Abstract
Background and Purpose
We aimed to determine the association between the annual changes in dopamine transporter (DAT) availability as measured by 123I-ioflupane (123I-FP-CIT) single-photon-emission computed tomography and single-nucleotide polymorphisms (SNPs) known to be risk factors in Parkinson's disease (PD).
Methods
In total, 150 PD patients were included from the Parkinson's Progression Markers Initiative database. Specific SNPs that are associated with PD were selected for genotyping. SNPs that were not in Hardy-Weinberg equilibrium or whose minor allele frequency was less than 0.05 were excluded. Twenty-three SNPs met the inclusion criteria for this study. The Kruskal-Wallis test was used to compare annual percentage changes in DAT availability for three subgroups of SNP.
Results
None of the 23 SNPs exerted a statistically significant effect (p<0.0022) on the decline of DAT availability in PD patients. However, we observed trends of association (p<0.05) between three SNPs of two genes with the annual percentage change in DAT availability: 1) rs199347 on the putamen (p=0.0138), 2) rs356181 on the caudate nucleus (p=0.0105), and 3) rs3910105 on the caudate nucleus (p=0.0374). A post-hoc analysis revealed that DAT availability was reduced the most for 1) the putamen in the CC genotype of rs199347 (vs. CT, p=0.0199; vs. TT, p=0.0164), 2) the caudate nucleus in the TT genotype of rs356181 (vs. CC, p=0.0081), and 3) the caudate nucleus in the CC genotype of rs3910105 (vs. TT, p=0.0317).
Parkinson's disease (PD), which is characterized by motor symptoms such as rigidity, hypokinesia, and tremor, is a neurological disorder caused by the loss of dopaminergic neurons in the substantia nigra that results in a decreased dopamine level in the striatum.1 Moreover, the dopamine transporter (DAT), which is present on the presynaptic dopaminergic nerve terminal and controls dopamine level via the reuptake of dopamine in the synaptic cleft, is decreased with dopaminergic neurodegeneration of nigrostriatal neurons.23 Since the uptake of 123I-ioflupane (123I-FP-CIT) in the striatum is correlated with DAT density, 123I-FP-CIT can be used to differentiate Parkinsonian syndromes with and without presynaptic dopaminergic loss.34 A systematic review found that DAT imaging had a sensitivity of 100% in patients with a clinical or neuropathological diagnosis of PD.5 It is well known that the DAT uptake of the striatum as measured by DAT imaging is correlated with the severity and duration of PD.67 The annual reduction rate of striatal DAT uptake is between 6% and 13% in PD.7
PD was previously considered a nongenetic disease caused by environmental factors such as viral infection or neurotoxins.8 However, following Polymeropoulos suggesting a genetic component of PD in 1997, various studies were conducted to identify the genetic factors affecting the etiology of PD using whole-exome sequencing and genome-wide association studies (GWAS).8 Mutations causing PD have now been identified in 15 genes, including SNCA, LRRK2, and MAPT.8
Few studies have evaluated the correlation between genetic mutations and features of DAT imaging in PD patients. McNeill et al.9 used 123I-FP-CIT single-photon-emission computed tomography (SPECT) to reveal that a striatal asymmetry index was higher in PD patients with GBA or LRRK2 than in those with PARKIN, PINK2, and SNCA. Huertas et al.10 reported that the striatal DAT availability as measured by 123I-FP-CIT SPECT in PD patients was associated with SNCA rs356219 and deleterious variants in GBA. However, to the best of our knowledge, no studies have evaluated the correlation between genetic mutations known to be risk factors in PD and chronological changes in striatal DAT availability in PD patients. The present study aimed to determine the association between the annual changes in DAT availability and single-nucleotide polymorphisms (SNPs) known to be risk factors in PD, in order to identify the prognostic value of genetic mutations in PD patients.
The data used in this study were obtained from the Parkinson's Progression Markers Initiative (PPMI) database (www.ppmi-info.org/data); up-to-date information about the study is available at www.ppmi-info.org.11 Males and females aged 30 years or older at screening were included. At baseline, patients with a PD diagnosis within the past 2 years, a Hoehn and Yahr stage not higher than II, a striatal dopamine deficit in 123I-FP-CIT SPECT, and who had not received treatment were included according to the PPMI criteria of PD groups. The following exclusion criteria are applied to the PPMI database: a clinical diagnosis of dementia, taking drugs within the previous 6 months that might interfere with 123I-FP-CIT SPECT, currently taking anticoagulants, having any other medical or psychiatric condition or laboratory abnormality, having taken investigational drugs or devices within 60 days prior to baseline, or showing evidence of clinically significant neurological disorders in magnetic resonance imaging. This study included PD patients who received two 123I-FP-CIT SPECT scans at both the initial screening and 12-month follow-up. The medical history, genotyping results, and 123I-FP-CIT SPECT scans were downloaded. The PPMI study was approved by the local Institutional Review Boards of all participating sites: Institute for Neurodegenerative Disorders, University of Pennsylvania, University of California, Los Angeles, Coriell Institute for Medical Research, Clinical Trials Coordination Center, Laboratory of Neurogenetics, National Institute on Aging National Institutes of Health, Institute for Neurodegenerative Disorders, Clinical Trials Statistical and Data Management Center, University of Iowa. Written informed consent was obtained from each subject at the time of enrollment for imaging data and clinical questionnaires. All of the study methods were performed in accordance with the relevant guidelines and regulations.
