Abstract
Objectives
The purpose of this study was to evaluate the synergic effect of recombinant human bone morphogenetic protein-2 (rhBMP-2) and low-level laser therapy (LLLT) on bisphosphonate-treated osteoblasts.
Materials and Methods
Human fetal osteoblast cells (hFOB 1.19) were cultured with 100 µM alendronate. Low-level Ga-Al-As laser alone or with 100 ng/mL rhBMP-2 was then applied. Cell viability was measured with MTT assay. The expression levels of receptor activator of nuclear factor kappa-B ligand (RANKL), macrophage colony-stimulating factor (M-CSF), and osteoprotegerin (OPG) were analyzed for osteoblastic activity inducing osteoclastic activity. Collagen type and transforming growth factor beta-1 were also evaluated for bone matrix formation.
Bisphosphonates (BPs) are synthetic drugs that are effective at inhibiting bone resorption. They are used for treatment of various osseous diseases such as osteoporosis, multiple myeloma, and bone metastasis of tumors and other conditions that cause bone fragility1. BP-related osteonecrosis of the jaw (BRONJ) is an undesirable by-product of BPs that appears in the oral and maxillofacial area23. The majority of BRONJ cases has been diagnosed in patients administered high doses of intravenous BPs for treatment/prevention of cancer-related malignances4. A smaller number have been reported in patients prescribed oral BPs for treatment of osteoprorosis5. BPs directly affect osteoclast precursors, resulting in decreased osteoclastogenesis, and indirectly stimulate osteoblasts to produce an inhibitor of osteoclast formation678. Osteoblasts and their stromal cells regulate osteoclastogenesis by producing proteins such as macrophage colony-stimulating factor (M-CSF), receptor activator of nuclear factor kappa-B ligand (RANKL), and osteoprotegerin (OPG). RANKL and M-CSF stimulate osteoclast progenitor cells in osteoclasts and promote bone resorption. OPG is a RANKL antagonist that inhibits bone resorption91011.
Some investigators have studied the effects of various low-level laser therapy (LLLT) protocols on fibroblasts and osteoblast-like cell proliferation in vitro, but few studies have analyzed the effects of these protocols on osteoprogenitors present in bone marrow such as mesenchymal cells and osteoclast precursor cells12. It was observed that LLLT had a stimulating effect on decreased cellular activity due to its biostimulatory characteristics.
Bone morphogenetic protein (BMP) is a subclass of transforming growth factor-beta (TGF-β) superfamilies and induces bone and cartilage formation. Urist first introduced the concept that growth factors can induce bone formation, regardless of the condition of the bone tissue13. To date, there are more than 20 known BMP subgroups, of which BMP-2 is the primary, utilized in many in vitro and in vivo research studies14. Recombinant human BMP-2 (rhBMP-2), a bone healing activating factor, exhibits osteogenic effects and has been used widely in dental clinics. Through a previous study, we know that rhBMP-2 and LLLT have stimulating effects on BP-treated osteoblasts15. In this study, we investigated the synergic effect of rhBMP-2 and LLLT on BP-treated osteoblasts.
Human fetal osteoblast cells (hFOB 1.19) were purchased from American Type Culture Collection (ATCC, Rockville, MD, USA) and cultured in an incubator at 34℃ with a 5% CO2 atmosphere. The culture medium consisted of a 1:1 mixture of Dulbecco's modified Eagle medium (DMEM) and F12 (Invitrogen, Carlsbad, CA, USA), supplemented with 10% fetal bovine serum (Invitrogen).
Cells were cultured on culture dishes and/or in several types of wells for 24 hours, after which the original medium was removed, and the cells were washed with phosphate-buffered saline (PBS). Alendronate (Sigma, St. Louis, MO, USA) stock solution was added to the cells in fresh medium to achieve final drug concentrations of 0, 1, 10, 50, 100, and 200 µM, followed by incubation for 24 to 72 hours. After alendronate application, rhBMP-2 (Cowellmedi, Busan, Korea) was also added to the cells in the medium to achieve concentrations of 0, 10, 25, 50, 75, and 100 ng/mL, followed by incubation for 24 to 72 hours.
The cells (1×105) were seeded in a 24-well plate. Following alendronate and rhBMP-2 treatment, a gallium-arsenide-aluminum (Ga-As-Al) laser (λ=808±3 nm, 80 mW, 80 mA; NDLUX, Seoul, Korea) was used for laser irradiation in a dark environment to prevent influences from other light sources. Laser energy was positioned above each well and applied continuously and vertically to the cells. The handpiece of the laser was fixed at 5 cm above the bottom of the culture plate, and the plate was moved to irradiate one well at a time. Laser irradiation was applied one time to each well for a duration of 15 seconds, and the total energy irradiated to each well was 1.2 J/cm2.
The MTT assay was used to monitor cell survivability. A total of 1×104 cells was seeded into each well on a 96-well plate, followed by incubation for 24 hours. Following incubation, alendronate was added to the cells, with or without rhBMP-2 and low level laser irradiation, and the cells were incubated for 24 to 72 hours. Afterward, the cells were treated with 500 µg/mL of MTT stock solution and incubated at 34℃ in a 5% CO2 atmosphere for 4 hours. The medium was removed, and formazan crystals were dissolved in dimethyl sulfoxide. Cell survivability was monitored with an enzyme-linked immunosorbent assay reader (Tecan, Männedorf, Switzerland) at an excitatory emission wavelength of 570 nm.
Western blot analysis was performed using mouse soluble RANKL (sRANKL), OPG, BMP-2, TGF-β1, osteopontin, poly (ADP-ribose) polymerase (PARP) antibodies, collagen type 1, and rabbit polyclonal anti-human GAPDH antibody (Santa Cruz Biotechnology, Santa Cruz, CA, USA). The cells (1×106) were cultured in 6-well plates. Following incubation with alendronate for 24 hours, aliquots of hFOB cells were either treated or not treated with rhBMP-2 and low-level laser irradiation. The cells were washed twice with ice-cold PBS and centrifuged at 750g for 10 minutes. Total cell proteins were extracted by lysis with RIPA buffer (300 mM NaCl, 50 mM Tris-HCl [pH 7.6], 0.5% TritonX-100, 2 mM PMSF, 2 µg/mL aprotinin, and 2 µg/mL leupeptin) and incubated at 4℃ for 1 hour. The lysates were then centrifuged at 14,000g for 15 minutes at 4℃, and sodium dodecyl sulfate (SDS) and sodium deoxycholic acid were added to final concentrations of 0.2%, respectively. The protein concentrations of the cell lysates were determined using a Bradford protein assay kit (Bio-Rad Laboratories, Richmond, CA, USA), with bovine serum albumin (BSA) as the protein standard. A 20 µg protein sample of each well was analyzed by 10% SDS-PAGE gel. Following separation, the gels were transferred to a polyvinylidene fluoride sheet (Amersham GE Healthcare, Little Chalfont, UK) and reacted with each antibody. Immunostaining with antibodies was performed by a Super Signal West Femto enhanced-chemiluminescence substrate and detected using an Alpha Imager HP (Alpha Innotech, Santa Clara, CA, USA). Equivalent protein loading for each gel was confirmed by Ponceau S staining. Quantification of protein bands densitometry was carried out using ImageJ 1.34s software (National Institutes of Health, Bethesda, MD, USA).
