Abstract
The ganglion cardiacum or juxtaductal body is situated along the left recurrent laryngeal nerve in the aortic window and is an extremely large component of the cardiac nerve plexus. This study was performed to describe the morphologies of the ganglion cardiacum or juxtaductal body in human fetuses and to compare characteristics with intracardiac ganglion. Ganglia were immunostained in specimens from five fetuses of gestational age 12–16 weeks and seven fetuses of gestational age 28–34 weeks. Many ganglion cells in the ganglia were positive for tyrosine hydroxylase (TH; sympathetic nerve marker) and chromogranin A, while a few neurons were positive for neuronal nitric oxide synthase (NOS; parasympathetic nerve marker) or calretinin. Another ganglion at the base of the ascending aorta carried almost the same neuronal populations, whereas a ganglion along the left common cardinal vein contained neurons positive for chromogranin A and NOS but no or few TH-positive neurons, suggesting a site-dependent difference in composite neurons. Mixtures of sympathetic and parasympathetic neurons within a single ganglion are consistent with the morphology of the cranial base and pelvic ganglia. Most of the intracardiac neurons are likely to have a non-adrenergic non-cholinergic phenotype, whereas fewer neurons have a dual cholinergic/noradrenergic phenotype. However, there was no evidence showing that chromogranin A- and/or calretinin-positive cardiac neurons corresponded to these specific phenotypes. The present study suggested that the ganglion cardiacum was composed of a mixture of sympathetic and parasympathetic neurons, which were characterized the site-dependent differences in and near the heart.
The ganglion cardiacum of Wrisberg [1] attaches to the left recurrent laryngeal nerve in the aortic window (Fig. 1). It is solitary and independent and is the largest of several ganglia scattered throughout the cardiac nerve plexus along the thoracic great arteries. To our knowledge, however, this ganglion has not been assessed immunohistochemically. Recent anatomical studies of human cardiac nerves (e.g., Pauza et al. [2], Kawashima [3]) did not analyze this ganglion, possibly because these studies focused on intracardiac neurons, especially epicardiac ganglia, not on extracardiac ganglia. In previous studies using whole-mount specimens, choline acetyltransferase was not regarded as a specific cholinergic nerve marker but as a pan-neuromarker [24].
Classically, autonomic nerves have been classified as (1) sympathetic or adrenergic nerves expressing tyrosine hydroxylase (TH) and/or dopamine β-hydroxylase and (2) parasympathetic or cholinergic nerves positive for vesicular acetylcholine transporter. The latter nerves are often positive for neuronal nitric oxide synthase (NOS). Immunohistochemical assays have shown the presence of these enzymes and markers in the intracardiac nerves and ganglion cells of rabbits [56], mice [78], rats [9], guinea pigs [101112], and humans [13141516]. Most of these studies, however, did not determine the topographical anatomy of the neurons examined. In addition, chromogranin A and calretinin were found to be useful neuromarkers in human fetuses because of their strong resistance to degradation in formalin solution [1718].
In human fetuses, the ganglion cardiacum has also been called the juxtaductal body [19] and the aortico-pulmonary ganglionic mass [20]. The terms “body” and “mass,” being similar to the coccygeal body, suggest that these ganglia are larger in size than most ganglia and are histologically unique, as they contain many non-ganglionic cells. Non-ganglionic cells are major constituents of the coccygeal body and, because of their vascular origin, are strongly positive for expression of smooth muscle actin (SMA) [21]. This study was designed to describe the morphologies of the ganglion cardiacum or juxtaductal body in human fetuses and to compare these characteristics with those of intracardiac ganglia.
This study was performed in accordance with the principles of the Declaration of Helsinki 1995 (as revised in 2013). Semiserial sagittal sections were obtained from five Chinese fetuses of gestational age 12–16 weeks and crown-rump length (CRL) 70–125 mm and from seven Japanese fetuses of gestational age 28–34 weeks and CRL, 235–290 mm.
The five Chinese fetuses were obtained by induced abortion and donated by their families to the Department of Anatomy, Yanbian University Medical College, Yanji, China, until 2016; their use for research was approved by the university ethics committee (No. BS-13-35). Following induced abortion, the mother was orally informed by an obstetrician at the college teaching hospital of the possibility of donating the fetus for research; no attempt was made to encourage the donation actively. After the mother agreed, the fetus was assigned a specimen number and stored in 10% w/w neutral formalin solution for more than 1 month. Because of specimen number randomization, there was no possibility of contacting the family at a later date. The trunk samples were decalcified by incubation at 4℃ in 0.5-mol/l EDTA (pH 7.5) (Decalcifying Solution B, Wako, Tokyo, Japan) for 3–5 days, depending on the size of the sample. The specimens were embedded in paraffin, sectioned sagittally at 20–50 µm intervals or serially, depending on specimen size, and cut into 5-µm thick sections.