123I-FP-CIT SPECT was performed during the screening visit for all subjects. SPECT scans were acquired 4.0±0.5 hr (mean±SD) after injecting 111–185 MBq 123I-FP-CIT. Subjects were pretreated with iodine solution or perchlorate prior to the injection in order to block uptake by the thyroid. Raw data were acquired into a 128×128 matrix, in steps of 3 or 4 degrees for the total projections. Raw projection data were reconstructed using iterative ordered subset expectation maximization with HERMES software (Hermes Medical Solutions, Stockholm, Sweden). The reconstructed images were transferred to PMOD software (PMOD Technologies, Zürich, Switzerland) for subsequent processing, including attenuation correction.
Downloaded scans were loaded using PMOD (version 3.6, PMOD Technologies) with the 123I-FP-CIT template.12 The specific binding of 123I-FP-CIT regarding the DAT was calculated using a region-of-interest analysis. Standard sets of a volume of interest (VOI) defining the caudate nucleus and putamen based on the Automated Anatomical Labeling atlas13 were used. The cerebellum was chosen as a reference region. The VOI template was applied to calculate specific binding ratios (SBRs) of the caudate nucleus and putamen as (target–cerebellum)/cerebellum. The annual percentage change in DAT availability was calculated as [(SBR at 12-month follow-up)–(SBR at screening)]/(SBR at screening)×100/[(interval between 12-month follow-up and screening, in days)/365].
All samples were genotyped using the Illumina NeuroX array following the manufacturer's protocol (Illumina, San Diego, CA, USA). The Genotyping Analysis Module within Genome Studio (version 1.9.4; https://support.illumina.com) was used to analyze data. The genotype frequencies of the SNPs were in Hardy-Weinberg equilibrium (>0.05). SNPs were removed if the minor allele frequency was less than 0.05. Twenty-three SNPs met the inclusion criteria for this study.
To compare clinical features according to three subgroups of SNP, we used the results of the Movement-Disorder-Society-sponsored Unified Parkinson's Disease Rating Scale (MDS-UPDRS) parts II and III. The MDS-UPDRS score was obtained at the time of screening.
Normality was examined using D'Agostino-Pearson omnibus test. The paired t-test and the Wilcoxon test were used to compare differences between SBRs at screening and at the 12-month follow-up and in the annual percentage changes in the caudate nucleus and putamen. The Kruskal-Wallis test was used to compare the annual percentage changes in DAT availability for three subgroups of SNP. Since 23 SNPs met the inclusion criteria for this study, significance was set at α=0.05/23 (0.0022) to correct for multiple comparisons. Post-hoc analysis was performed for pairwise comparisons of subgroups using Dunn's test. The Kruskal-Wallis test was used to analyze the difference of the MDS-UPDRS score according to three subgroups of SNPs. Statistical analyses were performed using GraphPad Prism 7 for Mac OS X (GraphPad Software, San Diego, CA, USA), and MedCalc software package (version 12.6.0.0, MedCalc, Ostend, Belgium).
This study included 150 PD patients (101 males and 49 females). The genotype and allele frequencies of the 23 SNPs in the PPMI database that met the inclusion criteria for this study are listed in Table 1. The SBRs of the caudate nucleus and putamen were 1.21±0.30 and 1.18±0.28, respectively, at screening, and 1.12±0.27 and 1.08±0.27 at the 12-month follow-up, thereby showing significant reductions for both structures (both p<0.0001) (Fig. 1). The mean interval between screening and the 12-month follow-up was 391.3 days. The annual percentage reduction in DAT availability was greater for the putamen (7.18%) than for the caudate nucleus (5.27%) (p=0.0417). The characteristics of the included subjects are summarized in Table 2.
None of the 23 SNPs exerted a statistically significant effect (p<0.0022) on the decline of DAT availability in PD patients. However, we observed trends of association (p<0.05) between three SNPs of two genes in terms of the annual percentage changes in DAT availability: 1) rs199347 on the putamen (p=0.0138), 2) rs356181 on the caudate nucleus (p=0.0105), and 3) rs3910105 on the caudate nucleus (p=0.0374). A post-hoc analysis revealed that DAT availability was reduced the most for 1) the putamen in the CC genotype of rs199347 (vs. CT, p=0.0199; vs. TT, p=0.0164), 2) the caudate nucleus in the TT genotype of rs356181 (vs. CC, p=0.0081), and 3) the caudate nucleus in the CC genotype of rs3910105 (vs. TT, p=0.0317) (Fig. 2). However, the MDS-UPDRS score did not differ between the rs199347, rs356181, and rs3910105 SNPs.
SNPs are variations in the positions of single nucleotides, and they have a frequency of at least 1% in the human genome.14 An SNP can exert its affects at the transcriptional, translational, and posttranslational levels to result in alterations to gene expression and protein stability.15 GWAS have identified numerous SNPs associated with cancer, infection, diabetes, and autoimmune, cardiovascular, gastrointestinal, and neuropsychiatric diseases.15 Polymorphisms in the SLC6A3 gene encoding DAT have been associated with DAT availability, and the number of tandem 9-repeats in the 3′ untranslated region of the gene was associated with greater striatal DAT availability than 10-repeats in normal subjects.16 The rs2652511 and re2937639 SNPs in the SLC6A3 gene were not associated with DAT availability.16 However, the rs2652510 SNP with genotype GG in the SLC6A3 gene was associated with the risk of PD.17
In the present study we demonstrated that the mean SBRs of the caudate nucleus and putamen differed significantly between at screening and at the 12-month follow-up. The annual reduction rate was 7.18% in the putamen and 5.27% in the caudate nucleus, which is consistent with previous findings.7 No SNP significantly affected the decline of DAT availability in PD patients. However, association trends were observed in rs199347, rs356181, and rs3910105.
