Abstract
PURPOSE
This study aimed to assess the effect of non-thermal plasma on the shear bond strength of resin cements to polyetherketoneketone (PEKK) in comparison to other surface treatment methods.
MATERIALS AND METHODS
Eighty PEKK discs were subjected to different surface treatments: (1) Untreated (UT); (2) Non-thermal plasma (NTP); (3) Sandblasting with 50 µm Al2O3 particles (SB); and (4) Sandblasting + Non-thermal plasma (SB+NTP). After each surface treatment, the contact angle was measured. Surface conditioning with Visio.Link was applied in all groups after pre-treatment. RelyX Unicem resin cement was bonded onto the PEKK specimens. After fabrication of the specimens, half of each group (n=10) was initially tested, while the other half was subjected to thermocycling (5℃ to 55℃ at 10,000 cycles). Shear bond strength (SBS) testing was performed using a universal testing machine, and failure modes were assessed using stereomicroscopy. The SBS results were analyzed statistically using one-way ANOVA followed by Tukey's post hoc test. Independent t-test was used to examine the effect of thermocycling (P<.05).
Polyaryletherketone (PAEK) is an advanced thermoplastic resin with both ether and ketone linkages in its chains. Different ratios and sequences of keto groups affect the rigidity and melting temperature of the polymer.1 The chemical structure of polyaromatic ketones provides good dimensional stability, biological inertness, high chemical and mechanical resistance to wear, and excellent toughness and fatigue properties.12 Polyetheretherketone (PEEK) is considered to be the most popular resin among the PAEK family. In medicine, PEEK is considered to be an advanced biomaterial that serves as an alternative implant material for titanium in orthopedic applications, trauma management, and spinal cord implants.34 Initial efforts have been made in dentistry for the usage of PEEK materials, specifically as temporary implant abutment, implant-supported bar or clasps, and dental clasps or frameworks for removable dental prostheses.5
Meanwhile, newly introduced polyetherketoneketone (PEKK) has a wide range of potential uses in dentistry due to its favorable chemical and mechanical properties. In comparison with PEEK materials, PEKK exhibits stronger solidification of the glass and polymer chains due to the high ratio of the keto group. This results in a higher melting point and 80% greater compressive strength.5 Due to its aforementioned features, good biocompatibility, and wide range of fabrication processes, the resin can be a suitable material of choice for crowns, fixed partial dentures, removable partial dentures, and frameworks for implant-supported prostheses.56 In addition, according to the finite analysis in a recent study, PEKK has high potential as a post and core material due to its favorable stress distribution.7 However, the grayish or whitish color and low translucency of the PAEK material limit its use as a monolithic dental restoration. Therefore, additional veneering is essential to obtain satisfactory esthetic results.8 Improved adhesive properties on resin cements are important for reduction of microleakage, enhanced retention, and improved prosthesis stability.9
Despite its numerous advantages, the PAEK group has difficulty forming strong and durable adhesion to resin materials due to its low surface energy and surface modification resistance.10 Non-thermal plasma (NTP) is considered to be an alternative surface treatment option to enhance the surface chemistry of the polymer without altering the bulk properties of these materials after treatment.11 NTP contains partially ionized cold gases that are commonly used for cleaning and surface activation by providing chemically active elements such as O3, OH, H2O2, NO, and OH radicals at a low temperature.12 Therefore, plasma treatment may offer an additional method for improving the wettability and surface energy for polymer materials, which can improve the bond strength to resin materials. According to earlier studies, plasma pre-treatment can be an effective way to improve the adhesive properties of PEEK.13 A recent study reported that argon plasma treatment significantly increased the shear bond strength of PEEK to composite materials (RelyX and SE BOND/Clearfil AP-X).9 However, studies on the effect of non-thermal plasma on PAEK materials are rare and limited to PEEK materials.
Therefore, this study investigated the effect of non-thermal plasma on the shear bond strength of resin cements to PEKK in comparison to other surface treatment methods. The effect of thermocycling among the groups was also evaluated. The null hypothesis was that NTP surface treatment provides no improvement in shear bond strength of resin cements to PEKK material.
