Abstract
Objectives
As a first step to study the anticaries effect of ethanol alone, we investigated the effects of ethanol on the expression levels of the atpB gene and proton permeability of Streptococcus mutans in suspension cultures.
Methods
S. mutans UA159 was grown in brain heart infusion medium at either pH 4.8 or 6.8. The total extracted RNA was reverse-transcribed into cDNA using a Superscript™ First-Strand Synthesis System. The resulting cDNA and negative controls were amplified by ABI PRISM 7700 real-time PCR system with SYBR Green PCR Master Mix. For proton flux assay, bacterial suspensions were titrated to pH 4.6 with 0.5 M HCl, and then additional 0.5 M HCl was added to decrease the pH values by approximately 0.4 units. The subsequent increase in pH was monitored using a glass electrode. Ten percent (v/v) butanol was added to the suspensions at 80 min to disrupt the cell membrane.
Dental caries is still a major oral health problem even in developed countries. Although 200-300 bacterial species have been found associated with dental biofilm, especially the presence of Streptococcus mutans (S. mutans) has been consistently linked with the formation of human dental caries1). Recent studies have shown that a large proportion of the S. mutans genome is required for acid tolerance234), however, the membrane-bound, proton-pumping F-ATPase is generally regarded as one of the key components of the acid tolerance response56789).
Proton permeability is an important factor permitting cells to maintain the proton-motive force (PMF) for vital energy transducing processes. PMF is essential for S. mutans activity. In addition to its high-affinity phosphotransferase sugar transport system, S. mutans has the second, low-affinity sugar uptake system, which is activated by PMF10). This is why S. mutans can survive under acidic conditions which are unfavorable to other bacteria.
Ethanol has long been used as a solvent for a number of cariostatic natural products. It is well known that ethanol attacks nucleic acids, lipid bilayers, and proteins of the cell and impairs its multiple functions in bacteria11). Even though it is highly probable that ethanol itself may affect the viability of S. mutans, no experiments have yet been performed to elucidate the anticaries effect of ethanol alone.
As a first step to study the anticaries effects of ethanol itself, we investigated the effects of ethanol on the expression levels of atpB gene of S. mutans in suspension cultures. Also, we checked the effects of ethanol on the proton permeability of planktonic S. mutans cells.
S. mutans UA159 (#700610) was purchased from ATCC (Manassas, VA, USA) and Brain Heart Infusion (BHI) medium from Becton, Dickinson & Company (Franklin, NJ, USA). All other chemicals were of the reagent grade.
Planktonic cells of S. mutans were grown in 50 ml BHI medium for 16 h at 37℃. The culture was used to inoculate 1 L flasks of prewarmed BHI medium and incubated at 37℃ until an absorbance reading of 0.7 at 600 nm was reached. The cultivation was done at either pH 4.8 or 6.8. During cultivation, the bacteria exhibited a tendency to acidify the cultivation medium. So, the pH value was continuously monitored and adjusted by a stepwise addition of 500 mM KOH, if necessary. The growth curves were measured every 15 minutes. The growth rates (c) were calculated as c=Δlog2(OD420)/Δt where OD420 is the optical density at 420 nm and t is time12).
Cells were harvested via centrifugation, resuspended and incubated in RNAProtect Bacteria Reagent at room temperature, and then centrifuged at 4,000×g for 10 min. The pellets were resuspended in 200 µl lysis buffer containing 20 mM Tris-HCl (pH 8.0), 20 mg/ml lysozyme, 1000 U/ml mutanolysin, and 60 mAU/ml proteinase k. They were incubated at 37℃ for 45 min, followed by two cycles of sonication for 15 sec twice.
RNA extractions were carried out with the RNeasy mini kit according to the manufacturer's instructions. RNA was quantified spectrophotometrically at A260 for concentration and at A260/A280 for purity.
Total intact RNA obtained from S. mutans UA159 was reverse-transcribed into cDNA using a Superscript™ First-Strand Synthesis System for RT-PCR according to the manufacturer's instructions.