Nalls et al.18 found that SNP rs199347 on chromosome 7, which was observed in the GPNMB gene, was correlated with PD in Caucasians. They demonstrated that rs199347 was associated with both methylation and expression changes in brain regions, and also showed that the risk allele (A) at rs199347 on chromosome 7 was associated with an increased expression of NUPL2 as well as with decreased methylation of GPNMB in brain regions.18 Those authors claimed that the risk at the locus containing rs199347 might be due to increased transcription of NUPL2 and also be further bolstered by decreased methylation. Murthy et al.19 also reported that rs199347 with major allele AA increased the expression of GPNMB in the brain cortex and putamen in PD patients. Among human brain cell types, the expression of GPNMB was highest in oligodendrocytes, followed by microglia and astrocytes.19 A recent study demonstrated that GPNMB attenuates the astrocyte inflammatory response in rodents.20 Neuroinflammation mediated by activated glial cells is a common feature of PD,21 which suggests that the progression of PD via the activation of glial cells is attenuated by the increased expression of GPNMB through rs199347 with major allele AA. These results imply that the annual reduction rate of DAT availability was reduced the most at allele CC and the least at allele AA. However, rs199347 was not associated with PD in a Taiwanese population22 or with PD in East Asians including residents of Singapore, Hong Kong, Malaysia, Korea, mainland China, and Taiwan.23 The present study utilized the PPMI database, the enrolled patients of various ethnicities, and so the correlation between changes in DAT availability and rs199347 might have been underestimated. A large population study of Caucasians should be performed to validate the protective effect of rs199347 with allele AA of the GPNMB gene in the progression of PD.
SNPs rs356181 and rs3910105 on chromosome 4 were observed in the SNCA gene, which encodes α-synuclein,2425 α-Synuclein consists of 140 amino acid residues and is implicated in the etiology of neurodegenerative diseases such as Alzheimer's disease, PD, dementia with Lewy bodies, and multiple-system atrophy.26 α-Synuclein is also considered to contribute to oligomers that are believed to play a key role in cell death and to be major components of Lewy bodies.26 Increased levels of SNCA mRNA have been reported in the midbrain and substantia nigra of PD patients compared with normal controls.27 However, a study evaluating the association between cerebrospinal fluid biomarkers including for α-synuclein and rs356181 and rs3910105 genotypes found no association between them.25
The SNCA gene has two CpG islands: 1) CpG-1 (located in the first exon) and 2) CpG-2 (located in the first intron).28 The methylation status of a CpG island is known to regulate SNCA in PD patients, and these islands are hypomethylated in PD patients compared with normal controls.28 However, another study found no correlation between hypomethylation of the SNCA-intron 1 and the α-synuclein level.29 In contrast, the risk alleles of both rs356181 and rs3910105 were associated with greater DNA methylation of the CpG island of the SNCA gene.30 Similarly, DNA methylation of the CpG island of the SNCA gene and SNPs rs356181 and rs3910105 appeared to be related with PD progression in the present study.
To the best of our knowledge, this is the first study to have investigated the correlation between genetic mutations and chronological changes in DAT availability. However, this study was subject to some limitations: 1) DAT availability could be affected by dopaminergic medications, 2) the reduction in striatal DAT availability might not be continuous, 3) the retrospective design makes selection bias inevitable, 4) the enrolled patients had various ethnicities that would affect the SNPs, and 5) the number of enrolled patients was small.
In conclusion, no SNP showed a statistically significant effect on the decline of DAT availability in PD patients. However, we identified association trends: 1) rs199347 on the putamen, 2) rs356181 on the caudate nucleus, and 3) rs3910105 on the caudate nucleus. These findings indicate that the rs 199347, rs356181, and rs3910105 SNPs might affect the progression of PD.
Acknowledgements
This study was supported by Biomedical Research Institute Grant (2018 B007), Pusan National University Hospital.
PPMI–a public-private partnership–is funded by the Michael J. Fox Foundation for Parkinson's Research and funding partners, including abbVie, Avid, Biogen, Bristol-Myers Squibb, COVANCE, GE Healthcare, Genentech, GlaxoSmithKline, Lundbeck, Lilly, Merck, MesoScaleDiscovery, Pfizer, Piramal, Roche, Sanofi Genzyme, Servier, TEVA, and UCB.
All procedures performed in studies involving human participants were in accordance with the ethical standards of the institutional and/or national research committee and with the 1964 Helsinki declaration and its later amendments or comparable ethical standards.
References
1. Lotharius J, Brundin P. Pathogenesis of Parkinson's disease: dopamine, vesicles and alpha-synuclein. Nat Rev Neurosci. 2002; 3:932–942. PMID: 12461550.
2. Thomas AJ, Attems J, Colloby SJ, O'Brien JT, McKeith I, Walker R, et al. Autopsy validation of 123I-FP-CIT dopaminergic neuroimaging for the diagnosis of DLB. Neurology. 2017; 88:276–283. PMID: 27940650.
3. Park E. A new era of clinical dopamine transporter imaging using 123I-FP-CIT. J Nucl Med Technol. 2012; 40:222–228. PMID: 23160562.
4. Djang DS, Janssen MJ, Bohnen N, Booij J, Henderson TA, Herholz K, et al. SNM practice guideline for dopamine transporter imaging with 123I-ioflupane SPECT 1.0. J Nucl Med. 2012; 53:154–163. PMID: 22159160.
5. Suwijn SR, van Boheemen CJ, de Haan RJ, Tissingh G, Booij J, de Bie RM. The diagnostic accuracy of dopamine transporter SPECT imaging to detect nigrostriatal cell loss in patients with Parkinson's disease or clinically uncertain parkinsonism: a systematic review. EJNMMI Res. 2015; 5:12. PMID: 25853018.


