Abstract
Hyper-O-GlcNAcylation is a general feature of cancer which contributes to various cancer phenotypes, including cell proliferation and cell growth. Quercetin, a naturally occurring dietary flavonoid, has been reported to reduce the proliferation and growth of cancer. Several reports of the anticancer effect of quercetin have been published, but there is no study regarding its effect on O-GlcNAcylation. The aim of this study was to investigate the anticancer effect of quercetin on HeLa cells and compare this with its effect on HaCaT cells. Cell viability and cell death were determined by MTT and terminal deoxynucleotidyl transferase-mediated deoxyuridine triphosphate nick-end labelling assays. O-GlcNAcylation of AMP-activated protein kinase (AMPK) was examined by succinylated wheat germ agglutinin pulldown and immunoprecipitation. Immunofluorescence staining was used to detect the immunoreactivitiy of O-linked N-acetylglucosamine transferase (OGT) and sterol regulatory element binding protein 1 (SREBP-1). Quercetin decreased cell proliferation and induced cell death, but its effect on HaCaT cells was lower than that on HeLa cells. O-GlcNAcylation level was higher in HeLa cells than in HaCaT cells. Quercetin decreased the expression of global O-GlcNAcylation and increased AMPK activation by reducing the O-GlcNAcylation of AMPK. AMPK activation due to reduced O-GlcNAcylation of AMPK was confirmed by treatment with 6-diazo-5-oxo-L-norleucine. Our results also demonstrated that quercetin regulated SREBP-1 and its transcriptional targets. Furthermore, immunofluorescence staining showed that quercetin treatment decreased the immunoreactivities of OGT and SREBP-1 in HeLa cells. Our findings demonstrate that quercetin exhibited its anticancer effect by decreasing the O-GlcNAcylation of AMPK. Further studies are needed to explore how quercetin regulates O-GlcNAcylation in cancer.
O-GlcNAcylation is a post-translational modification consisting of the addition of O-linked N-acetylglucosamine (O-GlcNAc) to serine/threonine residues on proteins [12]. Increased O-GlcNAcylation has been observed in several cancers, and this contributes to cell proliferation, survival, invasion, and metastasis, whereas decreasing O-GlcNAcylation reduces cancer progression [34567]. The identification of pharmacological compounds from natural products that decrease O-GlcNAcylation and have minimal side effects is a potentially attractive approach to developing more effective therapeutic strategies for cancer.
Quercetin, a naturally occurring dietary flavonoid found in various fruits and vegetables, has been reported to decrease the progression of several cancers, including cervical cancer [8910]. Several possible mechanisms of the anticancer effect of quercetin have been proposed [1112], but no study has reported on the effect of quercetin on O-GlcNAcylation. Uridine diphospho-GlcNAc (UDP-GlcNAc), a substrate for O-linked N-acetylglucosamine transferase (OGT) in the process of O-GlcNAcylation, is synthesised from glucose through the hexosamine pathway (HBP). The increase in glucose uptake in cancer cells elevates UDP-GlcNAc through HBP and thus drives cellular hyper-O-GlcNAcylation [1]. Quercetin decreases glucose uptake, which in turn may inhibit the hexosamine pathway, thereby potentially causing decreased O-GlcNAcylation [1314].
AMP-activated protein kinase (AMPK), a central energy sensor, is known as a crucial factor in the interaction between metabolism and cancer. AMPK activation is associated with cellular stressors, including low glucose and decreased ATP levels [1516171819]. Recently, it has been reported that decreased O-GlcNAcylation can activate AMPK and could therefore decrease cancer progression [2021]. Once activated, AMPK regulates various proteins involved in metabolism, which suppress energy consumption and cellular growth, such as sterol regulatory element binding protein 1 (SREBP-1), a protein involved in lipogenesis, which regulates enzymes involved in the synthesis of lipids, such as fatty acid synthase (FAS) and acetyl-CoA carboxylase (ACC) [222324].
AMPK activation has been found to reduce cervical cancer progression [2526]; however, AMPK activation as a result of O-GlcNAcylation in cervical cancer has not been established and needs to be elucidated. Here, we examined the effect of quercetin on global O-GlcNAcylation and activation of AMPK through O-GlcNAcylation in cervical cancer, with the aim of developing novel agents for cancer therapy.