The seven Japanese fetal specimens were parts of a collection maintained by the Department of Anatomy, Akita University, Akita, Japan. The fetuses had been donated by their families to the Department from 1975–1985 and preserved in 10% w/w neutral formalin solution for more than 30 years. The available data were limited to the date of donation and gestational age, but there was no information on family name, the names of the obstetrician and/or hospital and the reason for abortion. Use of these specimens for research was approved by the university ethics committee (No. 1428). After removal of the thoracic viscera, large paraffin blocks were prepared, containing the lungs, heart, thymus, esophagus and their associated arteries and veins. Semiserial sagittal sections at 50–200 µm intervals were prepared and cut into 5-µm thick sections.
The primary antibodies used for immunohistochemistry were (1) mouse monoclonal anti-human S100 protein (1:100, Z0311, Dako, Glostrup, Denmark); (2) mouse monoclonal anti-human chromogranin A (1:200, M0869, Dako); (3) rabbit polyclonal anti-human neuronal NOS (1:200, Cell Signaling Technology, Beverly, MA, USA); (4) rabbit polyclonal anti-human TH (1:500, ab152, Millipore-Chemicon, Temecula, CA, USA); (5) mouse monoclonal anti-human calretinin (1:2, MA1-39562, Invitrogen, Thermo Fisher Scientific, Yokohama, Japan); and (6) mouse monoclonal anti-human α-SMA (1:100, M0851, Dako). S100 protein was regarded as a pan-neuronal marker, neuronal NOS as a parasympathetic nerve marker and chromogranin A and TH as sympathetic nerve markers. Antigen retrieval for antibodies 2–5 was performed by heating in a microwave oven at 500 W for 15 minutes at pH 6. Following incubation with primary antibody, sections were incubated for 30 minutes with secondary antibody, consisting of a 1:1,000 dilution of Histofine Simple Stain Max-PO (Nichirei, Tokyo, Japan) labeled with horseradish peroxidase. Antigen-antibody reactions were detected by incubation for 3–5 min with diaminobenzidine (Histofine Simple Stain DAB, Nichirei). All samples were counterstained with hematoxylin. Negative controls consisted of samples without the primary antibody. All sections were observed and photographed with a Nikon Eclipse 80 (Tokyo, Japan).
All twelve specimens were positive for S100 and SMA, and some ganglion cells and nerves in four of the Chinese fetuses and six of the Japanese fetuses were positive for chromogranin A. Although nerve elements of 11 of the 12 fetuses were positive for calretinin, the finding that all peripheral nerves were positive for calretinin suggested that the positivity was non-specific. In contrast, nerve elements in two of the Chinese and five of the Japanese fetuses were negative for TH and NOS possibly due to long perservation.
The ganglion cardiacum was attached to the left recurrent laryngeal nerve behind the ductus arteriosus and was located in the aortic window (Figs. 2, 3, 4). This ganglion was oval and large in size, being 0.1–0.5 mm in maximum diameter at gestational age 12–16 weeks and 0.3–1.0 mm in maximum diameter at gestational age at 28–34 weeks. The ganglion cardiacum contained 20–200 neurons per section, and was accompanied by or contained a mass of undifferentiated cells that were negative for both S100 and SMA (Figs. 3A–C, 4B, C). When ganglion cells and undifferentiated cells were mixed, the latter cells were embedded in a S100-positive meshwork structure (Fig. 4B). Many ganglion cells in the ganglion cardiacum were positive for TH and chromogranin A (Figs. 2D, 3D, F), with few being positive for neuronal NOS or calretinin (Figs. 3E, 4D, E): Per section, there were 2–5 NOS-positive neurons, or fewer than 5% of cell bodies; 5–10 TH-positive neurons, or fewer than 10% of cell bodies; and 0–3 calretinin-positive neurons, or fewer than 1% of cell bodies.