hFOB cells were plated on 18 mm coverslips and treated with alendronate with/without rhBMP-2 and low-level laser irradiation for 1 day. The cells were washed twice with prewarmed PBS and fixed with 4% formaldehyde solution in PBS for 10 minutes at room temperature. The samples were washed two times with PBS and then underwent permeabilization with Triton X-100, followed by blocking with 10% goat serum in PBS. The cells were stained for 30 minutes with fluorescent phalloidin for detection of actin and then incubated with collagen type I (Santa Cruz Biotechnology) in 3% BSA overnight at 4℃. On the following day, the cells were washed with PBS, incubated with FITC-conjugated secondary antibodies (Santa Cruz Biotechnology) in 3% BSA-PBS for 60 minutes, rinsed in PBS, and finally mounted on slides using ProLong Gold antifade reagent with DAPI (Invitrogen, Eugene, OR, USA). Fluorescent images were observed and analyzed using a laser-scanning confocal microscope (Carl Zeiss, Göettingen, Germany).
Total RNA was extracted from hFOB cells using spin columns (Rneasy; Qiagen, Hilden, Germany) according to the manufacturer's instructions. RNA (2 µg) was reverse-transcribed using a RevertAid First-Stand Synthesis System kit for real-time polymerase chain reaction (RT-PCR; Thermo Fisher Scientific, Pittsburgh, PA, USA) according to the manufacturer's protocol. cDNA was amplified with a PCR Master Mix SYBR Green kit (Applied Biosystems, Warrington, UK), and PCR amplification was performed using a Chromo4 Real-Time PCR Detection System (Bio-Rad Laboratories). PCR conditions were as follows: incubation at 95℃ for 3 minutes, followed by 40 cycles of incubation at 95℃ for 15 seconds and 60℃ for 30 seconds. After the last cycle, melting curve analysis was performed at 0.5℃ intervals between temperatures of 55℃ to 95℃. The sense and antisense primer sequences for RANKL, OPG, M-CSF, and the housekeeping gene GAPDH are shown in Table 1.
Cell survivability assays were performed using hFOB cells incubated with alendronate concentrations of 0, 1, 10, 50, 100, and 200 µM for periods of 24, 48, and 72 hours.(Fig. 1) At 24 hours of incubation, cells treated with alendronate concentrations ≥100 µM exhibited significantly decreased survivability. To evaluate the effects of rhBMP-2 on hFOB cells, cell survivability was assayed at 24, 48, and 72 hours following treatment with rhBMP-2 at concentrations of 0, 10, 25, 50, 75, and 100 ng/mL.(Fig. 2) While the results showed significantly increased cell survivability at higher rhBMP-2 concentrations, there was no difference in survivability at the different time points. The effect of rhBMP-2 on alendronate-treated hFOB cells was investigated by performing cell survivability assays using cells treated with alendronate and rhBMP-2 at concentrations of 100 µM and 100 ng/mL, respectively, at 24- and 48-hour incubation times.(Fig. 3) At the 24-hour time point, treatment with rhBMP-2 was shown to significantly increase cell survivability. To investigate the effects of LLLT, cells in the various groups were subjected to LLL irradiation.(Fig. 4) LLLT was shown to increase cell survivability and also demonstrated a synergic effect with rhBMP-2. While LLLT seemed to have a greater effect than rhBMP-2 on cell survivability, the difference was not statistically significant.
To determine whether alendronate, rhBMP-2, and LLLT altered the expression of differentiation factors, all groups of cells were analyzed for secretion of TGF-β1 and BMP-2. The results showed that, compared to controls, treatment with 100 µM alendronate (negative control) resulted in decreased expression of both TGF-β1 and BMP-2. However, expression of TGF-β1 and BMP-2 increased following treatment with either rhBMP-2 or LLLT, and the effects of these two agents were similar in magnitude. When rhBMP-2 and LLLT were applied together, the effect was increased, and TGF-β1 expression was increased more than expression of BMP-2. Deposition of TGF-β1 was confirmed by immuno-fluorescence microscopy. The staining density around the nucleus decreased following treatment with alendronate but was restored following treatment with rhBMP-2 and LLLT.(Fig. 5, 6)
To determine whether alendronate, rhBMP-2, and LLLT have an effect on bone matrix formation, all groups of cells were analyzed for expression of collagen type I and osteopontin. The results showed that, compared to the controls, secretion of both collagen type I and osteopontin decreased after treatment with 100 µM alendronate (negative control). However, the decrease in collagen type I secretion was not statistically significant. The expression of collagen type I increased following application of rhBMP-2 and LLLT, with LLLT having a greater effect on osteopontin expression. Deposition of collagen type I was confirmed by immunofluorescence microscopy. The density of cytoplasm decreased following treatment with alendronate but was restored by treatment with rhBMP-2 and LLLT.(Fig. 7, 8)
RT-PCR was performed to investigate the expression of RANKL, OPG, and M-CSF, which are proteins-related to osteoclastogenesis. Compared to the control group, treatment with alendronate resulted in reduced expression of RANKL, OPG, and M-CSF. However, expression of all 3 cytokines increased following application of rhBMP-2 or LLLT. Treatment with rhBMP-2 resulted in greater effects on OPG and M-CSF expression, while treatment with LLLT exhibited a greater effect on expression of RANKL. However, the differences between the effects of rhBMP-2 and LLLT were not statistically significant. The combined application of rhBMP-2 and LLLT resulted in greater increases in cytokine expression than application of either treatment by itself.(Fig. 9)
In the present study, we studied the effects of alendronate, the most frequently prescribed oral BP in Korea. The strong inhibition of osteoclast function precipitated by BP therapy can lead to inhibition of normal bone turnover16. The toxic effects of BPs on osteoclasts are well understood and are thought to influence the occurrence of BRONJ. Additional complex mechanisms and events may have a correlation with BRONJ development, and there are various interactions between bone cells that must be considered17. Although the majority of BP studies conducted in vitro has focused on events occurring in osteoclastic lineage cells, recent studies suggest that BPs may also have a stimulatory effect on osteoblasts. García-Moreno et al.18 reported that, while high concentrations of alendronate inhibited osteoblast proliferation, lower concentrations produced no significant effects. This effect may depend on the soluble factors of osteoblasts that inhibit osteoclast formation and activiation1920.