Dulbecco's modified Eagle medium (DMEM), penicillin-streptomycin, and fetal bovine serum (FBS) were obtained from Gibco (Invitrogen, Carlsbad, CA, USA). Dimethyl sulfoxide, 6-diazo-5-oxo-norleucineis (DON), and Thiamet G were purchased from Sigma (St. Louis, MO, USA). Quercetin was purchased from Cayman Chemical (Ann Arbor, MI, USA). Mouse anti-GlcNAc (MA1-072-RL2) was purchased from Thermo Fisher Scientific (Rockford, IL, USA). Antibodies against AMPK-α (#2532), phospho-AMPK-α (#2535), Fas (#3180), ACC (#3662), caspase 3 (#9662), cleaved caspase 3 (#9661), poly(ADP ribose) polymerase (PARP) (#9532), and cleaved PARP (#5625) were obtained from Cell Signaling Technology (Danvers, MA, USA). Antibodies against OGT (sc-74546) and SREBP-1 (sc-8984) were purchased from Santa Cruz Biotechnology (Dallas, TX, USA). Antibody against b-actin (A5441) was obtained from Sigma. Secondary horseradish peroxidase-conjugated goat anti-mouse IgG (#31430) and goat anti-rabbit IgG (#31460) were obtained from Thermo Fisher Scientific.
Human immortalised keratinocyte cells (HaCaT) and cervical cancer cells (HeLa) were obtained from American Type Culture Collection (Manassas, VA, USA). HeLa and HaCaT cells were maintained in high-glucose DMEM with 10% FBS, 100 µg/mL streptomycin, and 100 units/mL penicillin (Invitrogen). Cells were cultured in 5% CO2 at 37℃.
HaCaT and HeLa cells were seeded at a density of 2,000 cells per well in a 96-well plate and incubated for 24 hours. The cells were then treated with quercetin at different concentrations for 24 hours and subjected to the 3-(4,5-dimethylthiazol-2-yl)-2,5-diphenyltetrazolium bromide (MTT) assay. MTT solution was added to each well and the plates were incubated at 37℃ for 2 hours. The formazan crystals formed were dissolved in dimethyl sulphoxide and the absorbance of the solution was measured at 570 nm using a microplate reader (Tecan, Maennedorf, Switzerland).
Cells were lysed in RIPA lysis buffer (25 mM Tris HCl pH 7.6, 150 mM NaCl, 1% NP-40, 1% sodium deoxycholate, 0.1% sodium dodecyl sulfate [SDS]) with protease inhibitor cocktail (Sigma Aldrich). The protein concentration was determined using the bicinchoninic acid protein assay kit (Thermo Scientific). Total protein lysates were separated by sodium dodecyl sulphate polyacrylamide gel electrophoresis (SDS-PAGE) and transferred to nitrocellulose membranes (Millipore, Billerica, MA, USA). The target proteins were detected by western blotting with selected antibodies. Chemiluminescence (ECL, Amersham Biosciences, Piscataway, NJ, USA) was used to detect protein bands. Blot images were captured on a RAS-4000 image reader (Fujifilm, Tokyo, Japan).
Cells were homogenised in RIPA lysis buffer (25 mM Tris HCl pH 7.6, 150 mM NaCl, 1% NP-40, 1% sodium deoxycholate, 0.1% SDS) with protease inhibitor cocktail. Lysates containing 200 µg of protein were incubated with agarose-bound succinylated wheat germ agglutinin (sWGA) beads (Vector Laboratories, Burlingame, CA, USA) overnight at 4℃. On the following day, the samples were centrifuged at 2,000 ×g for 2 minutes. Free GlcNAc was added during sWGA lectin-affinity purification to determine the specificity of the sWGA beads. Precipitates were washed three times with RIPA buffer, and proteins were eluted by boiling in SDS sample buffer. Lysates were then analysed by western blot.
The recommended antibodies were mixed with protein A/G PLUS-Agarose beads (Santa Cruz Biotechnology) and then incubated overnight with rotation at 4℃. The following day, the sample was centrifuged at 12,000 ×g, and the antibody-bead complex was washed and collected by centrifugation. The antibody-bead complex was then mixed with pre-cleared cell lysates and incubated overnight with rotation at 4℃. The lysate-bead/antibody conjugate mixture was then washed and collected by centrifugation. Samples were boiled in loading buffer to remove the agarose beads, and the proteins were then separated by SDS-PAGE on 10% acrylamide gels. Proteins were then transferred to membranes, probed with antibodies against the interacting protein of interest, and processed for western blotting as described above.