Most other ganglia on the cardiac side of the ganglion cardiacum were attached to the pulmonary arterial trunk, ascending aorta and/or left common cardinal vein in the pericardiac sac (Figs. 3A, 5). The left common cardinal vein ran near the ganglion cardiacum and passed between the left atrium and left main bronchus (Fig. 2A). These ganglia were consistently smaller than the ganglion cardiacum, being <0.2 mm in maximum diameter at gestational age 12–16 weeks and <0.3 mm in maximum diameter at gestational age 28–34 weeks. All of these ganglia contained neurons positive for chromogranin A and neuronal NOS (Fig. 5D, E), but only some contained TH-positive neurons (Fig. 5C). Similarly, all intramural neurons of the esophagus were positive for both neuronal NOS and chromogranin A (Fig. 5G, H), but often negative for TH (Fig. 5F). In addition, small epicardiac ganglia were seen along the coronary arterial branches, as well as on the atrium; their composite ganglion cells were frequently positive for chromogranin A, but rarely positive for TH or NOS.
The present study demonstrated that the ganglion cardiacum in fetuses contained abundant TH-positive neurons and a few NOS-positive neurons. These findings suggested that the ganglion cardiacum was composed of a mixture of sympathetic and parasympathetic neurons. This type of mixture is commonly seen in the human body, including in the pelvic [22] and cranial base [23] ganglia. Although the stellate ganglion is widely regarded as a typical sympathetic ganglion, it contains ganglion cells expressing parasympathetic nerve markers [2425]. The mixture has also been observed in other epicardiac ganglia along the thoracic great arteries. Ganglia along the great arteries, including the ganglion cardiacum, showed similar patterns of chromogranin A and TH expression. However, a ganglion along the left common cardinal vein contained no or few TH-positive neurons. In the latter, immunoreactivity for chromogranin A was likely to overlap with that of neuronal NOS. Such site-dependent differences have not been reported for ganglia in and near the heart. The observed heterogeneity of these ganglia may reflect a difference in fetal stages of neuronal development.
Although a previous study showed the presence of calbindin-positive neurons in intracardiac ganglia [9], our study was the first to show that ganglion cells and nerves in and near the heart were immunohistochemically positive for chromogranin A and calretinin. Chromogranin A has been used to identify the adrenal medulla [2627], whereas calretinin expression has been reported in ganglion cells of the pelvic plexus [28] and enteric ganglia [29]. Intracardiac ganglion cells may have specific immunohistochemical characteristics, with most or some of these neurons likely to be non-adrenergic and non-cholinergic (NANC) [30]; and some carrying a dual cholinergic/noradrenergic phenotype [81325]. Results of the present study indicate that both chromogranin A- and calretinin-positive neurons should not be considered simply sympathetic or parasympathetic. However, there is no evidence showing that chromogranin A- and/or calretinin-positive cardiac neurons correspond to the NANC or dual phenotypic.
The ganglion cardiacum or juxtaductal body usually contains or is accompanied by a cluster of undifferentiated cells negative for both S100 and SMA. This non-neuronal cluster was similar to the coccygeal body, but the absence of SMA expression differentiated this cluster from the vessel-poor type of the coccygeal body [21]. Thus, these undifferentiated cells were unlikely to be of vascular origin. Embryonic stem cells (NCAM+, synaptophysin+, neuron specific enolase+, chromogranin+, bcl-2+, cytokeratins−, CD45−, CD3−, carcinoembryonic antigen−) from human fetuses of gestational age 7–25 weeks were shown to cluster along the viscera, brain, spinal cord and bone [31]. Although we did not perform those detailed immunohistochemical identifications, undifferentiated cells in and near the ganglion cardiacum may correspond to this stem cell mass. Moreover, some chromogranin A-positive neurons in the cardiac ganglia may correspond to embryonic stem cells.
This study was performed to determine the topographical anatomy of the ganglion cardiacum in human fetuses. Many ganglion cells in the ganglia were positive for TH and chromogranin A, while a few neurons were positive for NOS or calretinin. Another ganglion at the ascending aorta carried almost the same neuronal populations, whereas a ganglion along the left common cardinal vein contained neurons positive for chromogranin A and NOS but no or few TH-positive neurons, suggesting a site-dependent difference in composite neurons. Consequentially, the present study suggested that the ganglion cardiacum or juxtaductal body was composed of a mixture of sympathetic and parasympathetic neurons, which were characterized the site-dependent differences in and near the heart.
Acknowledgements
This study was supported in part by a Grant-in-Aid for Scientific Research [JSPS KAKENHI No. 16K08435] from the Ministry of Education, Culture, Sports, Science and Technology in Japan.
References
1. Toldt C. Anatomischer Atlas fűr Studierende und Arzte. Vol. 6. Berlin: Urban & Schwarzenberg;1903.
2. Pauza DH, Skripka V, Pauziene N, Stropus R. Morphology, distribution, and variability of the epicardiac neural ganglionated subplexuses in the human heart. Anat Rec. 2000; 259:353–382. PMID: 10903529.
