Abstract
Objective
This study was performed to explore the effect of different bracket, archwire, and ligature combinations on resistance to sliding (RS) and rotational control in first-order angulation.
Methods
Three types of brackets (multi-level low friction [MLF], self-ligating, and conventional brackets) coupled with four nickel-titanium archwires (0.012, 0.014, 0.016, and 0.018-inch diameter) and two stainless steel ligatures (0.20 and 0.25 mm) were tested in different first-order angulations (0°, 2°, 4°, 6°, 8°, 10°, 15°, 20°) by using an Instron universal mechanical machine in the dry state at room temperature. RS value was evaluated and compared by one-way ANOVA.
Results
Under the same angulation, the RS values showed the following order: conventional brackets > MLF brackets > self-ligating brackets. The RS was the highest for conventional brackets and showed a tendency to increase. The RS for MLF brackets coupled with thinner archwires and ligatures showed a similar tendency as the RS for the self-ligating bracket. In contrast, the RS for MLF brackets coupled with thicker archwires and ligatures increased like that for conventional brackets. MLF brackets showed the greatest range of critical contact angles in first-order angulation.
Managing friction in clinical settings is important in orthodontic practice because friction is a constant factor when sliding mechanics are employed in tooth movement.1 Kusy and Whitley23 illustrated the resistance to sliding (RS) by partitioning it into three parts: classical friction, binding, and notching. Each of these parts dominates depending on whether the contact angle (θ) is less or more than the critical contact angle (θc). In second-order angulation, θ is the angle between the edge of the slot and the archwire, while θc is the boundary angle when the archwire is just in contact with both the superior and inferior edges of the slot (panel a in Figure 1A).23 In turn, the contact angle of first-order angulation (α) is the angle between the slot and the ligature wire (panel b in Figure 1A).
However, few studies have attempted to evaluate the RS of first-order angulations even though low friction and rotational control are equally important factors during the alignment and leveling stages. Self-ligating brackets have become popular in the last decade since they were reported to show low friction and secure archwire engagement,4 but studies have concluded that self-ligating brackets are no more efficient than conventional brackets for the purpose of alignment.5678910 This discrepancy may be explained by axial rotational control because low friction may occasionally offset derotation capacity. Thus, in most cases, reduction of friction and enhancement of rotational control would involve addressing one factor and losing control of the other.
MLF brackets, as a component of the physiologic anchorage spee-wire system technique, possess a specially constricted cervical design that prevents the ligature from compressing the archwire, when used with thinner archwires, and facilitates derotation when coupled with thicker archwires or ligatures (Figure 1B).1112 In this study, the RS in first-order angulations with different combinations of bracket, archwire, and ligature was evaluated in order to explore the relationship between rotational control and treatment efficiency in MLF, self-ligating, and conventional brackets.
Three types of brackets for the upper right canine were selected; self-ligating bracket (Figure 1C) (Shinye, Hangzhou, China), MLF bracket (Figure 1D) (Shinye), and conventional bracket (Figure 1E) (Shinye). All of the slots were 0.022 × 0.028 inches in size. Four nickel-titanium (NiTi) archwires (0.012, 0.014, 0.016, 0.018-inch diameter, superelastic NiTi; Shinye) and two steel ligatures (0.20 and 0.25 mm) were used in this study.
The RS values were evaluated by a custom-built testing apparatus that was fixed on a universal mechanical machine (Instron3367; Instron Corp., Kawasaki, Japan). A computer-controlled motor was connected to the apparatus. A universal bevel protractor was used as the carrier for bracket bonding and angle control. The testing brackets were bonded to the upper side of the universal bevel protractor and the mesial-distal direction of the slot was supposed to be parallel to the plump line direction (Figure 2A). With changes in the angulation, the component that held the brackets was moved horizontally backward to avoid deviations caused by the bonding height on angulation expression. The displacement of the horizontal movement was calculated by a trigonometric function (Figure 2B).