Cells were grown on glass cover slips in 24-well culture plates at 2×104 cells per well for 24 hours. Then, the cells were treated with 50 µM of quercetin for 24 hours. Next, cells were washed with phosphate buffered saline (PBS), fixed for 15 minutes at room temperature with 4% paraformaldehyde, and permeabilised for 15 minutes with 0.3% Triton X-100 in PBS for 10 minutes. Then, the cells were blocked with 5% normal donkey serum in PBS for 1 hour. Subsequently, the cells were incubated at 4℃ with the desired primary antibody in 5% normal donkey serum overnight, followed by incubation with a specific fluorescence-conjugated secondary IgG (Invitrogen) for 1 hour in the dark. Finally, cells were washed with PBS and mounted using ProLong Gold antifade mountant with 4′,6-diamidino-2-phenylindole dichloride (DAPI) (Invitrogen) for nuclear staining. All images were taken using a fluorescence microscope (BX51-DSU, Olympus, Tokyo, Japan).
Terminal deoxynucleotidyl transferase-mediated deoxyuridine triphosphate nick-end labelling (TUNEL) staining was performed to detect cells death using the In Situ Cell Death Detection Kit, TMR Red (Roche Molecular Biochemicals, Mannheim, Germany). Cells were grown on glass coverslips in 24-well culture plates at 2×104 cells per well for 24 hours. Next, the cells were treated with 50 µM of quercetin for 24 hours. Cells were washed with PBS, fixed with 4% paraformaldehyde for 15 minutes, and permeabilised for 2 minutes with 0.5% Triton X-100 in PBS on ice. Cells were then washed in PBS and incubated with TUNEL reaction mixture containing terminal deoxynucleotidyl transferase (TdT), and the reaction buffer containing fluorescein-dUTP (Roche Applied Sciences, Mannheim, Germany) for 60 minutes at 37℃. Finally, cells were washed with PBS and mounted using Pro-Long Gold antifade mountant with DAPI for nuclear staining. All images were taken using a fluorescence microscope (BX51-DSU, Olympus).
HaCaT and HeLa cells were treated with various concentrations of quercetin (10 µM, 20 µM, 50 µM, 100 µM, or 200 µM) for 24 hours, and cell viability was determined by the MTT assay. Quercetin decreased cell viability in a dose-dependent manner (Fig. 1A, B). Quercetin was less effective on HaCaT than on HeLa cells at the same concentration (Fig. 1C). The IC50 value of quercetin for HeLa cells was estimated to be 50 µM. Based on these data, we chose to treat the cells with 50 µM of quercetin to study its effect on cervical cancer. Next, we determined the effects of quercetin on the expression levels of apoptotic markers such as caspase 3, cleaved caspase 3, PARP, and cleaved PARP by western blot analysis. Results showed that quercetin increased the expression levels of both cleaved caspase 3 and cleaved PARP, with two-fold higher effects on HeLa cells than on HaCaT cells (Fig. 1D).
We examined OGT and O-GlcNAc expression levels and found that the levels of OGT and O-GlcNAcylation were higher in HeLa cells than in HaCaT cells (Fig. 2A, B). The effect of quercetin on OGT and O-GlcNAc levels was also higher in HeLa cells than in HaCaT cells (Fig. 2A, B). Activation of AMPK has been linked to O-GlcNAcylation, and several studies have reported that a decrease in O-GlcNAcylation activates AMPK [2021]. Therefore, we checked the effect of quercetin on O-GlcNAcylation of AMPK. O-GlcNAcylated proteins were pulled down by sWGA-lectin-affinity, and the proteins were analysed by western blotting against O-GlcNAc and AMPK. We found that HeLa cells have elevated O-GlcNAcylated AMPK levels compared to HaCaT cells (Fig. 2C). However, quercetin decreased the O-GlcNAcylation of AMPK extensively in HeLa cells, compared to HaCaT cells (Fig. 2C). Immunoprecipitation analysis also showed that O-GlcNAcylated AMPK was considerably higher in HeLa cells than in HaCaT cells, and that quercetin treatment decreased the O-GlcNAcylation of AMPK (Fig. 2D). Taken together, our results suggest that quercetin decreases O-GlcNAcylation, which then decreases O-GlcNAcylation of AMPK.
To elucidate the effect of O-GlcNAcylation on the activation of AMPK, we examined the expression levels of phosphorylated AMPK in the presence or absence of DON (6-diazo-5-oxo-norleucineis), which is a glutamine fructose-6-phosphate aminotransferase inhibitor, and Thiamet G, which is an O-GlcNAcase inhibitor. The results showed that DON decreased O-GlcNAcylation and increased the expression levels of phospho-AMPK, whereas Thiamet G increased O-GlcNAcylation and decreased the expression levels of phospho-AMPK (Fig. 3A). We also checked the effect of DON and Thiamet G on O-GlcNAcylation of AMPK using the sWGA assay. We found that the reduction of O-GlcNAcylation by DON resulted in decreased O-GlcNAcylation of AMPK, and the hyper-O-GlcNAcylation induced by Thiamet G resulted in increased O-GlcNAcylation of AMPK (Fig. 3B). Immunoprecipitation assays showed that the inhibition of O-GlcNAcylation leads to decreased interaction between O-GlcNAc and AMPK, while hyper O-GlcNAcylation increases the interaction between O-GlcNAc and AMPK (Fig. 3C), indicating that inhibition of O-GlcNAcylation decreased O-GlcNAcylated AMPK. These data suggest that alteration of O-GlcNAcylation regulates the activation of AMPK.