Two factors supplied by osteoblast lineage cells are of critical importance for osteoclastogenesis: M-CSF and RANKL. M-CSF binds to receptors on osteoclasts to induce differentiation, which ultimately leads to increased plasma calcium level through resorption of bone. M-CSF is required for proliferation and survival of osteoclast precursors, while RANKL is critical for precursor differentiation into mature, multinucleated osteoclasts. RANKL binding to receptor activator of nuclear factor kappa-B (RANK) results in rapid differentiation of osteoclast precursors to form mature osteoclasts. In addition to these positive regulators, osteoblast lineage cells produce OPG, which is the major inhibitor of osteoclast differentiation. Specifically, OPG is a member of the tumor necrosis factor receptor family and a soluble decoy receptor that ultimately binds to RANKL by binding and activating RANK. When OPG binds to RANK, there is rapid cessation of osteoclast formation, activation, and survival, leading to initiation of subsequent bone formation21.
TGF-1 is a member of the TGF-superfamily and one of the primary cytokines involved in growth and remodeling of bone. During bone resorption, active forms of these growth factors are released into the extracellular environment.
Osteopontin has been implicated as an important factor in bone remodeling and is synthesized by a variety of cell types22232425. Specifically, research suggests that osteopontin plays a role in anchoring osteoclasts to the mineral matrix of bones26.
In the present study, we investigated the expression of RANKL, OPG, and M-CSF in BP-treated hFOB cells for their effects on osteoclastogenesis. In addition, we surveyed the expression of TGF-β1 and BMP-2 for their roles as differentiation factors and also investigated the expression of collagen type I and osteopontin as factors involved in formation of bony matrix.
García-Moreno et al.18 evaluated the cytotoxic effects of alendronate on primary human osteoblasts and found no viable cells after 48 hours of culture at high alendronate concentrations. Additionally, synthesis of type I collagen, a vital component of the bony matrix, was completely inhibited. The present study also showed that alendronate had significant effects on osteoblast survival. Cells treated with alendronate for 24 hours at concentrations ≥100 µM exhibited significantly decreased survival. Additionally, the expression of both TGF-β1 and BMP-2 decreased following treatment with 100 µM alendronate (negative control). The expression of RANKL, OPG, and M-CSF, which are related to osteoclastogenesis, also decreased. These results suggest that high concentrations of alendronate suppress osteoblastic activity and osteoclastogenesis.
BMP-2 is well recognized as most effective for bone generation and has been approved for clinical use by the U.S. Food and Drug Administration. BMP-2 induces bone and cartilage formation and also plays a key role in osteoblast differentiation. Itoh et al.27 reported that BMP-2 enhanced the survival of purified osteoclasts supported by RANKL, but not by M-CSF. Zheng et al.28 showed that the BMP-2/7 heterodimer affects every osteoclastogenic event in a complicated dose-dependent manner and could increase the number of osteoclasts at concentrations of 10 to 150 ng/mL. In our investigation, we assayed the survivability of hFOB-2 cells following 24, 48, and 72 hours of incubation with various concentrations of rhBMP-2. We found that cell survival was slightly increased at higher rhBMP-2 concentrations, but was not affected by incubation time. Additionally, decreased expression of TGF-β1, BMP-2, RANKL, OPG, and M-CSF recovered to normal level after addition of rhBMP-2.
In the clinical field, diode lasers have been used predominantly for application as LLLT or biostimulation, and many studies have evaluated the efficacy of LLLT on various disorders29. In our study, the expression of collagen type I and BMP increased after treatment with rhBMP-2 and LLLT. These results were consistent with those of previous studies showing that LLLT promotes osteoblast proliferation and increases BMP expression30313233. Hirata et al.34 demonstrated that LLLT increases type I collagen expression in osteoblastic cells. Moreover, we observed significant increases of RANKL and M-CSF expression in cells treated with both LLLT and alendronate compared to alendronate-only treated cells. We suggest that LLLT influences the expression of RANKL, M-CSF, OPG, TGF-β1, and BMP to produce positive effects on bone osteoclastogenesis cell differentiation. The combined application of rhBMP-2 and LLLT exhibited a synergic effect, especially for TGF-β1.
While the expression of collagen type I and osteopontin slightly increased in each case, there was no evidence of a synergistic effect. This implies that formation of the bony matrix increased. Our study demonstrates the positive effects of rhBMP-2 and LLLT for partially recovering from the inhibitory effects of BPs and provides an experimental basis for the effectiveness of combining rhBMP-2 and LLLT as a treatment modality for BRONJ. In addition, the results showed that combined use of these modalities increased their therapeutic effectiveness compared to use of either modality alone. Therefore, we suggest that combined use of rhBMP-2 and LLLT will be more effective than the separate use of either modality for treatment of BRONJ.
The expression of osteoclastogenesis-related proteins RANKL, OPG, and M-CSF in hFOB cells decreased following treatment with alendronate and increased following application of rhBMP-2 and LLLT. Additionally, while alendronate suppressed the expression of TGF-β1 and BMP-2, which functions in cell differentiation, this expression recovered following combined application of rhBMP-2 and LLLT. Studies on expression of collagen type I and osteopontin, which are related to formation of the bony matrix, showed similar results. In all cases, combined application of rhBMP-2 and LLLT was more effective than application of either modality alone.
Notes
Authors' Contributions: S.Y.J. and J.M.S participated in data collection and wrote the manuscript. J.U.H. and C.H.K. participated in the study design and performed the statistical analysis. B.S.P., I.R.K., and S.H.S. participated in the study design and coordination and helped to draft the manuscript. All authors read and approved the final manuscript.
References
1. Boonyapakorn T, Schirmer I, Reichart PA, Sturm I, Massenkeil G. Bisphosphonate-induced osteonecrosis of the jaws: prospective study of 80 patients with multiple myeloma and other malignancies. Oral Oncol. 2008; 44:857–869. PMID: 18282788.