The resulting cDNA and negative controls were amplified by ABI PRISM 7700 real-time PCR system with SYBR Green PCR Master Mix (Applied Biosystems, Foster City, CA, USA). The primers were: 5′-AGGCCGACTTTACCACCTTT-3′(forward) and 5′-TCCGGTTGGAAAAGAAACAC-3′(reverse) and 5′-AAGCAACGCGAAGAACCTTA-3′ (forward) and 5′-GTCTCGCTAGAGTGCCCAAC-3′(reverse) for 16S rRNA. Each of the primers was designed using the Primer3 program
(version 0.4.0; http://frodo.wi.mit.edu/primer3/).
Each reaction tube contained 20 µl of reaction mixture, including 1X SYBR Green PCR Master Mix, cDNA, template, and 2 µl of 10 pM forward and reverse primers. The cycle profile was as follows: 1 cycle at 50℃ for 5 min and initial denaturation at 95℃ for 10 min, followed by 40 cycle amplification consisting of denaturation at 95℃ for 15 s, annealing at 54℃ for 30 s, and extension at 72℃ for 30 s.
The relative expression was calculated by normalizing the mRNA of the atpB gene with that of the 16S rRNA gene, which served as the reference gene.
The proton permeability was assessed using the procedure described previously1314). Cells were harvested by centrifugation and washed in 5 mM MgCl2. They were suspended at a cell density of 5 mg/ml in 20 mM potassium phosphate buffer (either pH 4.8 or 6.8) for 1 h to deplete the endogenous catabolites. The cells were centrifuged again and suspended at a cell density of 25 mg/ml in 50 mM KCl+1 mM MgCl2. The suspensions were then titrated to pH 4.6 with 0.5 M HCl until the pH value stabilized, as indicated by no pH change for at least 2 min. Then 0.5 M HCl was added to decrease the pH values in approximately 0.4 units. The subsequent increase in pH associated with proton movements across the cell membrane into the cytoplasm was monitored using a glass electrode. 10 % (v/v) butanol was added to the suspensions at 80 min to disrupt the cell membrane.
The data are presented as the mean± standard error of the mean (SEM). Student's t-test was performed to determine the significant difference. Univariate analysis of variance (ANCOVA) was conducted to test the effect of ethanol. Values were considered statistically significant when P value was <0.05. The statistical analyses were performed using IBM SPSS Statistics 23 (SPSS Inc., Chicago, IL, USA).
First, we measured the growth rates of bacteria cultured at either pH 4.8 or 6.8. Compared to pH 6.8, much slower growth rates were obtained at pH 4.8, especially in the presence of ethanol (Fig. 1 and Table 1). At pH 4.8, the growth rate of 0.006 min−1 was reduced to 0.002 min−1 and the doubling time (T) of 169 min increased to 417 min in the presence of 500 mM ethanol.
The F-ATPase operon promoter1516) and atp genes217) of S. mutans were transcriptionally upregulated at acidic pH. At pH 4.8, the expression level of the atpB gene was about 20% higher than at pH 6.8. At both pHs, ethanol inhibited the atpB gene expression in a concentration-dependent manner (Fig. 2). Though significant decreases in the expression levels of the atpB gene were obtained at relatively high concentrations of ethanol, we could observe 26% and 22% decreases at pHs 4.8 and 6.8, respectively, even at 10 mM.
Fig. 3 shows that, following the initial pH pulse, the pH of the cell suspension started to gradually increase as a consequence of cellular proton uptake. Ethanol dose-dependently increased proton permeability at both pHs (Fig. 3 and Table 2). We assume that the decreased proton efflux due to the decreased expression of the atpB gene may be one of the causes of an increase in the proton permeability.
In this research, we demonstrated the possibility of ethanol as an active ingredient that may have the anticaries effect. In a concentration-dependent manner, ethanol alone not only decreased the growth rate of S. mutans and the expression of the atpB gene but also increased the proton permeability at both pHs 4.8 and 6.8. The increase in proton permeability seems to be due, at least in part, to reduced expression of F-ATPase.