We next determined the effect of quercetin on SREBP-1, a downstream target of AMPK and a transcriptional factor involved in lipogenesis. We found that the levels of SREBP-1 were higher in HeLa cells than in HaCaT cells (Fig. 4A), and that quercetin treatment significantly decreased SREBP-1 levels. Next, we evaluated the effects of quercetin on downstream targets of SREBP-1, including FAS and ACC (Fig. 4A). We found that levels of FAS and ACC were significantly decreased in cells treated with quercetin (Fig. 4A). Based on this observed downregulation of SREBP1 and lipogenic enzymes by quercetin, we extrapolated that this effect could be mediated by activation of AMPK. Indeed, we found that treatment with quercetin activates AMPK by decreasing O-GlcNAcylation of AMPK and increasing AMPK phosphorylation (Figs. 2D, 4A). To confirm whether quercetin regulates SREBP-1 and lipogenic enzymes in cancer cells by activating AMPK via decreasing O-GlcNAcylation, we treated HeLa and HaCaT cells with DON and Thiamet G. The hyper-O-GlcNAcylation induced by Thiamet G resulted in decreased phosphorylated AMPK, as well as increased SREBP-1 and its transcriptional targets; interestingly, reducing O-GlcNAcylation by treatment with DON reversed these changes (Fig. 4B). Taken together, our data indicate that quercetin may decrease the expression of SREBP-1 and its transcriptional targets by activating AMPK via O-GlcNAcylation.
Immunofluorescence staining showed that, compared to HaCaT cells, the majority of HeLa cells were strongly positive for OGT and SREBP-1. Quercetin treatment decreased the immunoreactivities of OGT and SREBP-1. In non-treated HeLa cells, SREBP-1 immunoreactivity was dominant in the nucleus, and these cells were also positive for OGT, whereas quercetin treatment reversed these changes (Fig. 5A). Given that the decrease in O-GlcNAcylation decreases cell proliferation and induces cell death, we checked the effect of quercetin on cell death using the TUNEL assay. In non-treated cells, no TUNEL-positive cells were detected, whereas among quercetin-treated cells, TUNEL-positive cells were detected, as indicated by the arrowhead. Furthermore, cell death was higher in HeLa cells then in HaCaT cells (Fig. 5B). Taken together, our data suggest that the effects of quercetin are more severe on cancer cells than on normal epithelial cells, and quercetin may induce cell death by regulating global O-GlcNAcylation.
Natural products, particularly flavonoids found in the human diet, have been reported to exert anticancer effects on several types of cancer cells [2728]. Among them, quercetin has been reported to show anti-proliferative and apoptosispromoting effects, but the molecular mechanism is still unclear. In the present study, we found that quercetin decreased cell viability and induced cell death in cervical cancer cells, whereas its effect on human normal epithelial cell was limited. Here, we demonstrated that quercetin decreased cell proliferation and induced cell death in cervical cancer cells through regulation of O-GlcNAcylation.
O-GlcNAcylation is increased in various cancers; the elevated O-GlcNAcylation in cancer cells promotes cell growth and cell proliferation [345]. In agreement with previous studies, we found elevated levels of O-GlcNAcylation in cervical cancer cells compared to normal epithelial cells. Interestingly, quercetin significantly decreased global O-GlcNAcylation in cervical cancer cells. On the basis of previous reports, this decrease in O-GlcNAcylation reduces the growth and proliferation of several types of cancer cells [345]. We suggested that the anticancer effect of quercetin might be due to a decrease in O-GlcNAcylation. Recently, amentoflavone, a bioflavonoid derivative, has been identified to inhibit O-GlcNAcylation [29]. However, quercetin is the most commonly dietary flavonoid, and hence it could be efficiently used to target O-GlcNAcylation.