2. Marx RE. Pamidronate (Aredia) and zoledronate (Zometa) induced avascular necrosis of the jaws: a growing epidemic. J Oral Maxillofac Surg. 2003; 61:1115–1117. PMID: 12966493.


3. Lee JK, Kim KW, Choi JY, Moon SY, Kim SG, Kim CH, et al. Bisphosphonates-related osteonecrosis of the jaw in Korea: a preliminary report. J Korean Assoc Oral Maxillofac Surg. 2013; 39:9–13. PMID: 24471011.


4. Woo SB, Hellstein JW, Kalmar JP. Narrative [corrected] review: bisphosphonates and osteonecrosis of the jaws. Ann Intern Med. 2006; 144:753–761. PMID: 16702591.
5. Mavrokokki T, Cheng A, Stein B, Goss A. Nature and frequency of bisphosphonate-associated osteonecrosis of the jaws in Australia. J Oral Maxillofac Surg. 2007; 65:415–423. PMID: 17307586.


6. Rodan GA, Fleisch HA. Bisphosphonates: mechanisms of action. J Clin Invest. 1996; 97:2692–2696. PMID: 8675678.


7. Sahni M, Guenther HL, Fleisch H, Collin P, Martin TJ. Bisphosphonates act on rat bone resorption through the mediation of osteoblasts. J Clin Invest. 1993; 91:2004–2011. PMID: 8486770.


8. Murakami H, Takahashi N, Sasaki T, Udagawa N, Tanaka S, Nakamura I, et al. A possible mechanism of the specific action of bisphosphonates on osteoclasts: tiludronate preferentially affects polarized osteoclasts having ruffled borders. Bone. 1995; 17:137–144. PMID: 8554921.