6. Benamer HT, Patterson J, Wyper DJ, Hadley DM, Macphee GJ, Grosset DG. Correlation of Parkinson's disease severity and duration with 123I-FP-CIT SPECT striatal uptake. Mov Disord. 2000; 15:692–698. PMID: 10928580.
7. Kägi G, Bhatia KP, Tolosa E. The role of DAT-SPECT in movement disorders. J Neurol Neurosurg Psychiatry. 2010; 81:5–12. PMID: 20019219.
8. Billingsley KJ, Bandres-Ciga S, Saez-Atienzar S, Singleton AB. Genetic risk factors in Parkinson's disease. Cell Tissue Res. 2018; 373:9–20. PMID: 29536161.


9. McNeill A, Wu RM, Tzen KY, Aguiar PC, Arbelo JM, Barone P, et al. Dopaminergic neuronal imaging in genetic Parkinson's disease: insights into pathogenesis. PLoS One. 2013; 8:e69190. PMID: 23935950.


10. Huertas I, Jesús S, García-Gómez FJ, Lojo JA, Bernal-Bernal I, Bonilla-Toribio M, et al. Genetic factors influencing frontostriatal dysfunction and the development of dementia in Parkinson's disease. PLoS One. 2017; 12:e0175560. PMID: 28399184.