Increase in O-GlcNAcylation plays an important role in regulating cancer metabolism by modifying key transcription factors and metabolic enzymes [12]. AMPK, a cellular energy sensor and major component of nutrient pathway activation has been linked with O-GlcNAcylation, and numerous studies have revealed that inhibition of O-GlcNAcylation in cancer cells induces metabolic stress and thereby activates the AMPK pathway [2021]. Activation of AMPK reduces cell proliferation and induces apoptosis by regulating a number of tumour suppressor and pro-apoptotic proteins [151617]. Here, we found that cervical cancer cells have higher levels of O-GlcNAcylated AMPK and lower levels of phospho-AMPK than normal epithelial cells. We assume that the increased O-GlcNAcylation of AMPK in cervical cancer cells could cause decreased AMPK activation due to O-GlcNAcylation competing with phosphorylation. As previously reported, O-GlcNAc and phosphorylation in some cases have a reciprocal relationship where they compete for the same residue or adjacent sites [30]. However, quercetin decreases the O-GlcNAcylation of AMPK and increases the level of phosphorylated AMPK. Our results are supported by a recent study, which found that induction of O-GlcNAcylation reduces AMPK activation and promotes the proliferation of colon cancer cells. We further validated our results by inhibiting O-GlcNAcylation with DON or increasing O-GlcNAcylation with Thiamet G, resulting in the activation and suppression of AMPK, respectively. Our data suggest that O-GlcNAcylation-dependent reduction of AMPK signalling could be one of the major effectors of cancer progression.
We further examine the effect of quercetin on SREBP-1, a downstream protein target of AMPK that is involved in lipogenesis and is known to play a crucial role in cell proliferation. As speculated, SREBP-1 expression was significantly reduced in quercetin-treated cells, suggesting that the decreased expression of SREBP-1 might be due to AMPK activation. Likewise, our findings are supported by results from previous studies that have shown that AMPK activation regulates SREBP-1 and reduces cancer progression [22].
In conclusion, our findings demonstrate that quercetin exhibits its anticancer effect by decreasing O-GlcNAcylation, an important regulator of cancer progression. In addition, quercetin restores APMK activation by decreasing the O-GlcNAcylation of AMPK. Further studies are needed to explore the mechanism by which quercetin regulates O-GlcNAcylation levels, and to address its utility as a potential therapeutic agent in cancer.
Figures and Tables
Fig. 1
Quercetin decreases cell viability and induces cell death in HaCaT and HeLa cells. (A–C) MTT assay of HaCaT and HeLa cells after treatment with quercetin (0–200 µM) for 24 hours. Bar graph representing the viability of HaCaT (A) and HeLa (B) cells. (C) Line graph representing the comparison of cell viability between HaCaT and HeLa cells. (D) Representative western blot analysis and relative bar graph quantification of PARP, cleaved PARP, caspase 3, and cleaved caspase 3 in HeLa and HaCaT cells after treatment with 50 µM quercetin for 24 hours. Band intensity was normalised to β-actin. Each experiment was performed three times. PARP, poly (ADP ribose) polymerase; SEM, standard error of mean. Data represent the mean±SEM of three independent experiments. **P<0.005, ***P<0.001.
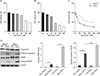
Fig. 2
Quercetin decreases the expression of OGT, O-GlcNAc, and O-GlcNAcylated AMPK. Representative western blot analysis and relative bar graph quantification of OGT (A) and O-GlcNAc (B) in HeLa and HaCaT cells after treatment with 50 µM quercetin for 24 hours. (C) Upper panel: western blots using anti-O-GlcNAc and AMPK antibodies, of O-GlcNAcylated proteins precipitated by agarose-bound succinylated WGA beads, from HaCaT and HeLa cells treated with or without 50 µM quercetin for 24 hours. The specificity of WGA binding was confirmed by adding GlcNAc. Lower panel: relative bar graph quantification of relative O-GlcNAc levels. (D) Upper panel: western blots, using anti-O-GlcNAc and AMPK antibodies, of AMPK immunoprecipitated from cell lysates of HaCaT and HeLa cells treated with or without 50 µM quercetin for 24 hours. Lower panel: relative bar graph quantification of relative O-GlcNAc levels. Densitometry of immunoprecipitated AMPK to O-GlcNAc was normalised to IgG. OGT, O-linked N-acetylglucosamine transferase; O-GlcNAc, O-linked N-acetylglucosamine; AMPK, AMP-activated protein kinase; WGA, wheat germ agglutinin; SEM, standard error of mean; CTL, control; Quer, quercetin; IB, immunoblot. Each experiment was performed three times. Data represent the mean±SEM of three independent experiments. *P<0.05, **P<0.005, ***P<0.001.