9. Aubin JE, Triffitt JT. Mesenchymal stem cells and the osteoblast lineage. In : Bilezikian JP, Raisz LG, Rodan GA, editors. Principles of bone biology. 2nd ed. New York: Academic;2002.
10. Tay JY, Bay BH, Yeo JF, Harris M, Meghji S, Dheen ST. Identification of RANKL in osteolytic lesions of the facial skeleton. J Dent Res. 2004; 83:349–353. PMID: 15044512.


11. Lacey DL, Timms E, Tan HL, Kelley MJ, Dunstan CR, Burgess T, et al. Osteoprotegerin ligand is a cytokine that regulates osteoclast differentiation and activation. Cell. 1998; 93:165–176. PMID: 9568710.


12. Khadra M, Lyngstadaas SP, Haanaes HR, Mustafa K. Effect of laser therapy on attachment, proliferation and differentiation of human osteoblast-like cells cultured on titanium implant material. Biomaterials. 2005; 26:3503–3509. PMID: 15621240.


14. Chen D, Zhao M, Mundy GR. Bone morphogenetic proteins. Growth Factors. 2004; 22:233–241. PMID: 15621726.


15. Kwon TK, Song JM, Kim IR, Park BS, Kim CH, Cheong IK, et al. Effect of recombinant human bone morphogenetic protein-2 on bisphosphonate-treated osteoblasts. J Korean Assoc Oral Maxillofac Surg. 2014; 40:291–296. PMID: 25551094.


16. Marx RE. Clinical concerns of alendronate use. J Oral Maxillofac Surg. 2008; 66:1322. PMID: 18486814.


17. Rustemeyer J, Bremerich A. Bisphosphonate-associated osteonecrosis of the jaw: what do we currently know? A survey of knowledge given in the recent literature. Clin Oral Investig. 2009; 14:59–64.


18. García-Moreno C, Serrano S, Nacher M, Farré M, Díez A, Mariñoso ML, et al. Effect of alendronate on cultured normal human osteoblasts. Bone. 1998; 22:233–239. PMID: 9580147.


19. Vitté C, Fleisch H, Guenther HL. Bisphosphonates induce osteoblasts to secrete an inhibitor of osteoclast-mediated resorption. Endocrinology. 1996; 137:2324–2333. PMID: 8641182.


20. Nishikawa M, Akatsu T, Katayama Y, Yasutomo Y, Kado S, Kugal N, et al. Bisphosphonates act on osteoblastic cells and inhibit osteoclast formation in mouse marrow cultures. Bone. 1996; 18:9–14. PMID: 8717530.


21. Marini H, Minutoli L, Polito F, Bitto A, Altavilla D, Atteritano M, et al. OPG and sRANKL serum concentrations in osteopenic, postmenopausal women after 2-year genistein administration. J bone Miner Res. 2008; 23:715–720. PMID: 18433304.


22. Choi ST, Kim JH, Kang EJ, Lee SW, Park MC, Park YB, et al. Osteopontin might be involved in bone remodelling rather than in inflammation in ankylosing spondylitis. Rheumatology (Oxford). 2008; 47:1775–1779. PMID: 18854347.


23. Ashizawa N, Graf K, Do YS, Nunohiro T, Giachelli CM, Meehan WP, et al. Osteopontin is produced by rat cardiac fibroblasts and mediates A(II)-induced DNA synthesis and collagen gel contraction. J Clin Invest. 1996; 98:2218–2227. PMID: 8941637.


24. Murry CE, Giachelli CM, Schwartz SM, Vracko R. Macrophages express osteopontin during repair of myocardial necrosis. Am J Pathol. 1994; 145:1450–1462. PMID: 7992848.
25. Ikeda T, Shirasawa T, Esaki Y, Yoshiki S, Hirokawa K. Osteopontin mRNA is expressed by smooth muscle-derived foam cells in human atherosclerotic lesions of the aorta. J Clin Invest. 1993; 92:2814–2820. PMID: 8254036.


26. Reinholt FP, Hultenby K, Oldberg A, Heinegård D. Osteopontin: a possible anchor of osteoclasts to bone. Proc Natl Acad Sci U S A. 1990; 87:4473–4475. PMID: 1693772.
27. Itoh K, Udagawa N, Katagiri T, Iemura S, Ueno N, Yasuda H, et al. Bone morphogenetic protein 2 stimulates osteoclast differentiation and survival supported by receptor activator of nuclear factorkappaB ligand. Endocrinology. 2001; 142:3656–3662. PMID: 11459815.
28. Zheng Y, Wang L, Zhang X, Zhang X, Gu Z, Wu G. BMP2/7 heterodimer can modulate all cellular events of the in vitro RANKL-mediated osteoclastogenesis, respectively, in different dose patterns. Tissue Eng Part A. 2012; 18:621–630. PMID: 21981321.
29. Goldman L, Goldman B, Van Lieu N. Current laser dentistry. Lasers Surg Med. 1987; 6:559–562. PMID: 3573930.


30. Stein A, Benayahu D, Maltz L, Oron U. Low-level laser irradiation promotes proliferation and differentiation of human osteoblasts in vitro. Photomed Laser Surg. 2005; 23:161–166. PMID: 15910179.
31. Renno AC, McDonnell PA, Parizotto NA, Laakso EL. The effects of laser irradiation on osteoblast and osteosarcoma cell proliferation and differentiation in vitro. Photomed Laser Surg. 2007; 25:275–280. PMID: 17803384.