This experimental study was conducted using 80 PEKK discs (Pekkton Ivory, Cendres+Métaux, Biel/Bienne, Switzerland) that measuresd 7 × 7 × 2 mm each. Subsequently, each PEKK disc was embedded in an auto-polymerizing acrylic resin (Polycoat, Aekyung Chemical Co., Daejeon, Korea). The bonding surfaces were polished using 200-grit up to 600-grit silicon carbide abrasive paper under water cooling for initial roughness standardization. The surfaces were cleaned in an ultrasonic water bath for 10 minutes and air-dried prior to any surface treatment procedures. The discs were randomly divided into 4 experimental groups consisting of 20 specimens according to surface treatment and are summarized in Table 1. Sandblasting was conducted using 50 µm alumina particles (LEMAT NT4, Wassermann, Germany) at a pressure of 0.5 MPa and a distance of 10 mm perpendicular to the treated surface. After sandblasting, the specimens were again subjected to an ultrasonic cleaner for 10 minutes prior to bonding. For non-thermal plasma treated specimens, low density air plasma was applied for 5 minutes at a distance of 10 mm. The application of Visio. link was performed immediately after every NTP treatment followed by resin cement. This procedure prevented the loss of plasma activation on the treated surface. All materials used is summarized in Table 2.
The evaluation of wettability was performed by fabricating six additional specimens for each surface treatment group. Static contact angle measurements were conducted using a goniometer machine (Phoenix 300 Touch, SEO, Suwon, Gyeonggi, Korea). The predetermined droplet was dispensed using a syringe attached above the specimen stage, and 0.5 µL of distilled water was dropped onto the center of each specimen. The droplet arc and contact angle at the interface were measured and recorded by the machine's software, 10 seconds after the injection.
After the different surface treatment methods were completed, Visio. link (Bredent, Senden, Germany) was applied to the treated PEKK surface and light cured for 90 seconds. A cylindrical plastic mold with an internal diameter of 3 mm and thickness of 2 mm was placed on the surface of the specimen (Fig. 1A). Self-adhesive resin cement RelyX Unicem (3M ESPE, Seefeld Germany) was applied on the tube and light cured using a Smart light LED curing light (Dentsply DeTrey, Konstanz, Germany) for 40 seconds. After the bonding procedures, half of each group (n = 10) were stored in distilled water at 37℃ for 24 hours before bond strength testing, while the other half of the specimens (n = 10) were subjected to thermocycling of 10,000 cycles with temperatures changing from 5℃ to 55℃.
The shear bond strength (SBS) test was performed using a universal testing machine (AG-10KNX, Shimadzu Co., Kyoto, Japan) at room temperature. A knife edge-shaped apparatus was used for testing at a crosshead speed of 1 mm/min. The average shear bond strength was calculated with the formula F = N/A2, where (F) represents the shear bond strength (in MPa), (N) is the maximum force exerted on the specimen, and (A) is the size of the bonding area (in mm2) (Fig. 1B).
Failure types were examined and classified into three groups: (a) Adhesive (failure at the connection between resin cement and PEKK), (b) Cohesive (failure inside the resin cement), and (c) Mixed (adhesive and cohesive). All failure types were assessed under a stereomicroscope (Damisystem, TaeShin BioScience, Namyangju, Korea) by one trained examiner. All experimental procedures are summarized in Figure 2.
The normality of data distribution was verified using Kolmogorov-Smirnov and Shapiro-Wilk tests. To determine the effects of different surface treatments on the wettability and shear bond strength for both thermocycling and non-thermocycling groups, one-way ANOVA followed by Tukey's post hoc was used. To examine the impact of thermocycling on the shear bond strength after surface treatment, the independent t-test was used (P < .05). All statistical tests were performed using SPSS version 22.0 (IBM, Armonk, NY, USA).
After shear bond testing, the UT group (control) exhibited the lowest SBS values, while the combination effect of SB + NTP showed significantly higher SBS values in all the experimental groups (UT < NTP < SB < SB + NTP, P < .05) (Fig. 3). After thermocycling, SBS+NTP showed significantly higher shear bond strength compared to the UT and NTP groups (P < .05) (Fig. 3). In addition, artificial aging significantly reduced the SBS value in the UT (P = .024), NTP (P = .001), and SB + NTP groups (P = .01) (Table 3).
The mean values (± SD) and images for contact angle (CA) measurements after different surface treatments are shown in Fig. 4. The UT group exhibited the highest contact angle, while the SB + NTP had the lowest contact angle among the groups. Failure mode analysis revealed that the UT and NTP groups showed predominantly adhesive failures, while mixed failures were observed in the SB and SB+NTP groups regardless of thermocycling. Although rare, cohesive failure was observed for groups treated with SB (20%) and SB + NTP (30%) (Fig. 5).
The surface topography and chemical nature of PEKK are both important in improving its bond strength to resin materials. Previous studies investigated the bonding properties of both composite resin and resin cements to PAEK. Several studies have shown that surface treatment using sulfuric acid alone914151617 or sulfuric acid in combination with airabrasion1819 improved its bond strength. However, the chairside application of sulfuric acid for PEEK surface treatment can be hazardous and should be avoided if possible. In addition, the influence of various adhesive systems on the bond strength to PEEK was also investigated. Studies reported that treatment using silica coating prior to the application of multifunctional acrylates containing primer will yield a durable bond.520 On the other hand, most of the studies showed that MMA (methyl methacrylate)-containing adhesive systems after air-abrasion with 50-µm Al2O3 were able to enhance the bond strength to PEEK.82122 Despite many attempts, the adequate bond strength of veneering composite and resin cements to PAEK materials remains to be achieved.