The upper clamp of the Instron machine was clamped on the upper side of the archwire and the other side was permanently connected with a section of the spring in order to allow the archwire to rise freely along the direction of the slot. Two tie-wires (0.20 and 0.25 mm) were coupled with MLF brackets, and one tie-wire (0.25 mm) was used for ligation with the conventional brackets.
The drawing force was measured using the load cell of the Instron machine with the rotational angle set in the following order: 0°, 2°, 4°, 6°, 8°, 10°, 15°, and 20°. For each combination of bracket, archwire, and ligature, eight drawing forces were measured. The drawing force of the spring was measured in advance to eliminate the effect of the force generated by the spring. Thus, a total of 144 drawing forces with 16 brackets, 16 archwires, and 16 springs were measured in the study. For each assessment, the sliding velocity of the archwire was fixed at 5 mm/minute for a displacement of 7 mm. All measurements were obtained in the dry state at room temperature.
The findings for each combination of bracket, archwire, ligature at a particular angle were recorded as descriptive statistics with means and standard deviations of the RS value calculated by software (IBM SPSS Statistics ver. 23; IBM Corp., Armonk, NY, USA). The obtained statistics were ranked, and the one-way ANOVA test was used to study the difference between different brackets with the same archwire.
The RS values of different bracket–archwire–ligature combinations with MLF brackets, self-ligating brackets, and conventional brackets at each first-order angulation are shown in Tables 1, 2, and 3. Barring some minor fluctuations, the RS value for the same archwire increased with the angulation when no clearance existed.
The RS values of MLF brackets with 0.012-inch archwires showed a flat curve (Figure 3A) even with changes in angulation. With an increase in the dimensions of the archwires and the ligatures, the turning points of the RS values appeared earlier. The RS values also showed a slightly higher increase when coupled with 0.25-mm ligatures for the same archwire (Table 1).
When coupled with 0.012-inch NiTi archwires, the MLF and self-ligating brackets showed RS values with minor variations, except for some fluctuations. The RS values tended to increase rapidly with 15° angulation for MLF brackets combined with two ligatures while the RS values of self-ligating brackets with 0.014-inch NiTi archwires remained stable. With 0.016-inch NiTi archwires, RS values for both MLF and self-ligating brackets exhibited a tendency to increase at 10° to 15° angulation. When coupled with 0.018-inch NiTi archwires, the RS values of MLF brackets increased at the beginning of the angulation changes. Meanwhile, the RS values of self-ligating brackets showed a more distinct increasing trend compared to those with the previous three archwires, and the turning point of the curve was between 5° and 10°. Compared to the RS values of MLF and self-ligating brackets, the RS values of conventional brackets tended to increase obviously as the angulation gradually increased (Figure 4).
One-way ANOVA tests were used to compare the differences in RS values among combinations of different brackets with the same archwire (Table 4). The post-hoc test demonstrated that when coupled with 0.012-inch NiTi archwires, the RS values of MLF, self-ligating, and conventional brackets differed from each other (p < 0.05), while the MLF brackets showed similar RS values even with different ligatures (p > 0.05). When coupled with 0.014- and 0.016-inch NiTi archwires, self-ligating and MLF brackets displayed similar RS values whereas the conventional brackets showed significantly larger RS values (p < 0.05). When coupled with 0.018-inch NiTi archwires, the RS values of self-ligating brackets and MLF brackets with 0.20-mm ligatures reveal similar values. The conventional brackets showed the largest values (p < 0.05). The results indicated that when combined with the same archwire, the RS values of these three brackets were in the following order; conventional brackets > MLF brackets > self-ligating brackets.