32. Kiyosaki T, Mitsui N, Suzuki N, Shimizu N. Low-level laser therapy stimulates mineralization via increased Runx2 expression and ERK phosphorylation in osteoblasts. Photomed Laser Surg. 2010; 28(Suppl 1):S167–S172. PMID: 20649430.


33. Shin SH, Kim KH, Choi NR, Kim IR, Park BS, Kim YD, et al. Effect of low-level laser therapy on bisphosphonate-treated osteoblasts. Maxillofac Plast Reconstr Surg. 2016; 38:48. PMID: 27995121.


34. Hirata S, Kitamura C, Fukushima H, Nakamichi I, Abiko Y, Terashita M, et al. Low-level laser irradiation enhances BMP-induced osteoblast differentiation by stimulating the BMP/Smad signaling pathway. J Cell Biochem. 2010; 111:1445–1452. PMID: 20830741.


Fig. 1
Survivability of human fetal osteoblast cells (hFOB 1.19) following treatment with different concentrations of alendronate. Cells treated with alendronate concentrations ≥100 µM exhibited significantly decreased survival. *P<0.05, compared to the control group.
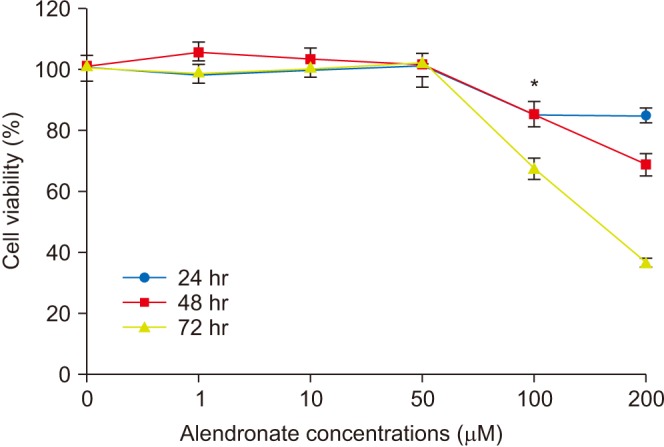
Fig. 2
Survivability of human fetal osteoblast cells (hFOB 1.19) following treatment with different concentrations of recombinant human bone morphogenetic protein-2 (rhBMP-2). Cell survival was significantly increased at higher rhBMP-2 concentrations, while no difference was observed at each time point. *P<0.05, compared to the control group.
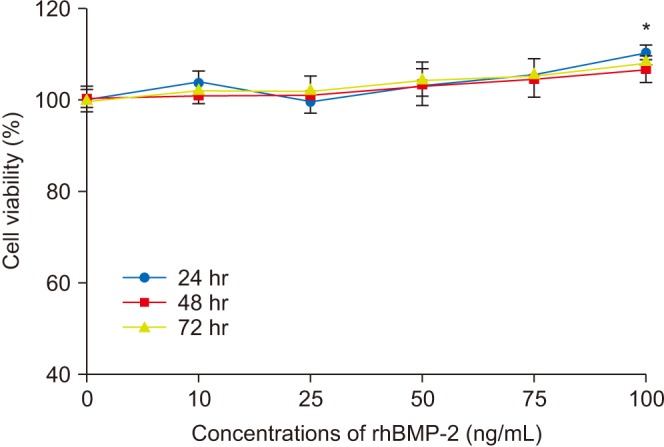
Fig. 3
Survivability of human fetal osteoblast cells (hFOB 1.19) following treatment with 100 µM alendronate and recombinant human bone morphogenetic protein-2 (rhBMP-2). *P<0.05, compared to the control group; †P<0.05, compared to the alendronate group.
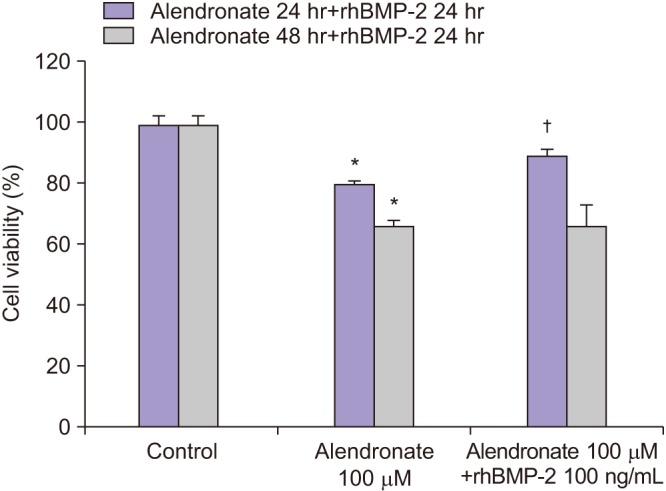
Fig. 4
