto C, Schulz E, Reifenberg K, Wenzel P, Münzel T, Daiber A. Vascular dysfunction in streptozotocin-induced experimental diabetes strictly depends on insulin deficiency. J Vasc Res. 2011; 48(4):275–284. PMID: 21273782.


10. Lenzen S. The mechanisms of alloxan- and streptozotocin-induced diabetes. Diabetologia. 2008; 51(2):216–226. PMID: 18087688.


11. Szkudelski T. The mechanism of alloxan and streptozotocin action in B cells of the rat pancreas. Physiol Res. 2001; 50(6):537–546. PMID: 11829314.
12. Eleazu CO, Eleazu KC, Chukwuma S, Essien UN. Review of the mechanism of cell death resulting from streptozotocin challenge in experimental animals, its practical use and potential risk to humans. J Diabetes Metab Disord. 2013; 12(1):60. PMID: 24364898.


13. Kolb H. Mouse models of insulin dependent diabetes: low-dose streptozocin-induced diabetes and nonobese diabetic (NOD) mice. Diabetes Metab Rev. 1987; 3(3):751–778. PMID: 2956075.


14. Like AA, Rossini AA. Streptozotocin-induced pancreatic insulitis: new model of diabetes mellitus. Science. 1976; 193(4251):415–417. PMID: 180605.


15. Wu KK, Huan Y. Diabetic atherosclerosis mouse models. Atherosclerosis. 2007; 191(2):241–249. PMID: 16979174.


16. Weide LG, Lacy PE. Low-dose streptozocin-induced autoimmune diabetes in islet transplantation model. Diabetes. 1991; 40(9):1157–1162. PMID: 1834505.


17. REITMAN S, FRANKEL S. A colorimetric method for the determination of serum glutamic oxalacetic and glutamic pyruvic transaminases. Am J Clin Pathol. 1957; 28(1):56–63. PMID: 13458125.


18. Motyl K, McCabe LR. Streptozotocin, type I diabetes severity and bone. Biol Proced Online. 2009; 11:296–315. PMID: 19495918.


19. Fontaine DA, Davis DB. Attention to Background Strain Is Essential for Metabolic Research: C57BL/6 and the International Knockout Mouse Consortium. Diabetes. 2016; 65(1):25–33. PMID: 26696638.


20. Collins S, Martin TL, Surwit RS, Robidoux J. Genetic vulnerability to diet-induced obesity in the C57BL/6J mouse: physiological and molecular characteristics. Physiol Behav. 2004; 81(2):243–248. PMID: 15159170.


21. Winzell MS, Ahrén B. The high-fat diet-fed mouse: a model for studying mechanisms and treatment of impaired glucose tolerance and type 2 diabetes. Diabetes. 2004; 53(Suppl 3):S215–S219. PMID: 15561913.
22. Mashimo T, Serikawa T. Rat resources in biomedical research. Curr Pharm Biotechnol. 2009; 10(2):214–220. PMID: 19199954.


23. Croy BA. The 1999 Reginald Thomson Lecture. Custom-built mice: unique discovery tools in biomedical research. Can Vet J. 2000; 41(3):201–206. PMID: 10738597.
24. Ardaillou R. [Transgenic mice: a major advance in biomedical research]. Bull Acad Natl Med. 2009; 193(8):1773–1782. PMID: 20669542.
25. Sundberg JP, Schofield PN. Commentary: mouse genetic nomenclature. Standardization of strain, gene, and protein symbols. Vet Pathol. 2010; 47(6):1100–1104. PMID: 20685919.
26. Junod A, Lambert AE, Stauffacher W, Renold AE. Diabetogenic action of streptozotocin: relationship of dose to metabolic response. J Clin Invest. 1969; 48(11):2129–2139. PMID: 4241908.


27. Junod A, Lambert AE, Orci L, Pictet R, Gonet AE, Renold AE. Studies of the diabetogenic action of streptozotocin. Proc Soc Exp Biol Med. 1967; 126(1):201–205. PMID: 4864021.


28. Furman BL. Streptozotocin-Induced Diabetic Models in Mice and Rats. Curr Protoc Pharmacol. 2015; 70:5.47.1–5.47.20.


29. Habibuddin M, Daghriri HA, Humaira T, Al Qahtani MS, Hefzi AA. Antidiabetic effect of alcoholic extract of Caralluma sinaica L. on streptozotocin-induced diabetic rabbits. J Ethnopharmacol. 2008; 117(2):215–220. PMID: 18359177.


30. Lee SI, Kim JS, Oh SH, Park KY, Lee HG, Kim SD. Antihyperglycemic effect of Fomitopsis pinicola extracts in streptozotocin-induced diabetic rats. J Med Food. 2008; 11(3):518–524. PMID: 18800901.