We have previously reported that 10 mM ethanol enhanced the stimulatory action of fluoride on proton permeability as well as the inhibitory action of fluoride on the F-ATPase activity only at pH 4.8 in S. mutans18). In this study, the anti-S. mutans property of ethanol alone started at 100 mM, a 10-fold higher concentration. Ethanol has been added to mouthrinses as an inactive ingredient that acts as a carrier for the flavor. Some mouthrinses on the market have more than 20%(v/v) alcohol, which is equal to 3.45 M, in them. At this high concentration, it is almost certain that some of the anticaries effects of the mouthrinses may have originated from ethanol itself. In addition, it is very likely that the cariostatic effects of a number of naturally occurring compounds may have been exaggerated because ethanol has long been used as a solvent for dissolving and/or efficient extraction of them. It is time to consider ethanol as an active ingredient instead of an inactive carrier.
Although fluoride has been unparalleled as an anticaries agent, it is generally accepted that the effectiveness of fluoride could be enhanced when combined with additional cariostatic agents. We believe that better cariostatic preparations may be developed if we use existing agents together with new agents whose mechanisms of action differ from those of fluoride and other anticaries agents. Ethanol is a well-known membrane perturbing agent. Ethanol can induce membrane expansion, accompanied by a decrease in the membrane thickness as well as disordering and enhanced interdigitation of lipid acyl chains19). We suggested that cytoplasmic membrane fluidity is one of the crucial factors that determine proton permeability in S. mutans20). Further experimentation is required to study the membrane effects of ethanol in S. mutans. In addition, we used S. mutans in suspensions. We expect that future experiments using S. mutans in biofilms will confirm the results of the present study.
Ethanol is a man's oldest social beverage-drug. Its beneficial and harmful effects have been with mankind for millennia. Ethanol has been appreciated, craved, respected, abhorred, and feared, but it has never been thoroughly understood. Of course ethanol containing products should not be recommended to children for long term use or to individuals with alcohol problems21). However, for external use only, ethanol has a number of advantages. It is user-friendly, nonallergenic, nontoxic, only minimally irritant, non-aggressive or corrosive22). If ethanol is proved to be an active anticaries ingredient, we may be able to not only reduce the effective concentrations of fluoride and/or other cariostatic agents but also enhance their anticaries activity.
Our findings suggest that ethanol has the potential for an active anticaries ingredient instead of an inactive carrier for the flavor. We expect that ethanol may be used together with fluoride and/or other anticaries agents in order to develop better anticaries toothpastes and/or mouthrinses.
Figures and Tables
Fig. 1
Growth curves of S. mutans UA159. The growth rates (c) were calculated as c=Δlog2(OD420)/Δt where OD420 is the optical density at 420 nm, and t is time. Data are reported as the mean±SEM of triplicate analysis of three preparations.
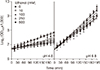
Fig. 2
The relative mRNA levels for the atpB gene of S. mutans UA159. The relative values were obtained by normalizing the atpB mRNA with expression of 16S rRNA gene. The Data are reported as mean±SEM of triplicate analysis of three preparations. *P<0.05, **P<0.01, ***P<0.001.
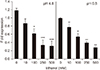
Fig. 3
Effects of ethanol on the proton permeability in S. mutans UA159. Data are reported as the mean±SEM of triplicate analysis of three preparations.
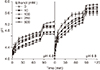
Table 2
Effects of ethanol on the proton permeability in S. mutans UA159a
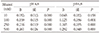
aUnivariate analysis of variance (ANCOVA) adjusted for time was performed to calculate the probability using the data in Fig. 3. 0 mM ethanol served as the control.
References
1. Loesche WJ. Role of Streptococcus mutans in human dental decay. Microbiol Rev. 1986; 50(4):353–380.
2. Baker JL, Abranches J, Faustoferri RC, Hubbard CJ, Lemos JA, Courtney MA, et al. Transcriptional profile of glucose-shocked and acid-adapted strains of Streptococcus mutans. Mol Oral Microbiol. 2015; 30(6):496–517.