Friction has been studied in numerous situations, including several classical studies on the second-order critical contact angle. Kusy and Whitney2 determined the formula for calculating the critical contact angle (θc) of second-order angulation to facilitate studies on the relationship between the RS and tooth movement. The bracket design, archwire, and ligation method were regarded as key factors affecting RS.31314 Other studies have not confirmed the superiority of self-ligating brackets in treatment efficiency.5678910 Since rotational control is also a critical part in the first stage of treatment, in which the deflection of the archwire plays an important role,15 friction is no longer the only factor that affects the treatment efficiency, and these assessments should also at least include rotational control. The aim of our study is to investigate the effect of rotational control on treatment efficiency.
The ligation acting on the archwire generates a couple to accomplish rotational control.16 Therefore, although the binding is hardly affected by the ligation method in second-order angulation,1718 ligation was assessed in this study as one of the vital factors affecting the RS in first-order angulation.
Based on the design of the MLF bracket noted above, the assumption that a thinner ligature could be chosen to decrease the RS while a thicker one could be used to strengthen rotational control was proposed. Nevertheless, in this study, the mean comparison indicated minor differences between the 0.20- and 0.25-mm steel ties (p > 0.05). Even so, the variations in the RS values between the two ligature groups were worth discussing.
The difference between the two steel wires coupled with 0.012, 0.014, and 0.016-inch NiTi archwires was 2.625 gF, 3.000 gF, and 2.625 gF, respectively. The difference increased to 6.625 gF when the steel wires were coupled with the 0.018-inch NiTi archwire. This could be because archwire sizes up to 0.018-inch coupled with a 0.25-mm ligature will only eliminate the clearance.19 This aspect might have generated the minor but different biological responses in clinical settings. Combining the data shown in Tables 1, 2, 3, the RS values of different steel ties coupled with 0.012-, 0.014-, and 0.016-inch NiTi archwires produced minimal difference from 0° to 15° rotation, while the RS values of different steel ties coupled with the 0.018-inch NiTi archwire produced distinctive values throughout 0° to 20°. If the formula for the critical contact angle in second-order angulation was analogously applied to the first-order angulation, one may speculate that the RS value would be similar when the angulation is below the critical contact angle, and that the 0.25-mm steel ties will allow the system to more easily achieve or exceed the critical binding angle. In addition, the apparent distinction that appeared in the 0.018-inch group was consistent with the findings of a previous study.19
Many studies have concluded that the elastomeric ligature results in greater friction than steel tying.16172021 Based on the ligation characteristics of MLF brackets, it could be inferred that steel tying should be considered as a subjective ligation technique according to various tooth movement targets. Different ligations such as the round tie, figure 8 tie, and diagonal tie also affect the friction.2021 The single ligation could serve to decrease the RS value by increasing the inter-bracket distance (Figure 5A). Likewise, light or tight tying with thinner or thicker ties, to some extent, may indicate the dominance of the passive or active configuration. Thus, since the dental arch represents a system where the components mutually influence each other, even in combination with an initial thin archwire, a thicker ligation could decrease the clearance in some positions. Similarly, thinner ligation could be used to decrease the RS in some positions even when thicker archwires are placed (Figure 5B). A thin ligature and archwire combination with MLF brackets is just like a “loosely tied stainless steel ligature” with a conventional twin wings bracket and produces negligible or the least friction.1822
The analysis of RS in first-order angulation is inspired by studies based on the second-order theory.2324 Similar to the second-order angulation, we believe that the critical contact angle also exists in first-order angulation (αc). Thus, αc might correlate with the size of an archwire, the width of a bracket, and the depth of the slot. Moreover, the depth of the slot may be determined by the geometric parameters of the slot and the size of the ligature (Figure 1).
