Abstract
Cellular stress severely disrupts endoplasmic reticulum (ER) function, leading to the abnormal accumulation of unfolded or misfolded proteins in the ER and subsequent development of endoplasmic reticulum stress (ERS). To accommodate the occurrence of ERS, cells have evolved a highly conserved, self-protecting signal transduction pathway called the unfolded protein response. Notably, ERS signaling is involved in the development of a variety of diseases and is closely related to tumor development, particularly in breast cancer. This review discusses recent research regarding associations between ERS and tumor metastasis. The information presented here will help researchers elucidate the precise mechanisms underlying ERS-mediated tumor metastasis and provide new directions for tumor therapies.
The endoplasmic reticulum (ER) is an important organelle in eukaryotic cells and serves as a site for protein synthesis, modification, and transport to the Golgi apparatus. Glucose deficiency, hypoxia, calcium imbalance and oxidative stress result in the accumulation of unfolded or misfolded proteins in the ER, which leads to endoplasmic reticulum stress (ERS). ERS is prevalent in tumor tissues and is reported to play a role in tumor development. One of the most important biological characteristics of tumors is the unrestricted proliferation, invasion and migration of tumor cells, which are required for development of distant metastasis and make treating tumors with radical surgery difficult.
Breast cancer is the most common malignant tumor in women, with high morbidity and mortality rates [1]. The global incidence has increased annually and is a serious threat to women's health [2]. Currently, breast cancer treatments generally include surgical resection combined with radiotherapy and chemotherapy. This treatment regimen effectively controls the patient's condition and can prolong survival. However, tumor invasion and migration may still occur after surgery. Therefore, knowledge of the genes related to these processes and their molecular mechanisms will advance the theoretical and experimental basis for the study and treatment of metastatic tumors.
Although accumulating evidence has shown that ERS signaling, particularly the unfolded protein response (UPR), induces breast cancer invasion, its specific mechanism and specific target molecules have not yet been elucidated. Here, we present a systematic review describing the molecular mechanisms by which ERS induces tumor invasion, with the aim of providing ideas for relevant basic research in the future.
The ER is a complicated, dynamic organelle which coordinates the synthesis, folding and maturation of proteins that are eventually secreted or localized in the ER, Golgi, and lysosomes. Oxidative stress, toxicity, ischemia, viral infections, and deficiencies in glucose and other physical or chemical factors can affect the correct folding of proteins in the ER. ER function is impaired when unfolded or misfolded proteins accumulate, resulting in ERS. Cells possess integrated signaling systems to prevent or respond to ERS, and restore homeostasis and normal ER function, including ER-associated degradation (ERAD), the UPR and apoptosis. ERAD occurs after misfolded or unfolded proteins are transferred to the cytoplasm, ubiquitinated and bound by the 26S proteasome [3]. Autophagy also degrades aggregate misfolded proteins. P62, an autophagy receptor protein, plays a crucial role in targeting the transport of these substances to cellular autophagy machinery for degradation by binding to misfolded proteins in the ER lumen [4].
Cells utilize a series of signal transduction pathways to promote correct protein folding, including protein kinase R-like ER kinase-eukaryotic translation initiation factor 2α (PERK-eIF2α), inositol-requiring enzyme 1a-X-box-binding protein 1 (IRE1-XBP1) and activating transcription factor 6 (ATF6) signaling. These pathways inhibit the production and accumulation of misfolded proteins, accelerate degradation of nonfunctional and toxic proteins, initiate the transcription of ERS-related genes and enhance ER self-repair ability; together, these pathways comprise the UPR. Although ERS and subsequent activation of the UPR is typically considered protective, excessive or persistent ERS plays a role in promoting cell death. When ER functions are severely impaired, caspases are activated to maintain normal mechanisms, eventually resulting in apoptosis. Essential downstream proteins include C/EBP homologous protein (CHOP), c-Jun N-terminal kinase (JNK), and ER-associated caspase-12 [5].
The UPR signaling pathway involves signal transduction between the ER and many organelles, including the nucleus, ribosomes, and the Golgi apparatus. The UPR protects cells undergoing ERS, and under normal conditions, three transmembrane proteins, PERK, IRE1 and ATF6, are bound to ERS receptor glucose-regulated protein 78/binding immunoglobulin heavy chain protein (GRP78/BiP) as a complex to maintain the resting state. During ERS, IRE1, PERK and ATF6 dissociate from GRP78 and then bind to unfolded and misfolded proteins to activate the UPR (Figure 1). IRE1, PERK and ATF6 later dissociate from unfolded or misfolded proteins through the actions of different signaling pathways downstream of the UPR. Ultimately, the dissociation of IRE1, PERK and ATF6 from GRP78 restores the physiological function and activity of the ER.
IRE1 and PERK are categorized as type I transmembrane proteins. After dissociating from GRP78, IRE1 and PERK activity is triggered by dimerization and autophosphorylation of their respective cytoplasmic domains. Homologous dimerization and autophosphorylation activate the ribonuclease activity of IRE1, which then cleaves specific bases from the intron of XBP1 messenger RNA (mRNA), altering the XBP1 mRNA open reading frame [6]. The translated product of cleaved XBP1 mRNA binds ERS response elements and induces the expression of GRP78, CHOP and ER degradation enhancer mannosidase α-like protein, leading to increased degradation of misfolded proteins and recovery of ER function. In addition, dimerization of IRE1 can activate the JNK pathway and the caspase-12 pathways, leading to apoptosis.
Activated PERK phosphorylates and inhibits eIF2α, which reduces protein load in the ER. The inhibition of cyclin D1 translation during ERS, is thought to result in cell cycle arrest [7]. These changes can also promote the expression of apoptosis-related proteins, such as ATF4 and CHOP, which induce ectopic expression of mitochondrial Bax and downregulation of Bcl-2 expression, ultimately inducing apoptosis.
Under ERS, a fraction of ATF6 is translocated from the ER to the Golgi apparatus, where it is then cleaved by site 1 protease and site 2 protease. The cleaved form of ATF6 can then enter the nucleus and upregulate the expression of ER genes associated with protein and lipid synthesis.
The development of malignant tumors is a multistage process involving multiple genes and interactions, and tumor metastasis is a characteristic feature of malignant tumors. Tumor metastasis involves cell proliferation, adhesion, migration, and angiogenesis, as well as degradation of the extracellular matrix (ECM). One of the most important steps in the metastasis process is the dissolution of the basement membrane (BM) and ECM barrier [8], regulated largely by matrix metalloproteinases (MMPs) [9].
In recent years, breast cancer has become one of the major diseases that threatens the health of women. Tumor biology studies suggest that breast cancer metastasis involves the detachment of tumor cells from the primary tumor, infiltration of surrounding tissues, invasion into blood vessels, transport to distant sites, and tumor metastasis in distal organs [1011]. Studies of the mechanism of breast cancer metastasis provide a theoretical basis for the development of drugs that prevent metastasis.
In response to long-term and intense stimuli, the UPR becomes a signal to initiate cell death in order to maintain homeostasis and the biological activity of the organism. Tumor cells adapt to ERS by creating a special microenvironment in which they use unfolded proteins to promote their own development. Solid tumors typically exhibit increased expression of ER chaperones, including GRP78 and GRP94, which are classical UPR markers [12]. The PERK signaling pathway may promote or suppress the occurrence of malignant tumors. PERK signaling may delay the occurrence of breast cancer and reduce the risk of lung cancer metastasis. However, long-term PERK activation may also lead to breast cancer by contributing to gene instability [13]. Long-term ERS-induced expression of CHOP, downstream of PERK signaling, leads to the death of precancerous cells, thus avoiding the formation of malignant tumors. The incidence of lung cancer is significantly increased upon GRP78 knockout in cell, suggesting that CHOP functions as a tumor suppressor [14].