3. Baker JL, Faustoferri RC, Quivey RG. Acid-adaptive mechanisms of Streptococcus mutans-the more we know, the more we don't. Mol Oral Microbiol. 2017; 32(2):107–117.


4. Quivey RG, Grayhack EJ, Faustoferri RC, Hubbard CJ, Baldeck JD, Wolf AS, et al. Functional profiling in Streptococcus mutans: construction and examination of a genomic collection of gene deletion mutants. Mol Oral Microbiol. 2015; 30(6):474–495.


5. Belli WA, Marquis RE. Adaptation of Streptococcus mutans and Enterococcus hirae to acid stress in conuous culture. Appl Environ Microbiol. 1991; 57(4):1134–1138.


6. Bender GR, Sutton SV, Marquis RE. Acid tolerance, proton permeabilities, and membrane ATPases of oral streptococci. Infect Immun. 1986; 53(2):331–338.


7. Hamilton IR, Buckley ND. Adaptation by Streptococcus mutans to acid tolerance. Oral Microbiol Immunol. 1991; 6(2):65–71.


8. Lemos JA, Abranches J, Burne RA. Responses of cariogenic streptococci to environmental stresses. Curr Issues Mol Biol. 2005; 7(1):95–108.
9. Quivey RG Jr, Kuhnert WL, Hahn K. Adaptation of oral streptococci to low pH. Adv Microb Physiol. 2000; 42:239–274.


10. Hamilton IR, St Martin EJ. Evidence for the involvement of proton motive force in the transport of glucose by a mutant of Streptococcus mutans strain DR0001 defective in glucose-phosphoenopyruvate phosphotransferase activity. Infect Immun. 1982; 36(2):567–575.


11. Seydlová G, Halada P, Fišer R, Toman O, Ulrych A, Svobodová J. DnaK and GroEL chaperones are recruited to the Bacillus subtilis membrane after short-term ethanol stress. J Appl Microbiol. 2012; 112(4):765–774.


12. Ban SH, Kim JE, Pandit S, Jeon JG. Influences of Dryopteris crassirhizoma extract on the viability, growth and virulence properties of Streptococcus mutans. Molecules. 2012; 17(8):9231–9244.


13. Petrackova D, Vecer J, Svobodova J, Herman P. Long-term adaptation of Bacillus subtilis 168 to extreme pH affects chemical and physical properties of the cellular membrane. J Membr Biol. 2010; 233(1-3):73–83.


14. Phan TN, Buckner T, Sheng J, Baldeck JD, Marquis RE. Physiologic actions of zinc related to inhibition of acid and alkali production by oral streptococci in suspensions and biofilms. Oral Microbiol Immunol. 2004; 19(1):31–38.


15. Hwang G, Liu Y, Kim D, Sun V, Aviles-Reyes A, Kajfasz JK, et al. Simultaneous spatiotemporal mapping of in situ pH and bacterial activity within an intact 3D microcolony structure. Sci Rep. 2016; 09. 08. DOI: 10.1038/srep32841. [Epub].


16. Kuhnert WL, Zheng G, Faustoferri RC, Quivey RG. The F-ATPase operon promoter of Streptococcus mutans is transcriptionally regulated in response to external pH. J Bacteriol. 2004; 186(24):8524–8528.


17. Gong Y, Tian XL, Sutherland T, Sisson G, Mai J, Ling J, et al. Global transcriptional analysis of acid-inducible genes in Streptococcus mutans: multiple two-component systems involved in acid adaptation. Microbiology. 2009; 155:3322–3332.


18. Lee SA, Jung SI, Kim JB, Kang JS. The pH-dependent effects of combining ethanol with fluoride on proton permeability in Streptococcus mutans. J Korean Acad Oral Health. 2016; 40(4):255–260.


19. Gurtovenko AA, Anwar J. Interaction of ethanol with biological membranes: the formation of non-bilayer structures within the membrane interior and their significance. J Phys Chem B. 2009; 113(7):1983–1992.


20. Cho CM, Jung SI, Kim MS, Lee SA, Kang JS. pH stress alters cytoplasmic membrane fluidity and atpB gene expression in Streptococcus mutans. J Life Sci. 2017; 27(1):15–22.


21. FDI commission. Mouthrinses and dental caries. Int Dent J. 2002; 52(5):337–345.