31. Merzouk H, Madani S, Chabane Sari D, Prost J, Bouchenak M, Belleville J. Time course of changes in serum glucose, insulin, lipids and tissue lipase activities in macrosomic offspring of rats with streptozotocin-induced diabetes. Clin Sci (Lond). 2000; 98(1):21–30. PMID: 10600655.


32. Ohno T, Horio F, Tanaka S, Terada M, Namikawa T, Kitoh J. Fatty liver and hyperlipidemia in IDDM (insulin-dependent diabetes mellitus) of streptozotocin-treated shrews. Life Sci. 2000; 66(2):125–131. PMID: 10666008.


33. Grill V, Björkman O, Gutniak M, Lindqvist M. Brain uptake and release of amino acids in nondiabetic and insulin-dependent diabetic subjects: important role of glutamine release for nitrogen balance. Metabolism. 1992; 41(1):28–32. PMID: 1538641.


34. Newgard CB, An J, Bain JR, Muehlbauer MJ, Stevens RD, Lien LF, Haqq AM, Shah SH, Arlotto M, Slentz CA, Rochon J, Gallup D, Ilkayeva O, Wenner BR, Yancy WS Jr, Eisenson H, Musante G, Surwit RS, Millington DS, Butler MD, Svetkey LP. A branched-chain amino acid-related metabolic signature that differentiates obese and lean humans and contributes to insulin resistance. Cell Metab. 2009; 9(4):311–326. PMID: 19356713.


35. Shah SH, Crosslin DR, Haynes CS, Nelson S, Turer CB, Stevens RD, Muehlbauer MJ, Wenner BR, Bain JR, Laferrère B, Gorroochurn P, Teixeira J, Brantley PJ, Stevens VJ, Hollis JF, Appel LJ, Lien LF, Batch B, Newgard CB, Svetkey LP. Branched-chain amino acid levels are associated with improvement in insulin resistance with weight loss. Diabetologia. 2012; 55(2):321–330. PMID: 22065088.


36. McCormack SE, Shaham O, McCarthy MA, Deik AA, Wang TJ, Gerszten RE, Clish CB, Mootha VK, Grinspoon SK, Fleischman A. Circulating branched-chain amino acid concentrations are associated with obesity and future insulin resistance in children and adolescents. Pediatr Obes. 2013; 8(1):52–61. PMID: 22961720.