In all situations, the variations in RS values tended to generally increase as the angulation increased without clearance. The differences were observed in the variation range and the critical contact angle values of the different combinations. This study found that the RS values of self-ligating brackets slowly increased. Even when the 0.018-inch NiTi archwires were placed, the variation curve remains flat till the angulation is increased to 15°. Bednar and Gruendeman16 found that the self-ligated Orec SPEED bracket produced a small moment from 2.5° to 15° rotation and a greater moment over 20° rotation. Miles8 and Miles et al.9 compared the Smart-clip and Damon 2 brackets with conventional brackets after initial alignment and found that the self-ligating brackets had a 0.2–0.7-mm irregularity because of the rotational play. Fleming et al.10 used the peer assessment rating (PAR) index to compare the treatment effect of Smart-clip and conventional brackets. Although the findings showed no statistical significance, the PAR score reduction was still lower in the self-ligating bracket group. These similar phenomena might indicate that the clearance of first-order angulation was usually greater in self-ligating brackets than in conventional brackets. In other words, the self-ligating bracket is not sensitive to subtle rotation. The clearance somewhat impedes the rotational control without an active control, so the derotation will not be realized until the rotational angulation is larger than αc. O'Reilly et al.25 concluded that most clinical situations involve binding and releasing phenomena rather than true friction. Thus, a low RS value is not the only target in orthodontic treatment.
The findings showed one setpoint of angulation after which the curve transformed from comparatively smooth to obviously steep (Figure 6). This setpoint could be considered as the critical contact angle in first-order angulation. Due to the particularity of the first-order direction, each combination of bracket, archwire, and ligation ought to correspond with one setpoint. The range of setpoints for each bracket represents its derotation ability. The turning points of the curves for self-ligating brackets were shown to be mostly concentrated in the range of 10° to 15°. The conventional brackets had few setpoints because the steel ties completely tied the archwires with no passive configuration. The MLF brackets showed the largest range of setpoints under different combinations. This could be because the contact angle for these brackets could always change with ligations and angulations. In addition to 0.20- and 0.25-mm steel ties, other ligature sizes could be chosen to realize different treatment goals. Due to its design, critical contact angle in first-order angulation on each tooth can be adjusted by clinicians even on the same archwire, and theoretically, with countless ligatures, there would be countless critical contact angles as well. Thus, the α could be stabilized at α≈αc, which would be the most efficient and effective status.26
The limitations of our study are inevitable. First, in contrast to the θc in second-order angulation, the calculation formula for αc in first-order angulation is more difficult to compute due to the complex structure of the irregular bracket cover or the uncertainty of the ligating position. Hence, the theoretical αc was not employed in this study. The conclusions were based more on empirical results instead of theoretical calculations. Second, the complexity of biomechanics is an inherently unsurmountable problem for in-vitro studies, necessitating further studies or clinical research to improve the understanding of this topic.
In summary, exploration of first-order angulation is more likely to demonstrate that irrespective of the archwire that is inserted in a complete dental arch, in which sliding and rotational control are simultaneously needed, choosing an appropriate ligation method can help orthodontists more efficiently align and level the teeth in the first stage of the treatment. Since the complexity of biomechanical tooth movement could not be completely imitated in an in-vitro study, clinical trial should be carried out to verify the theoretical hypothesis. This process should be more detailed than merely deciding which bracket is the best or advertising for any manufacturer. Indeed, this should involve continuous introspection based on previous clinical and scientific experience so as to more thoroughly meet the orthodontic goal.