Invasion and metastasis are long-term processes that occur during tumor development. A variety of cancer types, including breast cancer, can exhibit metastasis after radiation and chemotherapy treatments. This persistent invasion and migration is also the major cause of death in patients [1516]. Tumors will always metastasize towards to an environment conducive to survival; however, the mechanisms underlying the association between tumor metastasis and treatment strategies are unclear. UPR-related signaling proteins have recently been shown to affect tumor invasion and migration, processes that require the coordinated regulation of numerous signaling molecules. For example, Li et al. [17] revealed significant reductions in tumor invasion and metastasis following inhibition of ERS-induced heparinase expression in breast cancer cells. In addition, ERS induces C-C motif chemokine ligand 5 (CCL5) expression by upregulating the expression of unphosphorylated signal transducer and activator of transcription 3 (U-STAT3) rather than STAT3 phosphorylation at the Tyr705 site. ERS has also been shown to inhibit CCL5 secretion, leading to decreased transmigration of MCF-7 breast cancer cells [18]. CCL5 induces tumor angiogenesis and the recruitment of various types of stromal cells to primary tumor growth sites, which in turn leads to tumor cell motility, invasion and metastasis. Among other factors, ERS affects tumor metastasis through regulation of GRP78 [16], MMPs [19], epithelialmesenchymal transition (EMT) [20], autophagy [21] and reactive oxygen species (ROS) [22] (Table 1). Thus, studies that elucidate how these signal transduction pathways are mutually regulated and interdependent will be important for improving our understanding of tumor invasion and migration. Investigations of the link between ERS and tumor metastasis may provide new directions for cancer therapies.
GRP78 is a key regulator of ERS signaling and is expressed in a wide variety of organisms, ranging from yeasts to mammals [2324]. The GRP78 protein acts as an ER chaperone, controls protein folding and assembly, prevents protein aggregation, and regulates UPR signaling [252627]. As an important stress receptor, the level of the GRP78 protein is altered by a variety of factors in the tumor microenvironment. GRP78 is also involved in regulating the differentiation, survival, proliferation, apoptosis, invasion, and metastasis of cancer cells.
GRP78 protein levels are increased in breast cancer tissues and have been associated with prognosis, resistance, invasion and metastasis [151628293031]. GRP78 regulates tumor metastasis through the phosphatidylinositol-3-kinase/protein kinase B (PI3K/AKT) pathway. According to Liu et al. [32], GRP78 is involved in the PI3K/AKT pathway by binding to the PI3K subunit, thereby regulating the generation of MAb159, an anti-GRP78 monoclonal antibody. This weakens PI3K/AKT signaling and inhibits tumor growth and metastasis. GRP78 may represent a new biomarker for predicting chemotherapy responsiveness in patients with breast cancer [30].
Conversely, low GRP78 expression may promote tumor invasion and metastasis. GRP78 protein levels in tumor specimens from patients with breast cancer have been used to predict the initial prognosis of patients [33]. Scriven et al. [29] examined the levels of UPR activation markers, GRP78 and XBP1, in 395 human breast cancer specimens using immunohistochemical staining. Expression of GRP78 and XBP1 was seen in 76% and 90% of breast cancer specimens, respectively, which positively correlated with estrogen receptor expression.
Stathmin 1 (STMN1) is a protein involved in cancer metastasis, and upon STMN1 Ser25/Ser38 phosphorylation, GRP78 is a phospho-STMN1-binding protein. The specific interaction between these two proteins has been used to assess the metastatic potential breast cancers [34]. Yuan et al. [35], found that high GRP78 expression in pancreatic cancer activated focal adhesion kinase and JNK pathways and promotes MMP expression, tumor invasion and metastasis. Accordingly, GRP78 knockout in MiaPaCa-2 cells was found to inhibit JNK phosphorylation, reduce MMP expression, activate the RhoA pathway, and induce formation of stress fibers that inhibit the invasion of tumor cells.
MMPs are proteolytic enzymes that participate in tumor invasion and metastasis by employing metal ions, such as Ca2+ and Zn2+, as auxiliary factors to degrade the BM and ECM [36]. High levels of MMP-2 and MMP-9 have been reported to correlate with poor prognosis in breast cancer patients [1937]. In addition, MMP-1 is one of the most frequently upregulated proteins studied in breast cancer metastasis. MMP-1 not only acts as a proteolytic enzyme for digestion of the ECM but also cleaves and activates protease-activated receptor 1, a G-protein coupled receptor that activates intracellular signaling and subsequently promotes tumor metastasis [93839].
In addition, ERS and MMPs have been shown to have an inseparable relationship. For example, hypoxia causes ERS in the MDA-MB-231 breast cancer cell line, stimulating MMP-2 activity and inducing cell invasion and migration [40]. Park et al. [41] showed that tauroursodeoxycholic acid (TUDCA) decreased the expression of MMP-7 and MMP-13 in MDA-MB-231 breast cancer cells by reducing the ERS response, thus indicating important roles for MMP-7 and MMP-13 in tumor metastasis and invasion. Moreover, these findings indicate that ERS is a good target for treatment of cancer metastasis, and that TUDCA may be a good clinical antimetastatic drug candidate.
MMPs are also regulated by other molecules. Based on the results of in vitro and in vivo studies, ATF4 influences tumor cell migration and invasion by modulating MMP-2 and MMP-7 [42]. In the study by Pei et al. [43], the Chinese herbal medicine, plantamajoside, inhibited the proliferation, migration and invasion of MDA-MB-231 human breast cancer cells by inhibiting the activity of MMP-9 and MMP-2. Li et al. [44] found that high expression of human phosphatidylethanolamine-binding protein 4 lead to AKT activation, increasing the expression of MMPs and promoting the invasion and metastasis of breast cancer cells.
During tumor progression, abnormal EMT activation leads to the loss of polarity in epithelial cells. Decreased cell adhesion, increased cellular motility and other biological changes promote the destruction of cell polarity and tight junctions and the aggressiveness of tumor cells. Thus, EMT provides cancer cells with the ability to invade adjacent tissues and form distant metastases [45]. Based on current research findings, breast tumors enhance their invasion and metastasis via EMT [20]. Studies have confirmed that EMT can promote the invasion and metastasis of breast cancer [4647]. ERS is a major factor leading to EMT [48], and ERS induces EMT via a variety of cell signaling pathways [49]. Furthermore, inhibition of AKT and Src kinases has been found to block ERS-induced EMT, as well as tumor cell migration and invasion.
IRE1/XBP1 and PERK can also alter EMT and thus affect tumor metastasis. Li et al. [50] revealed that XBP1 promotes EMT and invasion in breast cancer cells by regulating Snail. Silencing either XBP1 or Snail inhibited EMT-induced cell invasion, indicating that XBP1/Snail is a novel pathway that contributes to the invasion of breast cancer cells. Cubillos-Ruiz et al. [51] showed that lysyl oxidase-like 2 overexpression activates the IRE1-XBP1 pathway, upregulates factors that induce EMT, and further contributes to the aggressiveness and metastasis of tumors. Additionally, Feng et al. [52] reported that PERK-eIF2α signaling is necessary for EMT processes, as well as cell invasion and metastasis. Using mammary epithelial cells, stimulation of EMT-related gene expression by the transcription factor, Twist, was associated with PERK activity, whereas inhibition of PERK activity attenuated cell migration and tumor formation. PERK has been developed as a potential therapy to prevent tumor metastasis by reducing EMT and invasiveness, [51]. ER protein 29 (ERp29) overexpression enhances EMT by downregulating Twist and Slug (SNAI2), activating E-cadherin and degrading fibronectin, thus reducing cell invasion and migration. Activation of the XBP1 pathway in breast cancer cells inhibits ERp29 expression and inhibits EMT, thereby increasing tumor invasiveness [5354]. In breast cancer cells cultured in serum-free medium in an hypoxic environment to mimic the stress state of tumor cells, anterior gradient 2 (AGR2) mRNA expression is significantly elevated [55]. While overexpression of AGR2 has been shown to reduce the adhesion of breast cancer cells, it has also been shown to increase their metastatic potential [56].