Figure 1
Changes in (A) body weight, (B) water and (C) food consumption after final administration of streptozotocin. Mice were given daily intraperitoneal injection with vehicle or 50 mg/kg body weight of streptozotocin (STZ) for 5 days. Changes were measured at least once a week after last dosing of streptozotocin during 4 weeks.
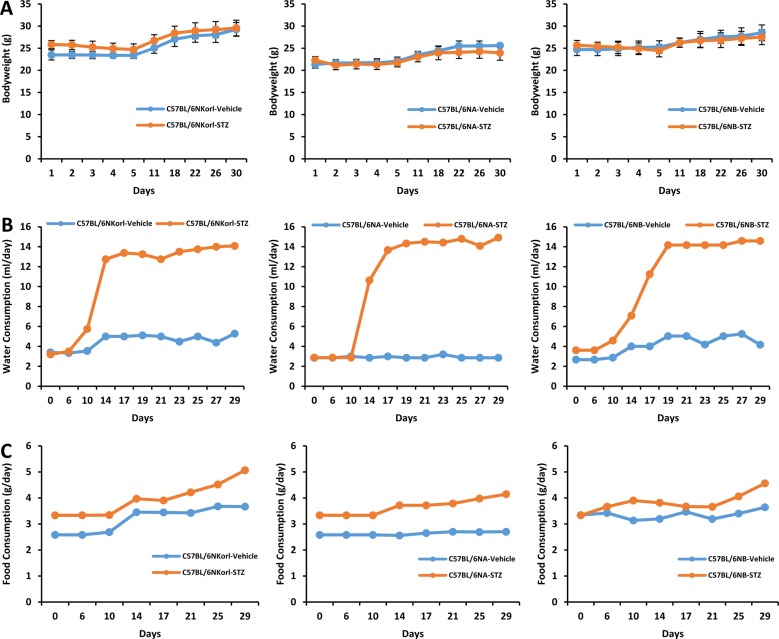
Figure 2
Changes of (A) serum glucose level, and relative ratio of (B) liver-to-body and (C) kidney-to-body weight in streptozotocin-treated mice. Mice were given daily intraperitoneal injection with vehicle or 50 mg/kg body weight of streptozotocin (STZ) for 5 days. Changes were examined 4 weeks after final administration. ***Significantly different from the corresponding vehicle-treated mice (ANOVA followed by Newman-Keuls multiple range test, P<0.001, respectively).
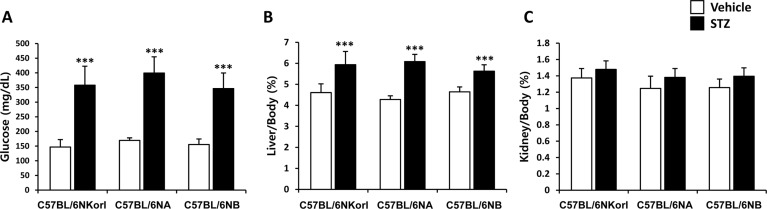
Figure 3
Effect of streptozotocin on the activities of (A) ALT and (B) AST. Mice were given daily intraperitoneal injection with vehicle or 50 mg/kg body weight of streptozotocin (STZ) for 5 days. Activities of ALT and AST were examined 4 weeks after final administration. *,**Significantly different from the corresponding vehicle-treated mice (ANOVA followed by Newman-Keuls multiple range test, P<0.05, 0.01, respectively).
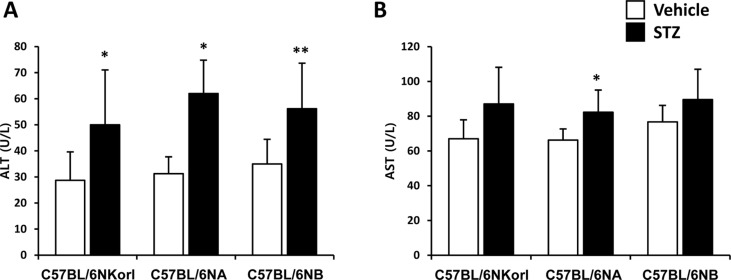
Figure 4
Changes in (A) total cholesterol, (B) HDL-cholesterol, and (C) LDL-cholesterol in streptozotocin-treated mice. Mice were given daily intraperitoneal injection with vehicle or 50 mg/kg body weight of streptozotocin (STZ) for 5 days. Changes were examined 4 weeks after final administration. *Significantly different from the corresponding vehicle-treated mice (ANOVA followed by Newman-Keuls multiple range test, P<0.05, respectively).
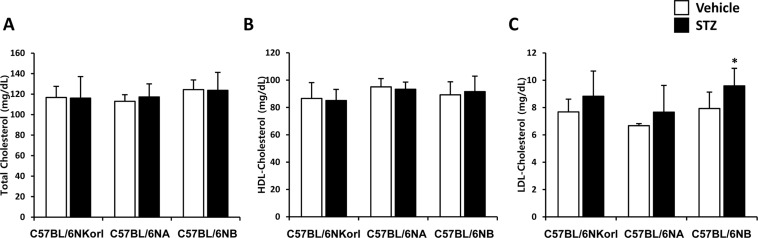
Figure 5
Heat map of changes in serum metabolites between vehicle- and streptozotocin (STZ)-treated mice. Mice were given daily intraperitoneal injection with vehicle or 50 mg/kg body weight of streptozotocin (STZ) for 5 days. Serum metabolites were determined 4 weeks after final administration. Each column represents one mouse sample and each row represents a different metabolite.
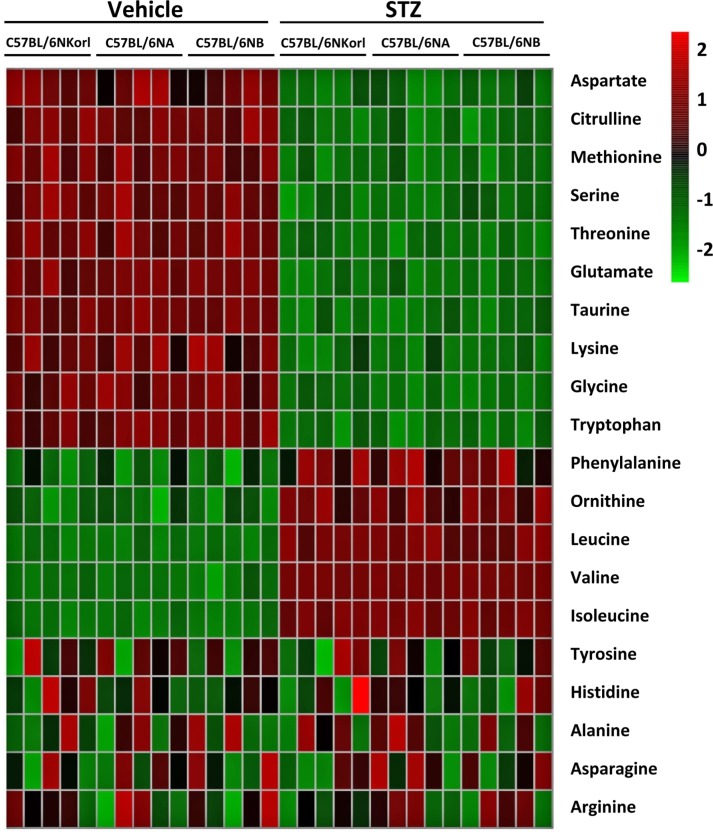