1. The RS in first-order angulation is affected by rotation angle, bracket design, size of the archwires, and the ligations. The RS value increases as the rotational angle increases when no clearance exists. Differences in bracket design, especially the design of the tie wings, influence the tightness of ligation and stretching force.
2. Differences in the choice of archwires and ligatures can change the clearance in first-order angulation, and might affect the range of the setpoints and rotational control for tooth movement. MLF brackets coupled with different archwires and ligations might achieve low RS and rotational control so as to improve the efficiency of orthodontic treatment.
Figures and Tables
Figure 1
A, a: Critical contact angle (θc) of second-order angulation; b: Critical contact angle of first-order angulation (αc). B, a: Traditional ligation method for a conventional bracket; b: A constricted cervical area of the multi-level low friction (MLF) bracket; c: The design of the MLF bracket can hold the ligature and keep it from compressing the archwire. Self-ligating bracket (C), MLF bracket (D), and conventional bracket (E) under a light microscope.
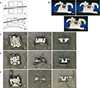
Figure 2
Mechanical apparatus. A, a: Instron machine; b: The testing bracket bonded on the upper side of the universal bevel protractor with the mesial-distal direction of the slot parallel to the plump line direction; c: The lateral view of the universal bevel protractor. The scale was used to regulate the angulation; d: The horizontal scale was used to measure the length that could counterbalance the deviation. B, The trigonometric function used to avoid the deviation by bonding height. A point was the midpoint of the bracket. When the rotational angle was set, the bracket not only rotated but also moved downward and forward. AB was the horizontal displacement and perpendicular to BC, while the vertical displacement could be neglected as it did not influence the findings. AB = sin∠ACB × AC. The brackets were moved backward previously to compensate for the horizontal error.
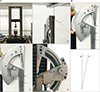
Figure 3
A, The resistance to sliding (RS) variation tendency of multi-level low friction (MLF) brackets. B, The RS variation tendency of self-ligating brackets (SLB). C, The RS variation tendency of conventional brackets (CON).
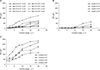
Figure 4
Comparison of different resistance to sliding (RS) variation tendencies for different brackets when coupled with the same archwire.
MLF, Multi-level low friction brackets; SLB, self-ligating brackets; CON, conventional brackets.
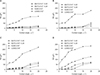
Figure 5
A, Single and full ligations of multi-level low friction (MLF) bracket in first-order angulation view. Characteristic alignment in first-order angulation by MLF and self-ligating brackets (B, MLF brackets; C, self-ligating brackets). The rotation of incisors (blue and red dots) is difficult to correct with self-ligating brackets because of the rotational play. The upper right canine (yellow dot) is ligated with a single ligation of the MLF bracket so as to reduce the RS for better lateral incisor (green dot) correction. MLF brackets could achieve low resistance to sliding and rotational control simultaneously by different ligation combinations.
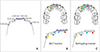
Figure 6
The range of setpoints (the dotted line areas), the collection of turning points of each curve, represents the span of rotational control. A, Multi-level low friction (MLF) brackets; B, self-ligating brackets (SLB); C, conventional brackets (CON).
RS, Resistance to sliding.
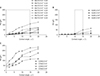
Table 1
Resistance to sliding values (gF) of multi-level low friction brackets combined with different archwires and ligatures
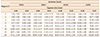
Table 2
Resistance to sliding values (gF) of self-ligating brackets combined with different archwires
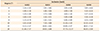
Table 3
Resistance to sliding values (gF) of conventional brackets combined with different archwires and 0.25-mm ligature
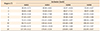
ACKNOWLEDGEMENTS
We thank the Shinya Company for donated materials including NiTi archwires, stainless steel wires and brackets. We also appreciate the help from Professor Hong Lin and researcher Wei Bai in Dental Material Research Laboratory of Peking University and Hospital of Stomatology for providing the equipments and guide of using mechanical apparatus.
Notes
References
1. Kusy RP, Whitley JQ. Friction between different wire-bracket configurations and materials. Semin Orthod. 1997; 3:166–177.