In recent years, the role of autophagy in the proliferation and metastasis of breast cancer has caused widespread concern in the medical community [57]. Some molecules or antitumor drugs can affect the proliferation and metastasis of breast cancer cells by regulating autophagy. For example, Bcl-2 promotes the proliferation of MCF-7 breast cancer cells by inhibiting autophagy [58]. Chiavarina et al. [59] reported that hypoxia-inducible factor 1α (HIF-1α) significantly inhibited the proliferation and tumorigenicity of MDA-MB-231 breast cancer cells. Furthermore, the antitumor drug methylenesubstituted 3,3′-diindolylmethane inhibited the proliferation of MDA-MB-231 breast cancer cells and by activating autophagy [60]. Indelicato et al. [40] reported that the autophagy inhibitor, trifluoperazine inhibits breast cancer cell proliferation, migration and invasion. Thus, autophagy appears to promote death and inhibit proliferation and metastasis in breast cancer cells [61].
Regulation of autophagy can promote cell survival or death by affecting ERS [6263]. ERS-induced release of Ca2+ from the ER into the cytoplasm activates various kinases and proteases involved in autophagy signaling [21]. Shimada et al. [64] reported that ERS induces autophagy by activating the mitogen-activated protein kinase signaling pathway. PERK and IRE1 signal transduction pathways are related to the activation of autophagy [21656667]. The PERK pathway is thought to be necessary for autophagy triggered by ERS via phosphorylation of eIF2 and activation of autophagy-related protein 12 [66]. PERK knockdown was recently shown to significantly reduce autophagosome formation [68]. IRE1 links ERS and autophagy by activating the tumor necrosis factor receptor-associated factor 2/c-Jun N-terminal kinase pathway [69]. The induction of ERS, the UPR and autophagy contributes to chemoresistance in breast cancer cells treated with the proteasome inhibitor, bortezomib [70]. Similarly, binding of ceramide to PERK during cancer treatments and promotes prosurvival pathways by activating autophagy [71].
Thus, the development of drugs that inhibit ERS and autophagy may represent an effective method for improving cancer treatment. The ERS-autophagy pathway is particularly important as a new molecular target in cancer therapy. However, both ERS, and subsequently activated autophagy, play dual roles in cell survival/death, making these pathways extremely complex. Therefore, a better understanding of methods to regulate this pathway and effectively treat cancer is necessary and warrants further preclinical and clinical investigation.
ROS are a type of oxygen reduction product produced by cells in the body. ROS, a second messenger, participates extensively in intracellular signal transduction, regulation of cellular responses to external stimuli, determination of cell fate [7273], and participation in physiological processes such as cell proliferation, differentiation, and apoptosis [74]. ROS also play a crucial role in the complex pathology of tumor metastasis. ROS scavengers effectively inhibit tumor cell metastasis [22]. Cannabidiol, a cannabinoid with low toxicity, inhibits the proliferation and invasion of human breast cancer cells by modulating extracellular signal-regulated kinase and ROS levels [75]. Small molecular compounds, such as millepachine and butein, have been shown to induce apoptosis by altering the production of ROS to inhibit the growth and metastasis of breast cancer [7677]. YLT26 inhibits breast cancer cell proliferation through the ROS-mitochondrial apoptotic pathway, slows breast tumor progression, and inhibits lung metastasis by affecting the in vivo immune microenvironment [78]. Therefore, the development of new small molecule compounds that increase intracellular ROS levels provide researchers with a new avenue for investigation in the treatment of malignant tumors.
ROS regulate intracellular signaling, and the balance between ROS production and clearance in cells is important for maintenance of the redox state. Normal physiological function and redox status in the ER are closely related. Moreover, the production of ROS as a by-product of protein folding and oxidative protein folding in the ER are closely linked [79]. Therefore, ROS are likely to be involved in regulating ERS-mediated autophagy and apoptosis. The PERK/eIF2α signaling pathway reduces oxidative stress by inhibiting translation initiation when protein folding in the ER is disrupted, or by inducing the expression of genes involved in antioxidant stress [80]. ROS generation occurs either upstream or downstream of the ER signal; however, the molecular mechanisms involved in this process are not well understood and may be simultaneously regulated by multiple signaling pathways.
Vascular endothelial growth factor (VEGF) protein family members promote tumor cell growth and angiogenesis and inhibit cancer cell apoptosis, which directly or indirectly, promotes tumor metastasis. Inhibition of IRE1 expression reduces tumor cell neovascularization and tumor growth rates, while increasing tumor cell invasion [81]. Moderate activation of PERK signaling acts in an autocrine manner by binding to VEGF receptor 2 to promote migration and invasion [8283]. Downstream of IRE1, XBP1 also plays a role in tumor metastasis. Chen et al. [84] found that in triple-negative breast cancer, the N-terminus bZIP domain of XBP1 interacts with HIF-1α to form a complex. This complex regulates CD44/CD24 by recruiting RNA polymerase II, thus promoting tumor cell invasion.
A recent study identified lysosomal-associated membrane protein 3 (LAMP3), also known as DC-LAMP, TSC-403 and CD208, as a novel hypoxia-induced candidate biomarker of UPR activation and part of the PERK/ATF4 pathway in tumors [85]. Subsequently, Nagelkerke et al. [86] showed that MDA-MB-231 cells grown in hypoxic microenvironments upregulate LAMP3 expression through the PERK/ATF4 pathway, thereby enhancing their ability to metastasize. Similarly, Dey et al. [87] revealed that ATF4 plays an important role in mediating tumor metastasis. ATF3 plays a key role in the coexpression of eIF2 kinase-induced genes in response to ERS and affects cancer cell survival and metastasis by downregulating early growth response protein 1 expression [88]. ATF6 activity increases during ERS, resulting in increased expression of protein tyrosine phosphatase 1B, which promotes the carcinogenesis of breast tissue and is associated with the lung metastasis of breast cancer [899091].
The ER plays an important role in protein synthesis and folding. Accumulation of unfolded or misfolded proteins in the ER leads to ERS and an intracellular steady-state imbalance. The UPR ameliorates ERS through various signaling pathways. ERS is increased during the development and progression of tumors and has a dual regulatory effect on the survival of cells. While ERS and the UPR protect normal cells, they can also induce tumorigenesis and promote the survival and development of tumor cells. Accordingly, PERK, IRE1 and GRP78 have been utilized as new targets for the treatment of tumor metastasis. Despite our preliminary understanding of ERS, knowledge of the role of ERS in tumor metastasis is limited. What other signaling proteins play a role in regulating tumor metastasis during the URP; the precise mechanism by which the UPR promotes cell survival, and transforms tumor metastasis responses; and whether ERS-induced tumor metastasis is related to the occurrence of other diseases are questions that remain to be answered. Understanding of the relationship between ERS and tumor metastasis through studies that explore the regulatory mechanisms involved in signal transduction and gene expression during ERS will be critical to inhibiting tumor cell metastasis, which has become the main goal of tumor therapy.
Notes
References
1. DeSantis C, Ma J, Bryan L, Jemal A. Breast cancer statistics, 2013. CA Cancer J Clin. 2014; 64:52–62. PMID: 24114568.