11. Parkinson Progression. The parkinson progression marker initiative (PPMI). Prog Neurobiol. 2011; 95:629–635. PMID: 21930184.
12. García-Gómez FJ, García-Solís D, Luis-Simón FJ, Marín-Oyaga VA, Carrillo F, Mir P, et al. Elaboration of the SPM template for the standardization of SPECT images with 123I-Ioflupane. Rev Esp Med Nucl Imagen Mol. 2013; 32:350–356. PMID: 23570700.
13. Tzourio-Mazoyer N, Landeau B, Papathanassiou D, Crivello F, Etard O, Delcroix N, et al. Automated anatomical labeling of activations in SPM using a macroscopic anatomical parcellation of the MNI MRI single-subject brain. Neuroimage. 2002; 15:273–289. PMID: 11771995.


14. Squire LR. Encyclopedia of Neuroscience. Boston: Elsevier Academic Press;2009.
15. Fareed M, Afzal M. Single nucleotide polymorphism in genome-wide association of human population: a tool for broad spectrum service. Egypt J Med Hum Genet. 2013; 14:123–134.


16. van de Giessen E, de Win MM, Tanck MW, van den Brink W, Baas F, Booij J. Striatal dopamine transporter availability associated with polymorphisms in the dopamine transporter gene SLC6A3. J Nucl Med. 2009; 50:45–52. PMID: 19091889.


17. Zhai D, Li S, Zhao Y, Lin Z. SLC6A3 is a risk factor for Parkinson's disease: a meta-analysis of sixteen years' studies. Neurosci Lett. 2014; 564:99–104. PMID: 24211691.


18. Nalls MA, Pankratz N, Lill CM, Do CB, Hernandez DG, Saad M, et al. Large-scale meta-analysis of genome-wide association data identifies six new risk loci for Parkinson's disease. Nat Genet. 2014; 46:989–993. PMID: 25064009.
19. Murthy MN, Blauwendraat C, Guelfi S, Hardy J, Lewis PA, Trabzuni D. Increased brain expression of GPNMB is associated with genome wide significant risk for Parkinson's disease on chromosome 7p15.3. Neurogenetics. 2017; 18:121–133. PMID: 28391543.


20. Neal ML, Boyle AM, Budge KM, Safadi FF, Richardson JR. The glycoprotein GPNMB attenuates astrocyte inflammatory responses through the CD44 receptor. J Neuroinflammation. 2018; 15:73. PMID: 29519253.