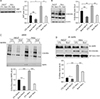
Fig. 3
O-GlcNAcylation regulates activation of AMPK. (A) Representative western blot analysis and relative bar graph quantification of O-GlcNAc, AMPK, and p-AMPK in HeLa and HaCaT cells after treatment with 100 µM DON or 10 µM TMG for 24 hours. β-actin was used as a loading control. (B) Upper panel: western blots, using anti-O-GlcNAc and AMPK antibodies, of O-GlcNAcylated proteins precipitated by agarose-bound succinylated WGA beads, from cell lysates of HaCaT and HeLa cells after treatment with 100 µM DON or 10 µM TMG for 24 hours. Specificity of WGA binding was confirmed by adding GlcNAc. Lower panel: quantitative analysis of relative O-GlcNAc level. (C) Upper panel: western blots, using anti-O-GlcNAc and AMPK antibodies, of AMPK immunoprecipitated from the cell lysates of HaCaT and HeLa cells treated with 100 µM DON or 10 µM TMG for 24 hours. Lower panel: quantitative analysis of relative O-GlcNAc levels. Densitometry of immunoprecipitated AMPK to O-GlcNAc was normalised to IgG. AMPK, AMP-activated protein kinase; O-GlcNAc, O-linked N-acetylglucosamine; DON, 6-diazo-5-oxo-norleucineis; TMG, Thiamet G; WGA, wheat germ agglutinin; CTL, control. Each experiment was performed three times. Data represent the mean±SEM of three independent experiments. *P<0.05, **P<0.005, ***P<0.001.
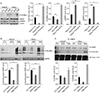
Fig. 4
Quercetin regulates SREBP-1 and its target proteins by activating AMPK via regulating O-GlcNAcylation. (A) Quercetin treatment causes decreased O-GlcNAcylation, increased p-AMPK levels, and decreased levels of SREBP-1 and its target proteins. Representative western blot analysis and relative bar graph quantification of O-GlcNAc, AMPK, pAMPk, SREBP-1, ACC, and FAS in the cell lysates of HeLa and HaCaT cells treated with or without 50 µM quercetin. (B) O-GlcNAcylation regulates expression of p-AMPK, SREBP-1, and SREBP-1 target proteins. Representative western blot analysis and relative bar graph quantification of O-GlcNAc, AMPK, pAMPk, SREBP-1, ACC, and FAS in the cell lysates of HeLa and HaCaT cells treated with 100 µM DON or 10 µM TMG. Band intensity was normalised to β-actin. SREBP-1, sterol regulatory element binding protein 1; AMPK, AMP-activated protein kinase; O-GlcNAc, O-linked N-acetylglucosamine; ACC, acetyl-CoA carboxylase; FAS, fatty acid synthase; DON, 6-diazo-5-oxo-norleucineis; SEM, standard error of mean; TMG, Thiamet G; CTL, control; Quer, quercetin. Each experiment was performed three times. Data represent the mean±SEM of three independent experiments. *P<0.05, **P<0.005, ***P<0.001.
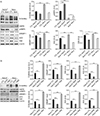
Fig. 5
Quercetin decreases the immunoreactivity of OGT and SREBP-1, and increases the number of TUNEL-positive cells. Representative images of double immunofluorescence staining for OGT (green) and SREBP-1 (red) in HaCaT and HeLa cells after treatment with or without 50 µM quercetin for 24 hours. Merged images were generated by overlaying the images of OGT and SREBP-1 staining. The enlarged portion is indicated by the box. (B) Representative image of TUNEL (red) along with DAPI (blue) staining for nuclear localisation in HaCaT and HeLa cells after treatment with or without 50 µM quercetin for 24 hours. Merge images were generated by overlaying the images of TUNEL and DAPI staining. Arrows indicate TUNEL-positive cells. OGT, O-linked N-acetylglucosamine transferase; SREBP-1, sterol regulatory element binding protein 1; TUNEL, terminal deoxynucleotidyl transferase-mediated deoxyuridine triphosphate nick-end labelling; CTL, control; Quer, quercetin. Scale bars=100 µm.
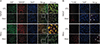
Acknowledgements
This work was supported by MAFRA(117082-03) and the Basic Science Research Program of the National Research Foundation (NRF) of Korea funded by the Ministry of Science, ICT, and Future Planning (NRF-2015R1A5A2008833).
References
1. Ma Z, Vosseller K. Cancer metabolism and elevated O-GlcNAc in oncogenic signaling. J Biol Chem. 2014; 289:34457–34465.