2. Torre LA, Bray F, Siegel RL, Ferlay J, Lortet-Tieulent J, Jemal A. Global cancer statistics, 2012. CA Cancer J Clin. 2015; 65:87–108. PMID: 25651787.


3. Wang M, Kaufman RJ. Protein misfolding in the endoplasmic reticulum as a conduit to human disease. Nature. 2016; 529:326–335. PMID: 26791723.


4. Yu H, Su J, Xu Y, Kang J, Li H, Zhang L, et al. p62/SQSTM1 involved in cisplatin resistance in human ovarian cancer cells by clearing ubiquitinated proteins. Eur J Cancer. 2011; 47:1585–1594. PMID: 21371883.


5. Li Y, Guo Y, Tang J, Jiang J, Chen Z. New insights into the roles of CHOP-induced apoptosis in ER stress. Acta Biochim Biophys Sin (Shanghai). 2014; 46:629–640. PMID: 25016584.


6. Yoshida H, Matsui T, Yamamoto A, Okada T, Mori K. XBP1 mRNA is induced by ATF6 and spliced by IRE1 in response to ER stress to produce a highly active transcription factor. Cell. 2001; 107:881–891. PMID: 11779464.


7. Harding HP, Zhang Y, Bertolotti A, Zeng H, Ron D. Perk is essential for translational regulation and cell survival during the unfolded protein response. Mol Cell. 2000; 5:897–904. PMID: 10882126.


8. Rodrigues LM, Theodoro TR, Matos LL, Mader AM, Milani C, Pinhal MA. Heparanase isoform expression and extracellular matrix remodeling in intervertebral disc degenerative disease. Clinics (Sao Paulo). 2011; 66:903–909. PMID: 21789398.


9. Kessenbrock K, Plaks V, Werb Z. Matrix metalloproteinases: regulators of the tumor microenvironment. Cell. 2010; 141:52–67. PMID: 20371345.


10. Malek AV, Bershteĭn LM, Filatov MV, Beliaev AM. Exosomal intercellular communication system and its role in the process of metastatic dissemination. Vopr Onkol. 2014; 60:429–436. PMID: 25552061.
11. Steeg PS. Tumor metastasis: mechanistic insights and clinical challenges. Nat Med. 2006; 12:895–904. PMID: 16892035.


12. Lee AS. Glucose-regulated proteins in cancer: molecular mechanisms and therapeutic potential. Nat Rev Cancer. 2014; 14:263–276. PMID: 24658275.