21. Wang Q, Liu Y, Zhou J. Neuroinflammation in Parkinson's disease and its potential as therapeutic target. Transl Neurodegener. 2015; 4:19. PMID: 26464797.


22. Wu HC, Chen CM, Chen YC, Fung HC, Chang KH, Wu YR. DLG2, but not TMEM229B, GPNMB, and ITGA8 polymorphism, is associated with Parkinson's disease in a Taiwanese population. Neurobiol Aging. 2018; 64:158.e1–158.e6.


23. Foo JN, Tan LC, Irwan ID, Au WL, Low HQ, Prakash KM, et al. Genome-wide association study of Parkinson's disease in East Asians. Hum Mol Genet. 2017; 26:226–232. PMID: 28011712.


24. Mata IF, Shi M, Agarwal P, Chung KA, Edwards KL, Factor SA, et al. SNCA variant associated with Parkinson disease and plasma alpha-synuclein level. Arch Neurol. 2010; 67:1350–1356. PMID: 21060011.


25. Kang JH, Mollenhauer B, Coffey CS, Toledo JB, Weintraub D, Galasko DR, et al. CSF biomarkers associated with disease heterogeneity in early Parkinson's disease: the Parkinson's Progression Markers Initiative study. Acta Neuropathol. 2016; 131:935–949. PMID: 27021906.


26. Xu W, Tan L, Yu JT. Link between the SNCA gene and parkinsonism. Neurobiol Aging. 2015; 36:1505–1518. PMID: 25554495.
27. Tagliafierro L, Chiba-Falek O. Up-regulation of SNCA gene expression: implications to synucleinopathies. Neurogenetics. 2016; 17:145–157. PMID: 26948950.


28. Miranda-Morales E, Meier K, Sandoval-Carrillo A, Salas-Pacheco J, Vázquez-Cárdenas P, Arias-Carrión O. Implications of DNA methylation in Parkinson's disease. Front Mol Neurosci. 2017; 10:225. PMID: 28769760.


29. Guhathakurta S, Evangelista BA, Ghosh S, Basu S, Kim YS. Hypomethylation of intron1 of α-synuclein gene does not correlate with Parkinson's disease. Mol Brain. 2017; 10:6. PMID: 28173842.