3. Kawashima T. The autonomic nervous system of the human heart with special reference to its origin, course, and peripheral distribution. Anat Embryol (Berl). 2005; 209:425–438. PMID: 15887046.
4. Taguchi K, Tsukamoto T, Murakami G. Anatomical studies of the autonomic nervous system in the human pelvis by the whole-mount staining method: left-right communicating nerves between bilateral pelvic plexuses. J Urol. 1999; 161:320–325. PMID: 10037431.
5. Brack KE. The heart's ‘little brain’ controlling cardiac function in the rabbit. Exp Physiol. 2015; 100:348–353. PMID: 25833107.
6. Pauziene N, Alaburda P, Rysevaite-Kyguoliene K, Pauza AG, Inokaitis H, Masaityte A, Rudokaite G, Saburkina I, Plisiene J, Pauza DH. Innervation of the rabbit cardiac ventricles. J Anat. 2016; 228:26–46. PMID: 26510903.
7. Maifrino LB, Liberti EA, Castelucci P, De Souza RR. NADPH-diaphorase positive cardiac neurons in the atria of mice: a morphoquantitative study. BMC Neurosci. 2006; 7:10. PMID: 16451738.
8. Rysevaite K, Saburkina I, Pauziene N, Vaitkevicius R, Noujaim SF, Jalife J, Pauza DH. Immunohistochemical characterization of the intrinsic cardiac neural plexus in whole-mount mouse heart preparations. Heart Rhythm. 2011; 8:731–738. PMID: 21232628.
9. Richardson RJ, Grkovic I, Anderson CR. Immunohistochemical analysis of intracardiac ganglia of the rat heart. Cell Tissue Res. 2003; 314:337–350. PMID: 14523644.
10. Steele PA, Gibbins IL, Morris JL, Mayer B. Multiple populations of neuropeptide-containing intrinsic neurons in the guinea-pig heart. Neuroscience. 1994; 62:241–250. PMID: 7816202.
11. Hoover DB, Ganote CE, Ferguson SM, Blakely RD, Parsons RL. Localization of cholinergic innervation in guinea pig heart by immunohistochemistry for high-affinity choline transporters. Cardiovasc Res. 2004; 62:112–121. PMID: 15023558.
12. Parsons RL, Locknar SA, Young BA, Hoard JL, Hoover DB. Presence and co-localization of vasoactive intestinal polypeptide with neuronal nitric oxide synthase in cells and nerve fibers within guinea pig intrinsic cardiac ganglia and cardiac tissue. Cell Tissue Res. 2006; 323:197–209. PMID: 16220273.
13. Tan AY, Li H, Wachsmann-Hogiu S, Chen LS, Chen PS, Fishbein MC. Autonomic innervation and segmental muscular disconnections at the human pulmonary vein-atrial junction: implications for catheter ablation of atrial-pulmonary vein junction. J Am Coll Cardiol. 2006; 48:132–143. PMID: 16814659.
14. Hoover DB, Isaacs ER, Jacques F, Hoard JL, Pagé P, Armour JA. Localization of multiple neurotransmitters in surgically derived specimens of human atrial ganglia. Neuroscience. 2009; 164:1170–1179. PMID: 19747529.
15. Singh S, Johnson PI, Javed A, Gray TS, Lonchyna VA, Wurster RD. Monoamine- and histamine-synthesizing enzymes and neurotransmitters within neurons of adult human cardiac ganglia. Circulation. 1999; 99:411–419. PMID: 9918529.
16. Singh S, Gray T, Wurster RD. Nitric oxide and carbon monoxide synthesizing enzymes and soluble guanylyl cyclase within neurons of adult human cardiac ganglia. Auton Neurosci. 2009; 145:93–98. PMID: 19106038.
17. Katori Y, Jin ZW, Kawase T, Hong KH, Murakami G, Cho BH. Developmental changes in the distribution of calretinin-immunoreactive cells in human fetal nasal epithelium. Okajimas Folia Anat Jpn. 2010; 87:5–10. PMID: 20715566.
18. Jin ZW, Cho KH, Jang HS, Murakami G, Rodriguez-Vazquez JF. Median sacral artery, sympathetic nerves, and the coccygeal body: a study using serial sections of human embryos and fetuses. Anat Rec (Hoboken). 2016; 299:819–827. PMID: 27149546.