13. Bobrovnikova-Marjon E, Grigoriadou C, Pytel D, Zhang F, Ye J, Koumenis C, et al. PERK promotes cancer cell proliferation and tumor growth by limiting oxidative DNA damage. Oncogene. 2010; 29:3881–3895. PMID: 20453876.


14. Huber AL, Lebeau J, Guillaumot P, Pétrilli V, Malek M, Chilloux J, et al. p58(IPK)-mediated attenuation of the proapoptotic PERK-CHOP pathway allows malignant progression upon low glucose. Mol Cell. 2013; 49:1049–1059. PMID: 23395000.


15. Qin XJ, Ling BX. Proteomic studies in breast cancer (Review). Oncol Lett. 2012; 3:735–743. PMID: 22740985.


16. Gnant M, Balic M, Petru E, Raunik W, Singer CF, Steger GG, et al. Treatment of bone metastases in patients with advanced breast cancer. Breast Care (Basel). 2012; 7:92–98. PMID: 22740794.


17. Li Y, Liu H, Huang YY, Pu LJ, Zhang XD, Jiang CC, et al. Suppression of endoplasmic reticulum stress-induced invasion and migration of breast cancer cells through the downregulation of heparanase. Int J Mol Med. 2013; 31:1234–1242. PMID: 23467544.


18. Zhang Y, Liao S, Fan W, Wei W, Wang C, Sun S. Tunicamycin-induced ER stress regulates chemokine CCL5 expression and secretion via STAT3 followed by decreased transmigration of MCF-7 breast cancer cells. Oncol Rep. 2014; 32:2769–2776. PMID: 25231320.


19. Duffy MJ, Maguire TM, Hill A, McDermott E, O'Higgins N. Metalloproteinases: role in breast carcinogenesis, invasion and metastasis. Breast Cancer Res. 2000; 2:252–257. PMID: 11250717.


20. Trimboli AJ, Fukino K, de Bruin A, Wei G, Shen L, Tanner SM, et al. Direct evidence for epithelial-mesenchymal transitions in breast cancer. Cancer Res. 2008; 68:937–945. PMID: 18245497.


21. Høyer-Hansen M, Bastholm L, Szyniarowski P, Campanella M, Szabadkai G, Farkas T, et al. Control of macroautophagy by calcium, calmodulin-dependent kinase kinase-beta, and Bcl-2. Mol Cell. 2007; 25:193–205. PMID: 17244528.
22. Ishikawa K, Takenaga K, Akimoto M, Koshikawa N, Yamaguchi A, Imanishi H, et al. ROS-generating mitochondrial DNA mutations can regulate tumor cell metastasis. Science. 2008; 320:661–664. PMID: 18388260.


23. Lee AS. The glucose-regulated proteins: stress induction and clinical applications. Trends Biochem Sci. 2001; 26:504–510. PMID: 11504627.


24. Monnerjahn C, Techel D, Meyer U, Rensing L. The grp78 promoter of Neurospora crassa: constitutive, stress and differentiation-dependent protein-binding patterns. Curr Genet. 2001; 39:319–326. PMID: 11525405.


25. Ni M, Lee AS. ER chaperones in mammalian development and human diseases. FEBS Lett. 2007; 581:3641–3651. PMID: 17481612.


26. Little E, Ramakrishnan M, Roy B, Gazit G, Lee AS. The glucose-regulated proteins (GRP78 and GRP94): functions, gene regulation, and applications. Crit Rev Eukaryot Gene Expr. 1994; 4:1–18. PMID: 7987045.


27. Haas IG. BiP (GRP78), an essential hsp70 resident protein in the endoplasmic reticulum. Experientia. 1994; 50:1012–1020. PMID: 7988659.


28. Lee E, Nichols P, Spicer D, Groshen S, Yu MC, Lee AS. GRP78 as a novel predictor of responsiveness to chemotherapy in breast cancer. Cancer Res. 2006; 66:7849–7853. PMID: 16912156.


29. Scriven P, Coulson S, Haines R, Balasubramanian S, Cross S, Wyld L. Activation and clinical significance of the unfolded protein response in breast cancer. Br J Cancer. 2009; 101:1692–1698. PMID: 19861963.


30. Fernandez PM, Tabbara SO, Jacobs LK, Manning FC, Tsangaris TN, Schwartz AM, et al. Overexpression of the glucose-regulated stress gene GRP78 in malignant but not benign human breast lesions. Breast Cancer Res Treat. 2000; 59:15–26. PMID: 10752676.


31. Liang Y, O'Driscoll L, McDonnell S, Doolan P, Oglesby I, Duffy K, et al. Enhanced in vitro invasiveness and drug resistance with altered gene expression patterns in a human lung carcinoma cell line after pulse selection with anticancer drugs. Int J Cancer. 2004; 111:484–493. PMID: 15239124.
32. Liu R, Li X, Gao W, Zhou Y, Wey S, Mitra SK, et al. Monoclonal antibody against cell surface GRP78 as a novel agent in suppressing PI3K/AKT signaling, tumor growth, and metastasis. Clin Cancer Res. 2013; 19:6802–6811. PMID: 24048331.


33. Lee E, Nichols P, Groshen S, Spicer D, Lee AS. GRP78 as potential predictor for breast cancer response to adjuvant taxane therapy. Int J Cancer. 2011; 128:726–731. PMID: 20473863.


34. Kuang XY, Jiang HS, Li K, Zheng YZ, Liu YR, Qiao F, et al. The phosphorylation-specific association of STMN1 with GRP78 promotes breast cancer metastasis. Cancer Lett. 2016; 377:87–96. PMID: 27130664.


35. Yuan XP, Dong M, Li X, Zhou JP. GRP78 promotes the invasion of pancreatic cancer cells by FAK and JNK. Mol Cell Biochem. 2015; 398:55–62. PMID: 25218495.


36. Decock J, Thirkettle S, Wagstaff L, Edwards DR. Matrix metalloproteinases: protective roles in cancer. J Cell Mol Med. 2011; 15:1254–1265. PMID: 21418514.


37. Turpeenniemi-Hujanen T. Gelatinases (MMP-2 and -9) and their natural inhibitors as prognostic indicators in solid cancers. Biochimie. 2005; 87:287–297. PMID: 15781315.