30. Hernandez DM. Genetic variation and DNA methylation in the context of neurological disease [dissertation]. London: University College London;2016.
Fig. 1
Serial changes in the SBR between screening and 12-month follow-up in both the caudate nucleus and putamen (*p<0.0001; data are means with 95% CIs). SBR: specific binding ratio.
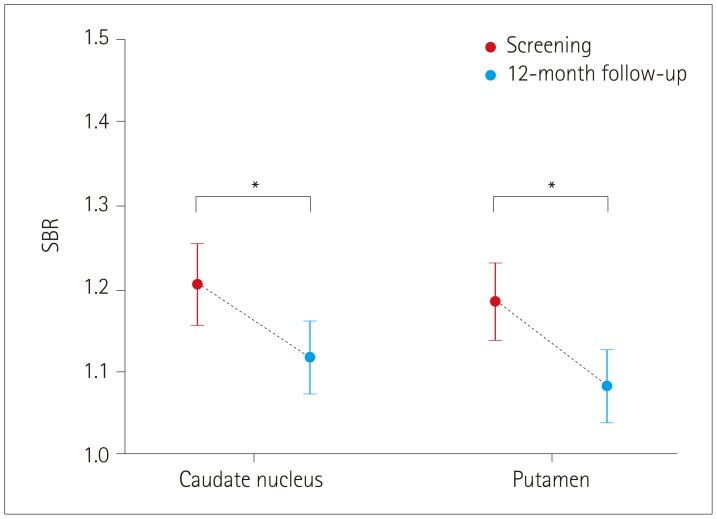
Fig. 2
Effects of single-nucleotide polymorphisms on the annual percentage change in DAT availability: rs199347 on the putamen (A), rs356181 on the caudate nucleus (B), and rs3910105 on the caudate nucleus (C) (*p<0.05, †p<0.01; data are means with 95% CIs). DAT: dopamine transporter.
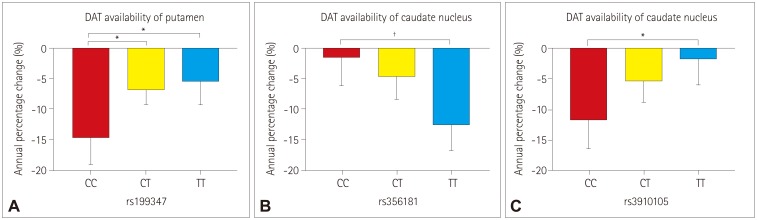
Table 1
Genotype frequencies for alleles
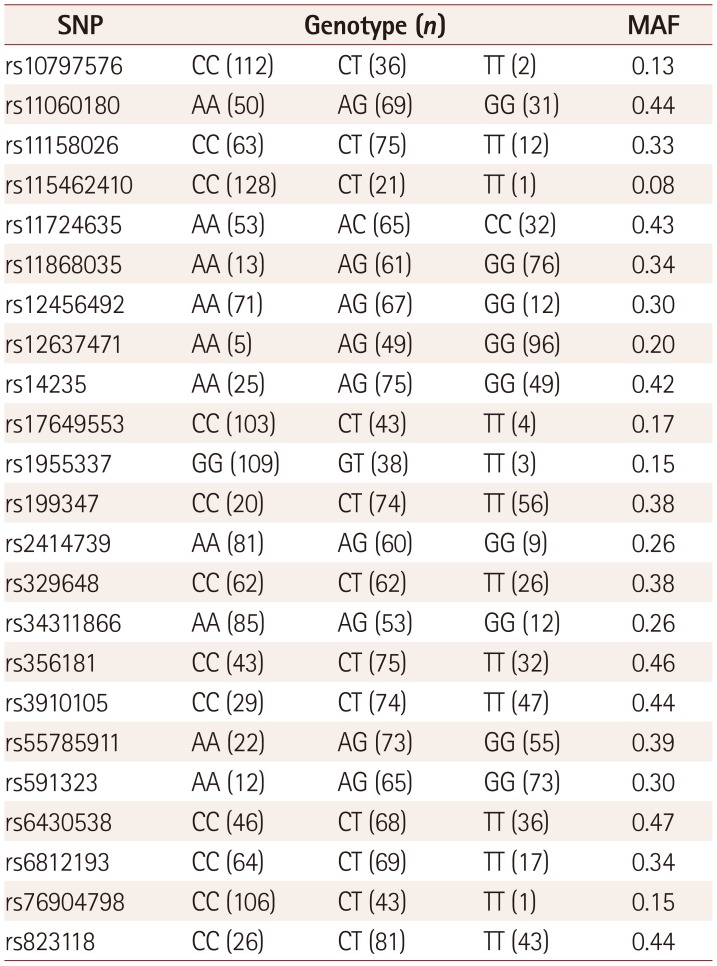
Table 2
Characteristics of the study subjects
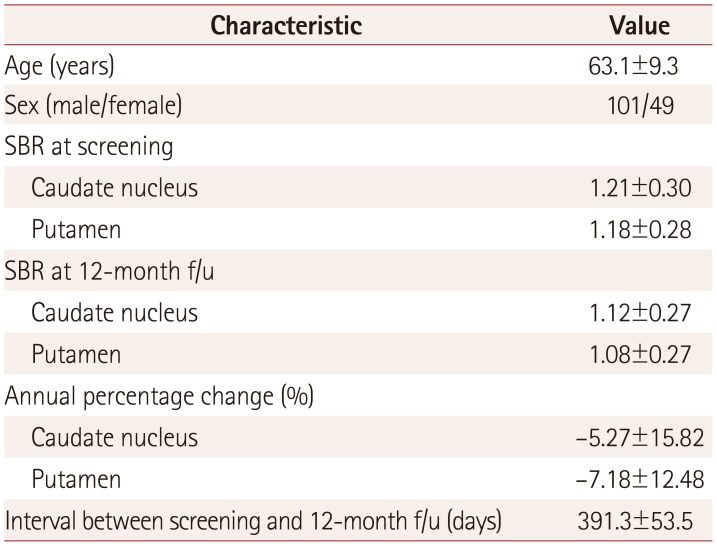