19. Licata RH. The human embryonic heart in the ninth week. Am J Anat. 1954; 94:73–125. PMID: 13124266.
20. Orts Llorca F, Domenech Mateu JM, Puerta Fonolla J. Innervation of the sinu-atrial node and neighbouring regions in two human embryos. J Anat. 1979; 128(Pt 2):365–375. PMID: 438095.
21. Jin ZW, Cho KH, Jang HS, Murakami G, Rodríguez-Vázquez JF, Yamamoto M, Abe SI. Coccygeal body revisited: an immunohistochemical study using donated elderly cadavers. Anat Rec (Hoboken). 2017; 300:1826–1837. PMID: 28545163.
22. Hinata N, Hieda K, Sasaki H, Murakami G, Abe S, Matsubara A, Miyake H, Fujisawa M. Topohistology of sympathetic and parasympathetic nerve fibers in branches of the pelvic plexus: an immunohistochemical study using donated elderly cadavers. Anat Cell Biol. 2014; 47:55–65. PMID: 24693483.
23. Kiyokawa H, Katori Y, Cho KH, Murakami G, Kawase T, Cho BH. Reconsideration of the autonomic cranial ganglia: an immunohistochemical study of mid-term human fetuses. Anat Rec (Hoboken). 2012; 295:141–149. PMID: 22095632.
24. Roudenok V, Kühnel W. Distribution of vasoactive intestinal polypeptide-, calcitonin gene-related peptide-, somatostatin- and neurofilament-immunoreactivities in sympathetic ganglia of human fetuses and premature neonates. Ann Anat. 2001; 183:213–216. PMID: 11396789.
25. Hoard JL, Hoover DB, Mabe AM, Blakely RD, Feng N, Paolocci N. Cholinergic neurons of mouse intrinsic cardiac ganglia contain noradrenergic enzymes, norepinephrine transporters, and the neurotrophin receptors tropomyosin-related kinase A and p75. Neuroscience. 2008; 156:129–142. PMID: 18674600.
26. Magro G, Grasso S. Immunohisochemical identification and comparison of glial cell lineage in foetal, neonatal, adult and neoplastic human adrenal medulla. Histochem J. 1997; 29:293–299. PMID: 9184844.
27. Inoue S, Cho BH, Song CH, Fujimiya M, Murakami G, Matsubara A. Migration and distribution of neural crest-derived cells in the human adrenal cortex at 9-16 weeks of gestation: an immunohistochemical study. Okajimas Folia Anat Jpn. 2010; 87:11–16. PMID: 20715567.
28. Papka RE, Collins J, Copelin T, Wilson K. Calretinin-immunoreactive nerves in the uterus, pelvic autonomic ganglia, lumbosacral dorsal root ganglia and lumbosacral spinal cord. Cell Tissue Res. 1999; 298:63–74. PMID: 10555540.
29. Liu CY, Zhang H, Christofi FL. Adenylyl cyclase co-distribution with the CaBPs, calbindin-D28 and calretinin, varies with cell type: assessment with the fluorescent dye, BODIPY forskolin, in enteric ganglia. Cell Tissue Res. 1998; 293:57–73. PMID: 9634598.
30. Hasan W. Autonomic cardiac innervation: development and adult plasticity. Organogenesis. 2013; 9:176–193. PMID: 23872607.
31. Terada T. Huge clusters of embryonic stem cells in human embryos: a morphologic study. Microsc Res Tech. 2014; 77:825–831. PMID: 25091607.
Fig. 1
Schematic representation of the cardiac nerve plexus and ganglion cardiacum. Ventral view of the heart in adults. The ganglion cardiacum is located near the ligamentum arteriosum (ligament) and along the left laryngeal recurrent nerve (LRN). AO, ascending aorta; PA, pulmonary arterial trunk; SVC, superior vena cava.