38. Hsu FN, Yang MS, Lin E, Tseng CF, Lin H. The significance of Her2 on androgen receptor protein stability in the transition of androgen requirement in prostate cancer cells. Am J Physiol Endocrinol Metab. 2011; 300:E902–E908. PMID: 21364123.


39. Lynch CC, Hikosaka A, Acuff HB, Martin MD, Kawai N, Singh RK, et al. MMP-7 promotes prostate cancer-induced osteolysis via the solubilization of RANKL. Cancer Cell. 2005; 7:485–496. PMID: 15894268.


40. Indelicato M, Pucci B, Schito L, Reali V, Aventaggiato M, Mazzarino MC, et al. Role of hypoxia and autophagy in MDA-MB-231 invasiveness. J Cell Physiol. 2010; 223:359–368. PMID: 20112292.


41. Park GY, Han YK, Han JY, Lee CG. Tauroursodeoxycholic acid reduces the invasion of MDA-MB-231 cells by modulating matrix metalloproteinases 7 and 13. Oncol Lett. 2016; 12:2227–2231. PMID: 27602168.


42. Zhu H, Chen X, Chen B, Chen B, Song W, Sun D, et al. Activating transcription factor 4 promotes esophageal squamous cell carcinoma invasion and metastasis in mice and is associated with poor prognosis in human patients. PLoS One. 2014; 9:e103882. PMID: 25078779.


43. Pei S, Yang X, Wang H, Zhang H, Zhou B, Zhang D, et al. Plantamajoside, a potential anti-tumor herbal medicine inhibits breast cancer growth and pulmonary metastasis by decreasing the activity of matrix metalloproteinase-9 and -2. BMC Cancer. 2015; 15:965. PMID: 26674531.


44. Li H, Huang F, Fan L, Jiang Y, Wang X, Li J, et al. Phosphatidylethanolamine-binding protein 4 is associated with breast cancer metastasis through Src-mediated Akt tyrosine phosphorylation. Oncogene. 2014; 33:4589–4598. PMID: 24276246.


45. Cardiff RD. Epithelial to mesenchymal transition tumors: fallacious or snail's pace. Clin Cancer Res. 2005; 11(24 Pt 1):8534–8537. PMID: 16361534.
46. Gjerdrum C, Tiron C, Høiby T, Stefansson I, Haugen H, Sandal T, et al. Axl is an essential epithelial-to-mesenchymal transition-induced regulator of breast cancer metastasis and patient survival. Proc Natl Acad Sci U S A. 2010; 107:1124–1129. PMID: 20080645.


47. Oliveras-Ferraros C, Cufi S, Vazquez-Martin A, Torres-Garcia VZ, Del Barco S, Martin-Castillo B, et al. Micro(mi)RNA expression profile of breast cancer epithelial cells treated with the anti-diabetic drug metformin: induction of the tumor suppressor miRNA let-7a and suppression of the TGFbeta-induced oncomiR miRNA-181a. Cell Cycle. 2011; 10:1144–1151. PMID: 21368581.
48. Shah PP, Dupre TV, Siskind LJ, Beverly LJ. Common cytotoxic chemotherapeutics induce epithelial-mesenchymal transition (EMT) downstream of ER stress. Oncotarget. 2017; 8:22625–22639. PMID: 28186986.


49. Zhong Q, Zhou B, Ann DK, Minoo P, Liu Y, Banfalvi A, et al. Role of endoplasmic reticulum stress in epithelial-mesenchymal transition of alveolar epithelial cells: effects of misfolded surfactant protein. Am J Respir Cell Mol Biol. 2011; 45:498–509. PMID: 21169555.
50. Li H, Chen X, Gao Y, Wu J, Zeng F, Song F. XBP1 induces snail expression to promote epithelial-to-mesenchymal transition and invasion of breast cancer cells. Cell Signal. 2015; 27:82–89. PMID: 25280941.
51. Cubillos-Ruiz JR, Bettigole SE, Glimcher LH. Tumorigenic and immunosuppressive effects of endoplasmic reticulum stress in cancer. Cell. 2017; 168:692–706. PMID: 28187289.


52. Feng YX, Sokol ES, Del Vecchio CA, Sanduja S, Claessen JH, Proia TA, et al. Epithelial-to-mesenchymal transition activates PERK-eIF2alpha and sensitizes cells to endoplasmic reticulum stress. Cancer Discov. 2014; 4:702–715. PMID: 24705811.
53. Zhang D, Richardson DR. Endoplasmic reticulum protein 29 (ERp29): an emerging role in cancer. Int J Biochem Cell Biol. 2011; 43:33–36. PMID: 20920593.


54. Bambang IF, Xu S, Zhou J, Salto-Tellez M, Sethi SK, Zhang D. Overexpression of endoplasmic reticulum protein 29 regulates mesenchymalepithelial transition and suppresses xenograft tumor growth of invasive breast cancer cells. Lab Invest. 2009; 89:1229–1242. PMID: 19770839.


55. Zweitzig DR, Smirnov DA, Connelly MC, Terstappen LW, O'Hara SM, Moran E. Physiological stress induces the metastasis marker AGR2 in breast cancer cells. Mol Cell Biochem. 2007; 306:255–260. PMID: 17694278.


56. Liu D, Rudland PS, Sibson DR, Platt-Higgins A, Barraclough R. Human homologue of cement gland protein, a novel metastasis inducer associated with breast carcinomas. Cancer Res. 2005; 65:3796–3805. PMID: 15867376.


57. Berardi DE, Campodónico PB, Díaz Bessone MI, Urtreger AJ, Todaro LB. Autophagy: friend or foe in breast cancer development, progression, and treatment. Int J Breast Cancer. 2011; 2011:595092. PMID: 22295229.


58. Akar U, Chaves-Reyez A, Barria M, Tari A, Sanguino A, Kondo Y, et al. Silencing of Bcl-2 expression by small interfering RNA induces autophagic cell death in MCF-7 breast cancer cells. Autophagy. 2008; 4:669–679. PMID: 18424910.


59. Chiavarina B, Whitaker-Menezes D, Migneco G, Martinez-Outschoorn UE, Pavlides S, Howell A, et al. HIF1-alpha functions as a tumor promoter in cancer associated fibroblasts, and as a tumor suppressor in breast cancer cells: autophagy drives compartment-specific oncogenesis. Cell Cycle. 2010; 9:3534–3551. PMID: 20864819.
60. Vanderlaag K, Su Y, Frankel AE, Burghardt RC, Barhoumi R, Chadalapaka G, et al. 1,1-Bis(3′-indolyl)-1-(p-substituted phenyl)methanes induce autophagic cell death in estrogen receptor negative breast cancer. BMC Cancer. 2010; 10:669. PMID: 21129193.