2. Kusy RP, Whitley JQ. Influence of archwire and bracket dimensions on sliding mechanics: derivations and determinations of the critical contact angles for binding. Eur J Orthod. 1999; 21:199–208.


3. Kusy RP, Whitley JQ. Resistance to sliding of orthodontic appliances in the dry and wet states: influence of archwire alloy, interbracket distance, and bracket engagement. J Biomed Mater Res. 2000; 52:797–811.


5. Chen SS, Greenlee GM, Kim JE, Smith CL, Huang GJ. Systematic review of self-ligating brackets. Am J Orthod Dentofacial Orthop. 2010; 137:726.e1–726.e18. discussion 726-7.


6. Fleming PS, Johal A. Self-ligating brackets in orthodontics. A systematic review. Angle Orthod. 2010; 80:575–584.

7. Ong E, McCallum H, Griffin MP, Ho C. Efficiency of self-ligating vs conventionally ligated brackets during initial alignment. Am J Orthod Dentofacial Orthop. 2010; 138:138.e1–138.e7. discussion 138-9.


8. Miles PG. Smartclip versus conventional twin brackets for initial alignment: is there a difference? Aust Orthod J. 2005; 21:123–127.

9. Miles PG, Weyant RJ, Rustveld L. A clinical trial of Damon 2 vs conventional twin brackets during initial alignment. Angle Orthod. 2006; 76:480–485.

10. Fleming PS, DiBiase AT, Lee RT. Randomized clinical trial of orthodontic treatment efficiency with self-ligating and conventional fixed orthodontic appliances. Am J Orthod Dentofacial Orthop. 2010; 137:738–742.


11. Chen S, Chen G, Xu T. Clinical application of the PASS technique. J Clin Orthod. 2015; 49:508–515.

12. Ko CC, Chen S, Zang H. Mechanical properties of various archwires and their clinical application in the PASS system. In : Xu TM, editor. Physiologic Anchorage control: a new orthodontic concept and its clinical application. New York: Springer;2017. p. 71–86.
13. Nanda R, Ghosh J. Biomechanical considerations in sliding mechanics. In : Nanda R, editor. Biomechanics in clinical orthodontics. Philadelphia: Saunders;1997. p. 188–217.
14. Pizzoni L, Ravnholt G, Melsen B. Frictional forces related to self-ligating brackets. Eur J Orthod. 1998; 20:283–291.


15. Rinchuse DJ, Miles PG. Self-ligating brackets: present and future. Am J Orthod Dentofacial Orthop. 2007; 132:216–222.


16. Bednar JR, Gruendeman GW. The influence of bracket design on moment production during axial rotation. Am J Orthod Dentofacial Orthop. 1993; 104:254–261.


17. Thorstenson GA, Kusy RP. Effect of archwire size and material on the resistance to sliding of self-ligating brackets with second-order angulation in the dry state. Am J Orthod Dentofacial Orthop. 2002; 122:295–305.


18. Thorstenson GA, Kusy RP. Comparison of resistance to sliding between different self-ligating brackets with second-order angulation in the dry and saliva states. Am J Orthod Dentofacial Orthop. 2002; 121:472–482.


19. Jiang R, Teng F, Du F, Chen H, Xu TM. Drift dentition and low-friction appliance. In : Xu TM, editor. Physiologic Anchorage control: a new orthodontic concept and its clinical application. New York: Springer;2017. p. 103–120.
20. Bednar JR, Gruendeman GW, Sandrik JL. A comparative study of frictional forces between orthodontic brackets and arch wires. Am J Orthod Dentofacial Orthop. 1991; 100:513–522.


21. Suryawanshi GR, Sundareswaran S, Philip K, Kumar S. In vitro evaluation of different methods of ligation on friction in sliding mechanics. Orthodontics (Chic.). 2013; 14:e102–e109.


22. Hain M, Dhopatkar A, Rock P. The effect of ligation method on friction in sliding mechanics. Am J Orthod Dentofacial Orthop. 2003; 123:416–422.


23. Yeh CL, Kusnoto B, Viana G, Evans CA, Drummond JL. In-vitro evaluation of frictional resistance between brackets with passive-ligation designs. Am J Orthod Dentofacial Orthop. 2007; 131:704.e11–704.e22.


24. Pesce RE, Uribe F, Janakiraman N, Neace WP, Peterson DR, Nanda R. Evaluation of rotational control and forces generated during first-order archwire deflections: a comparison of self-ligating and conventional brackets. Eur J Orthod. 2014; 36:245–254.


25. O'Reilly D, Dowling PA, Lagerstrom L, Swartz ML. An ex-vivo investigation into the effect of bracket displacement on the resistance to sliding. Br J Orthod. 1999; 26:219–227.