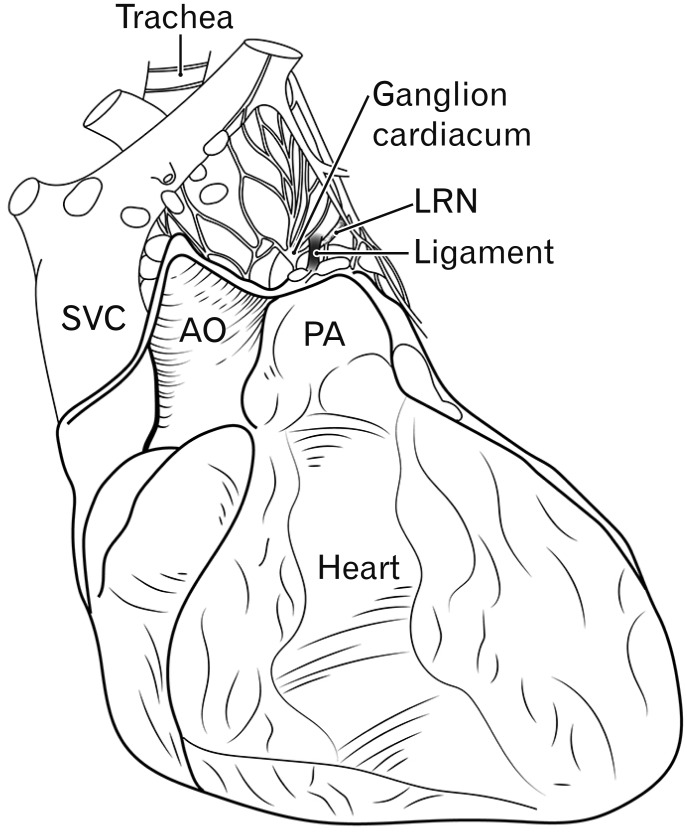
Fig. 2
The ganglion cardiacum at gestational age 10 weeks (crown-rump length 50 mm). Sagittal sections. Panels A and B are stained with hematoxylin and eosin, and panels C, D, and E with antibodies to S100, chromogranin A (chrA), and smooth muscle action (SMA), respectively. Panel A is 50 microns anterior to panel B; panel C corresponds to a square in panel A; and panels D and E correspond to the ganglion cardiacum (ggl) in a square in panel C. The ggl contains a large number of chrA-positive cells, but it does not express SMA. AO, aorta, BO, Botallo's duct (ductus arteriosus); BR, left main bronchus; LA, left atrium; LCCV, left common cardinal vein; PA, pulmonary artery; RAU, right auricle; RV, right ventricle; X, vagus nerve. Scale bars=1 mm (A–C), 0.1 mm (D, E).
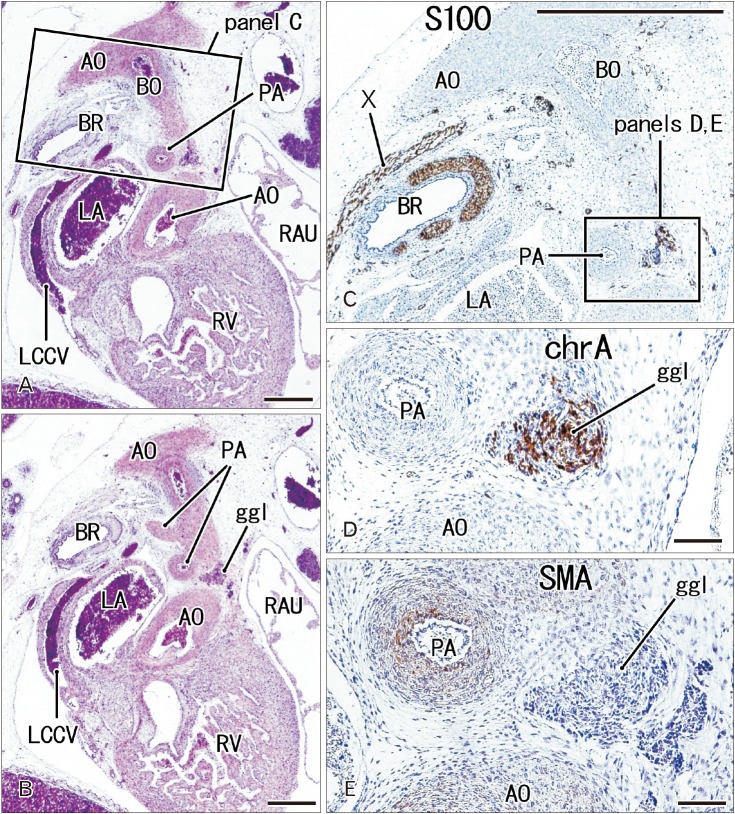
Fig. 3
The ganglion cardiacum (ggl) at gestational age 14 weeks (crown-rump length 106 mm). Sagittal sections stained with antibodies to S100 protein (A), chromogranin A (chrA) (B, F), tyrosine hydroxylase (TH) (C, D), and neuronal nitric oxide synthase (NOS) (E). Panels B and C show sections near panel A. Panel D (or F) is a higher magnification view of a square in panel C (or B). Panels D and E display adjacent sections. The ggl contains abundant neurons expressing chrA (F) or TH (arrows in D), but few NOS-positive cells (arrows in E). A cell mass near the ganglion (star in panels A–C) contains nerves positive for S100 and chrA (A, B). The other ganglion near the aortic valve (AOV) is shown in Fig. 5. AO, aorta, BO, Botallo's duct (ductus arteriosus); BR, left main bronchus; ES, esophagus; LA, left atrium; LCCV, left common cardinal vein; PA, pulmonary artery; THY, thymus. Scale bars=1 mm (A–C), 0.1 mm (D–F).