Non-thermal plasma (NTP) surface treatment may be able to achieve mechanical and chemical changes in surface morphology to attain sufficient bond strength.9 Chemical changes include surface alteration of non-polar polymers by forming oxygen-containing functional groups such as C=O and –OH.23 According to a previous study, large numbers of hydroxyl and carboxylic acid groups were observed on the PEEK surface after plasma treatment (oxygen, air, and argon).13 The formed carboxyl group is expected to react with the epoxide group of the methacrylate-based dental adhesive systems to form covalent bonds between the adhesive and polymer. For shear bond strength, a study reported that argon plasma treatment significantly improved the bond strength between the PEEK surface and RelyX Unicem in comparison to the group with no treatment, hydrofluoric acid treatment, or sandblasting.9 In contrast, a previous in vitro study showed that helium plasma treatment did not produce bonding improvement between PEEK and self-adhesive resin cements.24 In this present study, pre-treatment with NTP significantly improved the bond strength of PEKK to resin cements. In addition, when NTP was immediately applied after sandblasting prior to surface conditioning with Visio. link, it exhibited significantly higher bond strength among the other groups. This may be a result of the alteration of the PEKK surface from sandblasting and the chemical changes after NTP treatment. Therefore, the adhesive was able to penetrate into the surface pores to create an efficient bond. In addition, exposure to plasma discharge is a sufficient way to break chemical bonds (C–C, C–H) and leaves free radicals at or near the surface.25 These free radicals provide additional binding sites with other surface radicals or by chain transfer reaction for both Visio. link and resin cements after sandblasting.2526 A previous in vitro study demonstrated a similar result after the PEKK surface was treated with a combination of oxygen gas plasma and sandblasting.27
Thermocycling or artificial aging simulates the long-term durability of bond strength within the interface of each material in the oral environment. All specimens were subjected to 10,000 cycles in a thermocycling machine, which approximately corresponds to one service year.22 In previous investigations, artificial aging induced higher bond strength values due to post-polymerization of the adhesive system and resin materials.828 However, other previous studies observed a significant decrease in SBS after plasma treatment.2427 In this study, artificial aging produced significantly decreased bond strength in all groups treated with plasma. These results might be caused by low resistance to hydrolysis of the hydrogen bond after plasma application during thermocycling. Although the long-term effects of NTP remain unclear, the combination effect of NTP and SB in improving SBS has potential use in clinical application of prostheses using PEKK material. However, long-term clinical trials are needed to further evaluate the effect of non-thermal plasma on shear bond strength.
The contact angles on untreated PEKK specimens have higher values compared to the specimens after surface treatment. This is due to the low-energy and hydrophobic nature of the polymer surface.29 In this study, the wettability of the material increased after surface treatment when the sandblast treatment was combined with non-thermal plasma. Introduction of the –COOH group further lowered the contact angle after sandblasting, from a mean value of 69.5° to 40.8°. Surface activation after plasma treatment produced more functional groups, thus increasing hydrophilicity.
Failure mode analysis indicated that there was no difference in fracture mode depending on aging. Adhesive failure was predominant in the UT and NTP groups, while mixed failure was more common in the SB and SB + NTP groups. Groups that predominantly exhibited adhesive types of failure indicated that the bond strength between the composite material and resin cement was not sufficient.30 On the other hand, mixed type was due to the uneven stress distribution at the interface during loading. The less common cohesive type was a result of the uneven stress distribution at the bonding interface.31
The results obtained in the present study demonstrated increased bond strength between resin cement and PEKK after surface treatment using non-thermal plasma (NTP). Therefore, the null hypothesis that NTP has no effect on shear bond strength was rejected.
Within the limitations of this present study, surface treatment with air-type non-thermal plasma in combination with sandblasting improved shear bond strength between PEKK material and resin cements. However, artificial aging significantly reduced the shear bond strength. Further research is needed to verify and understand the effects of these treatments.
References
1. Domininghaus H. Resin material and its properties, 6. Berlin, Heidelberg: Springer-Verlag;2005. p. 1203–1222.
2. Mark H. Encyclopedia of polymer science and technology, 3. Hoboken, NJ: John Wiley & Sons;2007. p. 377.
3. Kurtz SM, Devine JN. PEEK biomaterials in trauma, orthopedic, and spinal implants. Biomaterials. 2007; 28:4845–4869. PMID: 17686513.