2. Ferrer CM, Sodi VL, Reginato MJ. O-GlcNAcylation in cancer biology: linking metabolism and signaling. J Mol Biol. 2016; 428:3282–3294.


3. Jiang M, Qiu Z, Zhang S, Fan X, Cai X, Xu B, Li X, Zhou J, Zhang X, Chu Y, Wang W, Liang J, Horvath T, Yang X, Wu K, Nie Y, Fan D. Elevated O-GlcNAcylation promotes gastric cancer cells proliferation by modulating cell cycle related proteins and ERK 1/2 signaling. Oncotarget. 2016; 7:61390–61402.


4. Moriwaki K, Asahi M. Augmented TME O-GlcNAcylation promotes tumor proliferation through the inhibition of p38 MAPK. Mol Cancer Res. 2017; 15:1287–1298.


5. Ma Z, Vocadlo DJ, Vosseller K. Hyper-O-GlcNAcylation is antiapoptotic and maintains constitutive NF-kappaB activity in pancreatic cancer cells. J Biol Chem. 2013; 288:15121–15130.
6. Ferrer CM, Lu TY, Bacigalupa ZA, Katsetos CD, Sinclair DA, Reginato MJ. O-GlcNAcylation regulates breast cancer metastasis via SIRT1 modulation of FOXM1 pathway. Oncogene. 2017; 36:559–569.