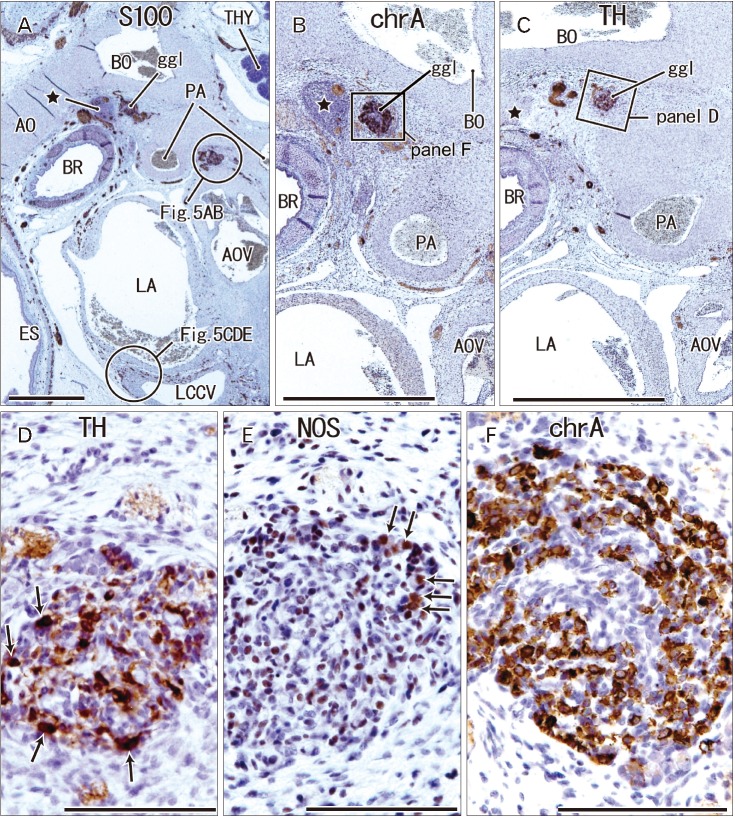
Fig. 4
The ganglion cardiacum (ggl) at gestational age 28 weeks (crown-rump length 235 mm). Sagittal sections stained with antibodies to S100 protein (B), smooth muscle actin (SMA) (C) and calretinin (CAL) (D, E). Panels B–E represent nearly sections, with panel E showing a plane 0.1 mm posterior to panel D. In panel B, the ggl surrounds the left laryngeal recurrent nerve (LRN). The ggl is negative for SMA (C) and contains few neurons positive for calretinin (D, E). AO, aorta, BO, Botallo's duct (ductus arteriosus); BR, left main bronchus; LY, lymph nodes; X, vagus nerve. Scale bars=1 mm (A–C), 0.1 mm (D, E).