61. Koren I, Kimchi A. Cell biology: promoting tumorigenesis by suppressing autophagy. Science. 2012; 338:889–890. PMID: 23161981.
62. Schröder M, Sutcliffe L. Consequences of stress in the secretory pathway: the ER stress response and its role in the metabolic syndrome. Methods Mol Biol. 2010; 648:43–62. PMID: 20700704.
63. White E. Deconvoluting the context-dependent role for autophagy in cancer. Nat Rev Cancer. 2012; 12:401–410. PMID: 22534666.


64. Shimada Y, Kobayashi H, Kawagoe S, Aoki K, Kaneshiro E, Shimizu H, et al. Endoplasmic reticulum stress induces autophagy through activation of p38 MAPK in fibroblasts from Pompe disease patients carrying c.546G>T mutation. Mol Genet Metab. 2011; 104:566–573. PMID: 21982629.
65. Zismanov V, Lishner M, Tartakover-Matalon S, Radnay J, Shapiro H, Drucker L. Tetraspanin-induced death of myeloma cell lines is autophagic and involves increased UPR signalling. Br J Cancer. 2009; 101:1402–1409. PMID: 19755988.


66. Kouroku Y, Fujita E, Tanida I, Ueno T, Isoai A, Kumagai H, et al. ER stress (PERK/eIF2alpha phosphorylation) mediates the polyglutamineinduced LC3 conversion, an essential step for autophagy formation. Cell Death Differ. 2007; 14:230–239. PMID: 16794605.
67. Fujita E, Kouroku Y, Isoai A, Kumagai H, Misutani A, Matsuda C, et al. Two endoplasmic reticulum-associated degradation (ERAD) systems for the novel variant of the mutant dysferlin: ubiquitin/proteasome ERAD(I) and autophagy/lysosome ERAD(II). Hum Mol Genet. 2007; 16:618–629. PMID: 17331981.


68. Kim KW, Moretti L, Mitchell LR, Jung DK, Lu B. Endoplasmic reticulum stress mediates radiation-induced autophagy by perk-eIF2alpha in caspase-3/7-deficient cells. Oncogene. 2010; 29:3241–3251. PMID: 20348950.
69. Ogata M, Hino S, Saito A, Morikawa K, Kondo S, Kanemoto S, et al. Autophagy is activated for cell survival after endoplasmic reticulum stress. Mol Cell Biol. 2006; 26:9220–9231. PMID: 17030611.
70. Milani M, Rzymski T, Mellor HR, Pike L, Bottini A, Generali D, et al. The role of ATF4 stabilization and autophagy in resistance of breast cancer cells treated with Bortezomib. Cancer Res. 2009; 69:4415–4423. PMID: 19417138.


71. Park MA, Walker T, Martin AP, Allegood J, Vozhilla N, Emdad L, et al. MDA-7/IL-24-induced cell killing in malignant renal carcinoma cells occurs by a ceramide/CD95/PERK-dependent mechanism. Mol Cancer Ther. 2009; 8:1280–1291. PMID: 19417161.


72. Li Z, Li Z. Glucose regulated protein 78: a critical link between tumor microenvironment and cancer hallmarks. Biochim Biophys Acta. 2012; 1826:13–22. PMID: 22426159.


73. Luo B, Lee AS. The critical roles of endoplasmic reticulum chaperones and unfolded protein response in tumorigenesis and anticancer therapies. Oncogene. 2013; 32:805–818. PMID: 22508478.


74. Scherz-Shouval R, Elazar Z. Regulation of autophagy by ROS: physiology and pathology. Trends Biochem Sci. 2011; 36:30–38. PMID: 20728362.


75. McAllister SD, Murase R, Christian RT, Lau D, Zielinski AJ, Allison J, et al. Pathways mediating the effects of cannabidiol on the reduction of breast cancer cell proliferation, invasion, and metastasis. Breast Cancer Res Treat. 2011; 129:37–47. PMID: 20859676.


76. Wu W, Ye H, Wan L, Han X, Wang G, Hu J, et al. Millepachine, a novel chalcone, induces G2/M arrest by inhibiting CDK1 activity and causing apoptosis via ROS-mitochondrial apoptotic pathway in human hepatocarcinoma cells in vitro and in vivo. Carcinogenesis. 2013; 34:1636–1643. PMID: 23471882.
77. Cho SG, Woo SM, Ko SG. Butein suppresses breast cancer growth by reducing a production of intracellular reactive oxygen species. J Exp Clin Cancer Res. 2014; 33:51. PMID: 24919544.


78. Xiong Y, Ye T, Wang M, Xia Y, Wang N, Song X, et al. A novel cinnamide YLT26 induces breast cancer cells apoptosis via ROS-mitochondrial apoptotic pathway in vitro and inhibits lung metastasis in vivo. Cell Physiol Biochem. 2014; 34:1863–1876. PMID: 25503322.
79. Hung JY, Hsu YL, Ni WC, Tsai YM, Yang CJ, Kuo PL, et al. Oxidative and endoplasmic reticulum stress signaling are involved in dehydrocostuslactone-mediated apoptosis in human non-small cell lung cancer cells. Lung Cancer. 2010; 68:355–365. PMID: 19700217.


80. Marciniak SJ, Yun CY, Oyadomari S, Novoa I, Zhang Y, Jungreis R, et al. CHOP induces death by promoting protein synthesis and oxidation in the stressed endoplasmic reticulum. Genes Dev. 2004; 18:3066–3077. PMID: 15601821.


81. Auf G, Jabouille A, Guérit S, Pineau R, Delugin M, Bouchecareilh M, et al. Inositol-requiring enzyme 1alpha is a key regulator of angiogenesis and invasion in malignant glioma. Proc Natl Acad Sci U S A. 2010; 107:15553–15558. PMID: 20702765.
82. Jamison S, Lin Y, Lin W. Pancreatic endoplasmic reticulum kinase activation promotes medulloblastoma cell migration and invasion through induction of vascular endothelial growth factor A. PLoS One. 2015; 10:e0120252. PMID: 25794107.