4. Toth JM, Wang M, Ester BT, Scifert JL, Seim HB, Turner HS. Polyetheretherketone as a biomaterial for spinal applications. Biomaterials. 2006; 27:324–334. PMID: 16115677.


5. Fuhrmann G, Steiner M, Freitag-Wolf S, Kern M. Resin bonding to three types of polyaryletherketones (PAEKs)-durability and influence of surface conditioning. Dent Mater. 2014; 30:357–363. PMID: 24461250.


6. Han KH, Lee JY, Shin SW. Implant- and Tooth-Supported Fixed Prostheses Using a High-Performance Polymer (Pekkton) Framework. Int J Prosthodont. 2016; 29:451–454. PMID: 27611747.


7. Lee KS, Shin JH, Kim JE, Kim JH, Lee WC, Shin SW, Lee JY. Biomechanical Evaluation of a Tooth Restored with High Performance Polymer PEKK Post-Core System: A 3D Finite Element Analysis. Biomed Res Int. 2017; 2017:1373127. PMID: 28386547.


8. Stawarczyk B, Keul C, Beuer F, Roos M, Schmidlin PR. Tensile bond strength of veneering resins to PEEK: impact of different adhesives. Dent Mater J. 2013; 32:441–448. PMID: 23719006.


9. Zhou L, Qian Y, Zhu Y, Liu H, Gan K, Guo J. The effect of different surface treatments on the bond strength of PEEK composite materials. Dent Mater. 2014; 30:e209–e215. PMID: 24768752.


10. Noiset O, Schneider YJ, Marchand-Brynaert J. Adhesion and growth of CaCo2 cells on surface-modified PEEK substrata. J Biomater Sci Polym Ed. 2000; 11:767–786. PMID: 11011772.


11. Chen M, Zhang Y, Sky Driver M, Caruso AN, Yu Q, Wang Y. Surface modification of several dental substrates by non-thermal, atmospheric plasma brush. Dent Mater. 2013; 29:871–880. PMID: 23755823.


12. Rodriguez-Villanueva C, Encinas N, Abenojar J, Martinez MA. Assessment of atmospheric plasma treatment cleaning effect on steel surfaces. Surf Coat Technol. 2013; 236:450–456.
13. Comyn J, Mascia L, Xiao G, Parker BM. Plasma-treatment of polyetheretherketone (PEEK) for adhesive bonding. Int J Adhes Adhes. 1996; 16:97–104.


14. Schmidlin PR, Stawarczyk B, Wieland M, Attin T, Hämmerle CH, Fischer J. Effect of different surface pre-treatments and luting materials on shear bond strength to PEEK. Dent Mater. 2010; 26:553–559. PMID: 20206986.


15. Stawarczyk B, Jordan P, Schmidlin PR, Roos M, Eichberger M, Gernet W, Keul C. PEEK surface treatment effects on tensile bond strength to veneering resins. J Prosthet Dent. 2014; 112:1278–1288. PMID: 24969411.


16. Stawarczyk B, Beuer F, Wimmer T, Jahn D, Sener B, Roos M, Schmidlin PR. Polyetheretherketone-a suitable material for fixed dental prostheses? J Biomed Mater Res B Appl Biomater. 2013; 101:1209–1216. PMID: 23564476.


17. Sproesser O, Schmidlin PR, Uhrenbacher J, Roos M, Gernet W, Stawarczyk B. Effect of sulfuric acid etching of polyetheretherketone on the shear bond strength to resin cements. J Adhes Dent. 2014; 16:465–472. PMID: 25264546.
18. Hallmann L, Mehl A, Sereno N, Hammerle C. The improvement of adhesive properties of PEEK through pre-treatments. Appl Surf Sci. 2012; 258:7213–7218.
19. Pourkhalili H, Dastjerdi MR, Soltankarimi V, Razavi AS, Ramezani A, Talari FS, Alhavaz A. Effect of different surface treatment on the shear bond strength of veneering composite to polyetherketone core material. Int J Adv Biotech Res. 2016; 7:1116–1121.
20. Kern M, Lehmann F. Influence of surface conditioning on bonding to polyetheretherketon (PEEK). Dent Mater. 2012; 28:1280–1283. PMID: 23036863.