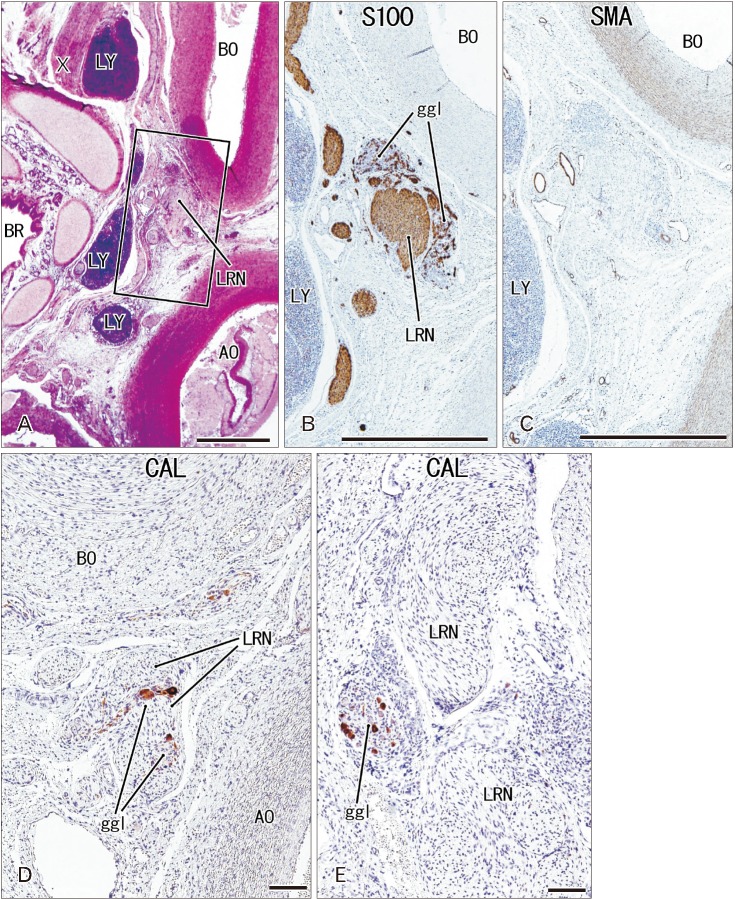
Fig. 5
Ganglion cell clusters other than the ganglion cardiacum. Sagittal sections stained with antibodies to tyrosine hydroxylase (TH) (A, C, F), neuronal nitric oxide synthase (NOS) (B, D, G) and chromogranin A (chrA) (E, H). Panels A and B display a ganglion adjacent to the aortic valve (AOV; topographical anatomy, see the upper circle in Fig. 3A), while panels C–E display a small ganglion (arrow) near the left common cardinal vein (LCCV; topographical anatomy, see the lower circle in Fig. 3A). The former ganglion contains abundant TH-positive neurons (A), whereas the latter ganglion is negative for neurons expressing TH (C). Panels F–H show intramural neurons of the esophagus (ES). TH-positive nerves are present near (arrows in panel F), but not in the ES. All panels were prepared at the same magnification. Scale bar=0.1 mm (A).
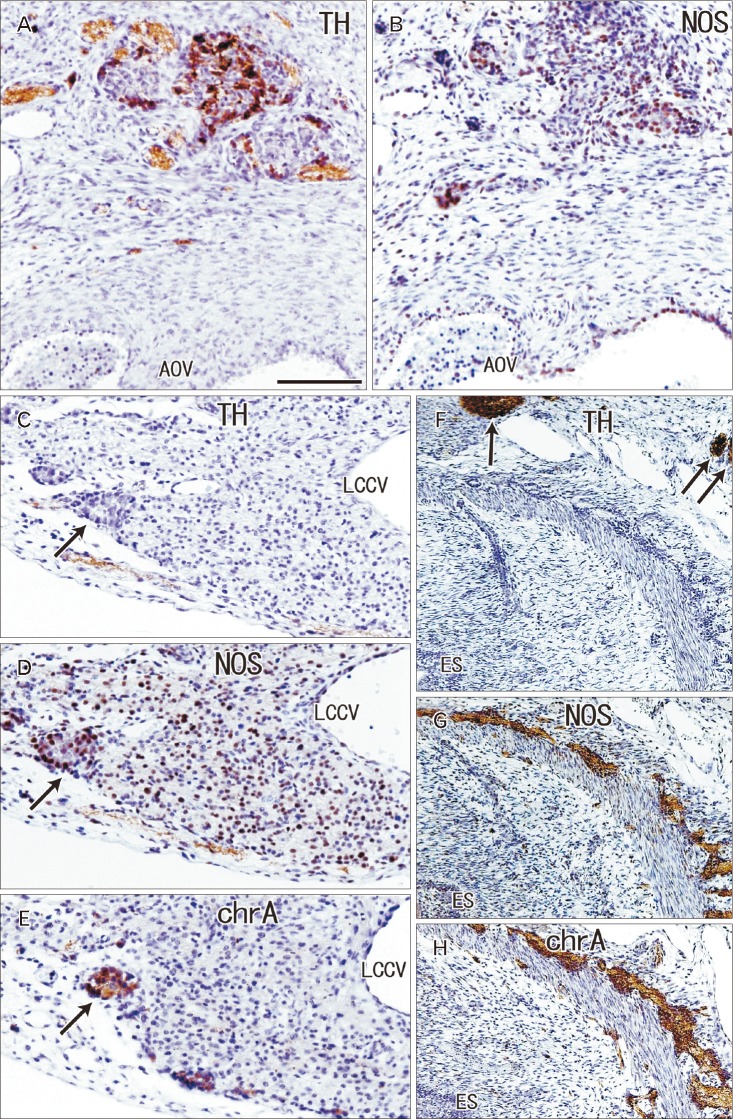