Survivability of human fetal osteoblast cells (hFOB 1.19) following treatment with 100 µM alendronate, recombinant human bone morphogenetic protein-2 (rhBMP-2), and low-level laser therapy (LLLT). LLLT was shown to increase cell survivability and also demonstrated a synergic effect with rhBMP-2. *P<0.05, compared to the control group; †P<0.05, compared to the alendronate group. (a: control, b: alendronate+fresh media, c: alendronate+rhBMP-2 100 ng/mL, d: alendronate+LLLT [80 mW, 15 seconds], e: alendronate+rhBMP-2/LLLT [80 mW, 15 seconds])
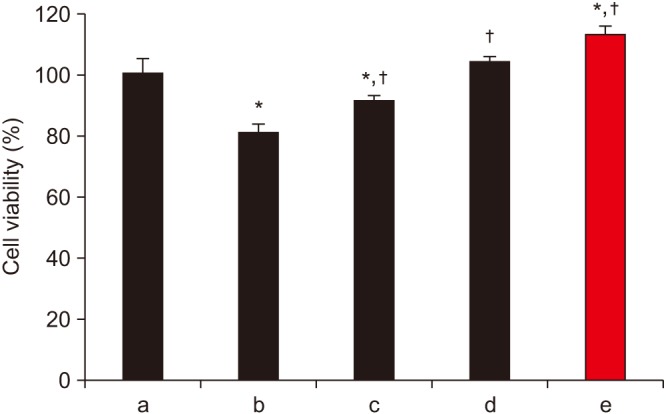
Fig. 5
Effects of alendronate, recombinant human bone morphogenetic protein-2 (rhBMP-2), and low-level laser therapy (LLLT) on expression of transforming growth factor-beta1 (TGF-β1). A. Confocal microscopic assay for expression of TGF-β1. B. Western blot assay for expression of TGF-β1. Expression of TGF-β1 increased when following treatment with either rhBMP-2 or LLLT. The staining density around the nucleus decreased following treatment with alendronate but was restored by rhBMP-2 and LLLT. (a: control, b: alendronate 100 µM, c: alendronate 100 µM+rhBMP-2 100 ng/mL, d: alendronate 100 µM+LLLT [80 mW, 15 seconds], e: alendronate 100 µM+rhBMP-2/LLLT [80 mW, 15 seconds])
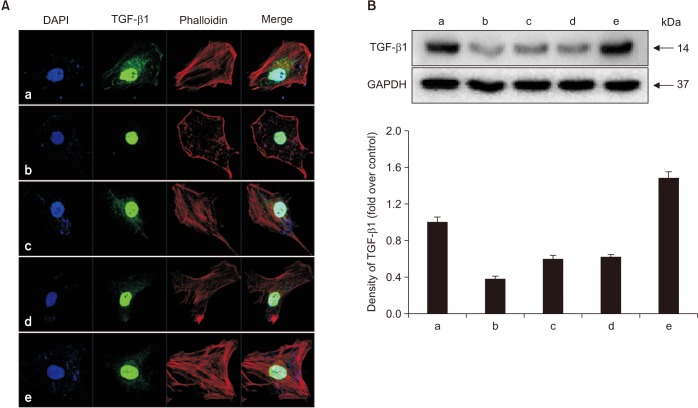
Fig. 6
Effects of alendronate, recombinant human bone morphogenetic protein-2 (rhBMP-2), and low-level laser therapy (LLLT) on expression of BMP-2. Western blot assay for BMP-2 expression. When rhBMP-2 and LLLT were applied together, the effect was increased, and transforming growth factor-beta1 expression was increased more than that of BMP-2. (a: control, b: alendronate 100 µM, c: alendronate 100 µM+rhBMP-2 100 ng/mL, d: alendronate 100 µM+LLLT [80 mW, 15 seconds], e: alendronate 100 µM+rhBMP-2/LLLT [80 mW, 15 seconds])
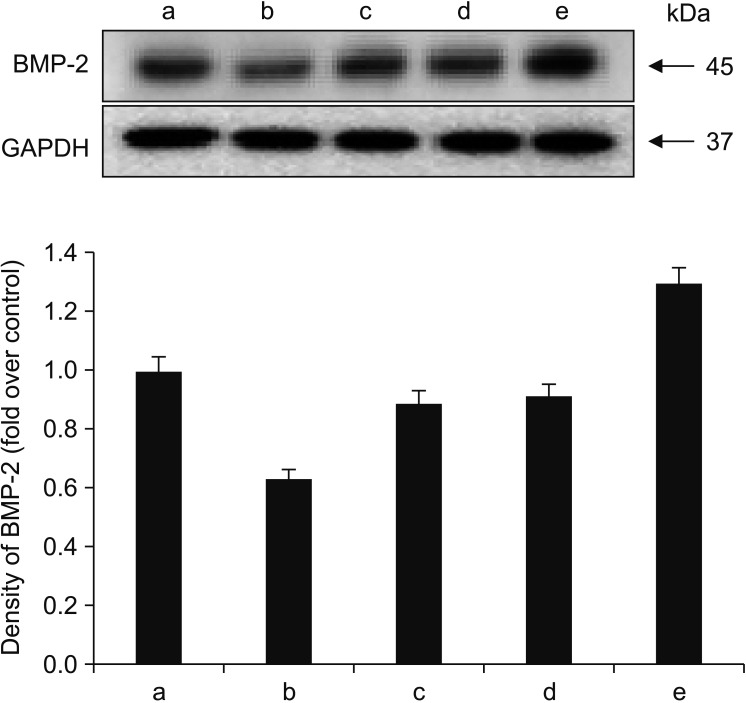
Fig. 7