83. Lin W, Lin Y, Li J, Harding HP, Ron D, Jamison S. A deregulated integrated stress response promotes interferon-gamma-induced medulloblastoma. J Neurosci Res. 2011; 89:1586–1595. PMID: 21688289.
84. Chen X, Iliopoulos D, Zhang Q, Tang Q, Greenblatt MB, Hatziapostolou M, et al. XBP1 promotes triple-negative breast cancer by controlling the HIF1alpha pathway. Nature. 2014; 508:103–107. PMID: 24670641.
85. Mujcic H, Rzymski T, Rouschop KM, Koritzinsky M, Milani M, Harris AL, et al. Hypoxic activation of the unfolded protein response (UPR) induces expression of the metastasis-associated gene LAMP3. Radiother Oncol. 2009; 92:450–459. PMID: 19726095.


86. Nagelkerke A, Bussink J, Mujcic H, Wouters BG, Lehmann S, Sweep FC, et al. Hypoxia stimulates migration of breast cancer cells via the PERK/ATF4/LAMP3-arm of the unfolded protein response. Breast Cancer Res. 2013; 15:R2. PMID: 23294542.


87. Dey S, Sayers CM, Verginadis II, Lehman SL, Cheng Y, Cerniglia GJ, et al. ATF4-dependent induction of heme oxygenase 1 prevents anoikis and promotes metastasis. J Clin Invest. 2015; 125:2592–2608. PMID: 26011642.


88. Park SH, Kim J, Do KH, Park J, Oh CG, Choi HJ, et al. Activating transcription factor 3-mediated chemo-intervention with cancer chemokines in a noncanonical pathway under endoplasmic reticulum stress. J Biol Chem. 2014; 289:27118–27133. PMID: 25122760.


89. Gu F, Nguyên DT, Stuible M, Dubé N, Tremblay ML, Chevet E. Proteintyrosine phosphatase 1B potentiates IRE1 signaling during endoplasmic reticulum stress. J Biol Chem. 2004; 279:49689–49693. PMID: 15465829.


90. Julien SG, Dubé N, Read M, Penney J, Paquet M, Han Y, et al. Protein tyrosine phosphatase 1B deficiency or inhibition delays ErbB2-induced mammary tumorigenesis and protects from lung metastasis. Nat Genet. 2007; 39:338–346. PMID: 17259984.


91. Bentires-Alj M, Neel BG. Protein-tyrosine phosphatase 1B is required for HER2/Neu-induced breast cancer. Cancer Res. 2007; 67:2420–2424. PMID: 17347513.


Figure 1
Endoplasmic reticulum stress (ERS) and breast cancer metastasis. Glucose-regulated protein 78 (GRP78) dissociates from ERS transducers, including inositol-requiring enzyme 1 (IRE1), protein kinase R-like endoplasmic reticulum kinase (PERK) and activating transcription factor 6 (ATF6), when unfolded or misfolded proteins accumulate in the endoplasmic reticulum lumen. ERS regulates tumour metastasis mainly through matrix metalloproteinases (MMPs), epithelial-mesenchymal transition (EMT), autophagy, reactive oxygen species (ROS), and other transcription factors.
eIF2α=eukaryotic translation initiation factor 2α; XBP1=X-box-binding protein 1; S1P=site 1 protease; S2P=site 2 protease; ATF4=activating transcription factor 4; JNK=c-Jun N-terminal kinase.
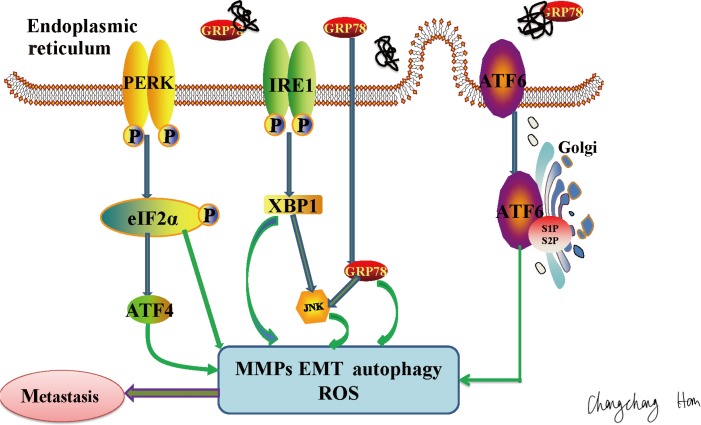
Table 1
Endoplasmic reticulum stress pathways are related to tumor metastasis
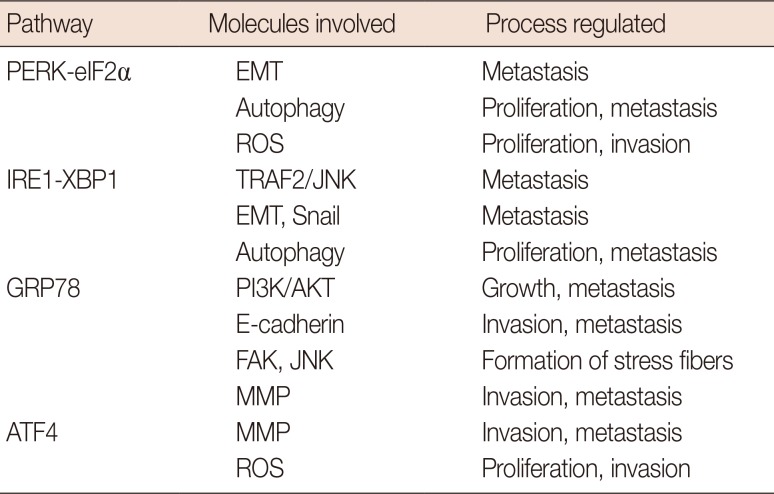
PERK-eIF2α=protein kinase R-like endoplasmic reticulum kinase-eukaryotic translation initiation factor 2α; EMT=epithelial-mesenchymal transition; ROS=reactive oxygen species; IRE1-XBP1=inositol-requiring enzyme 1a-X-box-binding protein 1; TRAF2/JNK=tumor necrosis factor receptor-associated factor 2/c-Jun N-terminal kinase; GRP78=glucose-regulated protein 78; PI3K/AKT=phosphatidylinositol-3-kinase/protein kinase B; FAK=focal adhesion kinase; MMP=matrix metalloproteinase; ATF4=activating transcription factor 4.