7. Niu Y, Xia Y, Wang J, Shi X. O-GlcNAcylation promotes migration and invasion in human ovarian cancer cells via the RhoA/ ROCK/MLC pathway. Mol Med Rep. 2017; 15:2083–2089.
8. Miles SL, McFarland M, Niles RM. Molecular and physiological actions of quercetin: need for clinical trials to assess its benefits in human disease. Nutr Rev. 2014; 72:720–734.


9. Russo M, Spagnuolo C, Tedesco I, Bilotto S, Russo GL. The flavonoid quercetin in disease prevention and therapy: facts and fancies. Biochem Pharmacol. 2012; 83:6–15.


10. Li XM, Liu J, Pan FF, Shi DD, Wen ZG, Yang PL. Quercetin and aconitine synergistically induces the human cervical carcinoma HeLa cell apoptosis via endoplasmic reticulum (ER) stress pathway. PLoS One. 2018; 13:e0191062.


11. Kashyap D, Mittal S, Sak K, Singhal P, Tuli HS. Molecular mechanisms of action of quercetin in cancer: recent advances. Tumour Biol. 2016; 37:12927–12939.


12. Li X, Zhou N, Wang J, Liu Z, Wang X, Zhang Q, Liu Q, Gao L, Wang R. Quercetin suppresses breast cancer stem cells (CD44(+)/CD24(−)) by inhibiting the PI3K/Akt/mTOR-signaling pathway. Life Sci. 2018; 196:56–62.
13. Hamilton KE, Rekman JF, Gunnink LK, Busscher BM, Scott JL, Tidball AM, Stehouwer NR, Johnecheck GN, Looyenga BD, Louters LL. Quercetin inhibits glucose transport by binding to an exofacial site on GLUT1. Biochimie. 2018; 151:107–114.


14. Moreira L, Araújo I, Costa T, Correia-Branco A, Faria A, Martel F, Keating E. Quercetin and epigallocatechin gallate inhibit glucose uptake and metabolism by breast cancer cells by an estrogen receptor-independent mechanism. Exp Cell Res. 2013; 319:1784–1795.


15. Kuhajda FP. AMP-activated protein kinase and human cancer: cancer metabolism revisited. Int J Obes (Lond). 2008; 32:Suppl 4. S36–S41.


16. Luo Z, Zang M, Guo W. AMPK as a metabolic tumor suppressor: control of metabolism and cell growth. Future Oncol. 2010; 6:457–470.


17. Kim I, He YY. Targeting the AMP-activated protein kinase for cancer prevention and therapy. Front Oncol. 2013; 3:175.


18. Luo Z, Saha AK, Xiang X, Ruderman NB. AMPK, the metabolic syndrome and cancer. Trends Pharmacol Sci. 2005; 26:69–76.


19. Li W, Saud SM, Young MR, Chen G, Hua B. Targeting AMPK for cancer prevention and treatment. Oncotarget. 2015; 6:7365–7378.


20. Ishimura E, Nakagawa T, Moriwaki K, Hirano S, Matsumori Y, Asahi M. Augmented O-GlcNAcylation of AMP-activated kinase promotes the proliferation of LoVo cells, a colon cancer cell line. Cancer Sci. 2017; 108:2373–2382.
21. Ferrer CM, Lynch TP, Sodi VL, Falcone JN, Schwab LP, Peacock DL, Vocadlo DJ, Seagroves TN, Reginato MJ. O-GlcNAcylation regulates cancer metabolism and survival stress signaling via regulation of the HIF-1 pathway. Mol Cell. 2014; 54:820–831.


22. Li Y, Xu S, Mihaylova MM, Zheng B, Hou X, Jiang B, Park O, Luo Z, Lefai E, Shyy JY, Gao B, Wierzbicki M, Verbeuren TJ, Shaw RJ, Cohen RA, Zang M. AMPK phosphorylates and inhibits SREBP activity to attenuate hepatic steatosis and atherosclerosis in dietinduced insulin-resistant mice. Cell Metab. 2011; 13:376–388.


23. Wen YA, Xiong X, Zaytseva YY, Napier DL, Vallee E, Li AT, Wang C, Weiss HL, Evers BM, Gao T. Downregulation of SREBP inhibits tumor growth and initiation by altering cellular metabolism in colon cancer. Cell Death Dis. 2018; 9:265.


24. Li X, Chen YT, Hu P, Huang WC. Fatostatin displays high antitumor activity in prostate cancer by blocking SREBP-regulated metabolic pathways and androgen receptor signaling. Mol Cancer Ther. 2014; 13:855–866.


25. Kwan HT, Chan DW, Cai PC, Mak CS, Yung MM, Leung TH, Wong OG, Cheung AN, Ngan HY. AMPK activators suppress cervical cancer cell growth through inhibition of DVL3 mediated Wnt/beta-catenin signaling activity. PLoS One. 2013; 8:e53597.
26. Yung MM, Chan DW, Liu VW, Yao KM, Ngan HY. Activation of AMPK inhibits cervical cancer cell growth through AKT/ FOXO3a/FOXM1 signaling cascade. BMC Cancer. 2013; 13:327.


27. Chahar MK, Sharma N, Dobhal MP, Joshi YC. Flavonoids: a versatile source of anticancer drugs. Pharmacogn Rev. 2011; 5:1–12.
28. Batra P, Sharma AK. Anti-cancer potential of flavonoids: recent trends and future perspectives. 3 Biotech. 2013; 3:439–459.