Effects of alendronate, recombinant human bone morphogenetic protein-2 (rhBMP-2), and low-level laser therapy (LLLT) on expression of collagen type I. A. Confocal microscopic assay for expression of collagen type I. B. Western blot assay for expression of collagen type I. Expression of collagen type I increased following application of rhBMP-2 and LLLT. The decreased density of cytoplasm with alendronate was restored by treatment with rhBMP-2 and LLLT. (a: control, b: alendronate 100 µM, c: alendronate 100 µM+rhBMP-2 100 ng/mL, d: alendronate 100 µM+LLLT [80 mW, 15 seconds], e: alendronate 100 µM+rhBMP-2/LLLT [80 mW, 15 seconds])
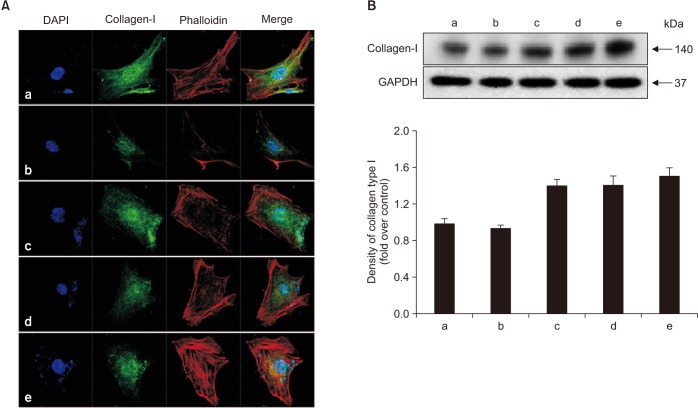
Fig. 8
Effects of alendronate, recombinant human bone morphogenetic protein-2 (rhBMP-2), and low-level laser therapy (LLLT) on expression of osteopontin. Western blot assay for expression of osteopontin. LLLT exhibited a greater effect on osteopontin expression. (a: control, b: alendronate 100 µM, c: alendronate 100 µM+rhBMP-2 100 ng/mL, d: alendronate 100 µM+LLLT [80 mW, 15 seconds], e: alendronate 100 µM+rhBMP-2/LLLT [80 mW, 15 seconds])
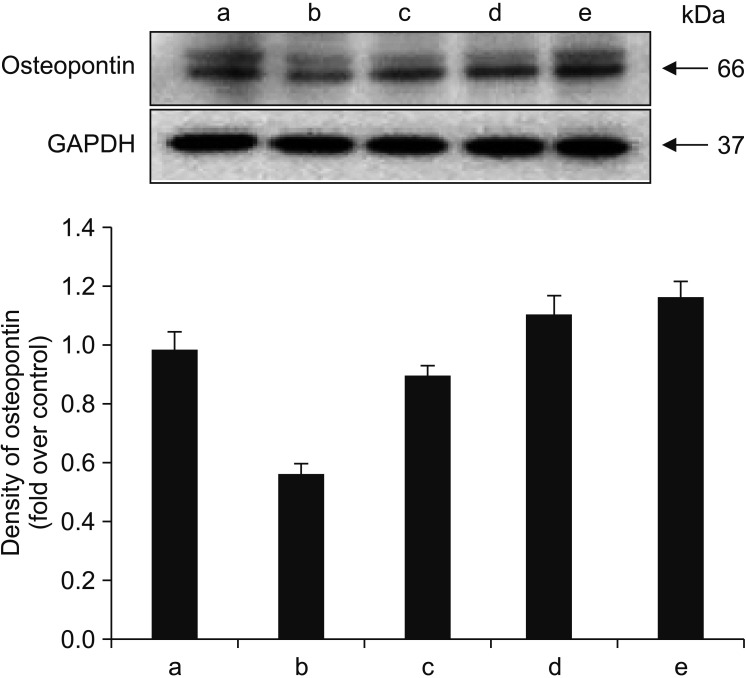
Fig. 9
Effects of alendronate, recombinant human bone morphogenetic protein-2 (rhBMP-2), and low-level laser therapy (LLLT) on receptor activator of nuclear factor kappa-B (RANKL), osteoprotegerin (OPG), and macrophage colony-stimulating factor (M-CSF) expression as determined by real-time polymerase chain reaction. A. RANKL expression. B. OPG expression. C. M-CSF expression. Treatment with rhBMP-2 resulted in greater effects on OPG and M-CSF expression, while treatment with LLLT had an effect on expression of RANKL. However, the differences between the effects of rhBMP-2 and LLLT were not statistically significant. *P<0.05, compared to the control group; †P<0.05, compared to the alendronate group. (a: control, b: alendronate 100 µM, c: alendronate 100 µM+rhBMP-2 100 ng/mL, d: alendronate 100 µM+LLLT [80 mW, 15 seconds], e: alendronate 100 µM+rhBMP-2/LLLT [80 mW, 15 seconds])
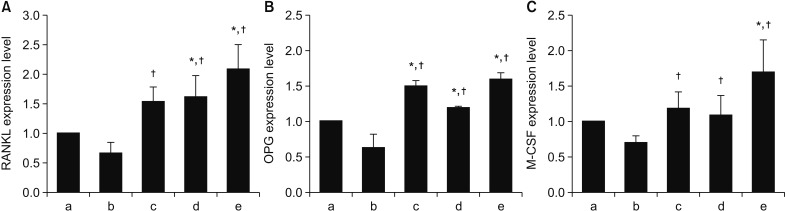