21. Keul C, Liebermann A, Schmidlin PR, Roos M, Sener B, Stawarczyk B. Influence of PEEK surface modification on surface properties and bond strength to veneering resin composites. J Adhes Dent. 2014; 16:383–392. PMID: 25133270.
22. Stawarczyk B, Taufall S, Roos M, Schmidlin PR, Lümkemann N. Bonding of composite resins to PEEK: the influence of adhesive systems and air-abrasion parameters. Clin Oral Investig. 2018; 22:763–771.


23. Yavirach P, Chaijareenont P, Boonyawan D, Pattamapun K, Tunma S, Takahashi H, Arksornnukit M. Effects of plasma treatment on the shear bond strength between fiber-reinforced composite posts and resin composite for core build-up. Dent Mater J. 2009; 28:686–692. PMID: 20019419.


24. Stawarczyk B, Bähr N, Beuer F, Wimmer T, Eichberger M, Gernet W, Jahn D, Schmidlin PR. Influence of plasma pretreatment on shear bond strength of self-adhesive resin cements to polyetheretherketone. Clin Oral Investig. 2014; 18:163–170.


25. Pelagade S, Singh NL, Shah S, Qureshi A, Rane RS, Mukherjee S, Deshpande UP, Ganesan V, Shripathi T. Surface free energy analysis for bipolar pulsed argon plasma treated polymer films. J Phys. 2010; 208:012107.


26. Kim S, Lee KJ, Seo Y. Polyetheretherketone (PEEK) surface functionalization by low-energy ion-beam irradiation under a reactive O2 environment and its effect on the PEEK/copper adhesives. Langmuir. 2004; 20:157–163. PMID: 15745014.


27. Stawarczyk B, Silla M, Roos M, Eichberger M, Lümkemann N. Bonding behaviour of polyetherketoneketone to methylmethacrylate-and dimethacrylate-based polymers. J Adhes Dent. 2017; 19:331–338. PMID: 28849801.
28. Piwowarczyk A, Lauer HC, Sorensen JA. In vitro shear bond strength of cementing agents to fixed prosthodontic restorative materials. J Prosthet Dent. 2004; 92:265–273. PMID: 15343162.


29. Nair K, Whiteside B, Grant C, Patel R, Tuinea-Bobe C, Norris K, Paradkar A. Investigation of plasma treatment on micro-injection moulded microneedle for drug delivery. Pharmaceutics. 2015; 7:471–485. PMID: 26529005.


30. Van Meerbeek B, Peumans M, Poitevin A, Mine A, Van Ende A, Neves A, De Munck J. Relationship between bond-strength tests and clinical outcomes. Dent Mater. 2010; 26:e100–e121. PMID: 20006379.


31. Meng X, Yoshida K, Atsuta M. Hardness development of dual-cured resin cements through different thicknesses of ceramics. Dent Mater J. 2006; 25:132–137. PMID: 16706308.


Fig. 3
Mean shear bond strengths of the specimens with different surface treatments without thermocycling. UT: Untreated, NTP: Non-thermal plasma, SB: Sandblasting. Different letters show a significant difference (P < .05).
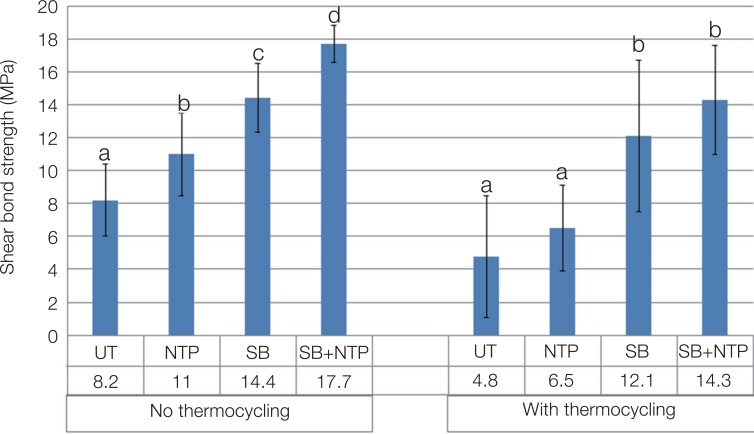
Fig. 4
Images of the contact angle (CA) after different surface treatments. UT: Untreated, NTP: Non-thermal plasma, SB: Sandblasting.

Fig. 5
Types of failure modes in various surface treatments. UT: Untreated, NTP: Non-thermal plasma, SB: Sandblasting.
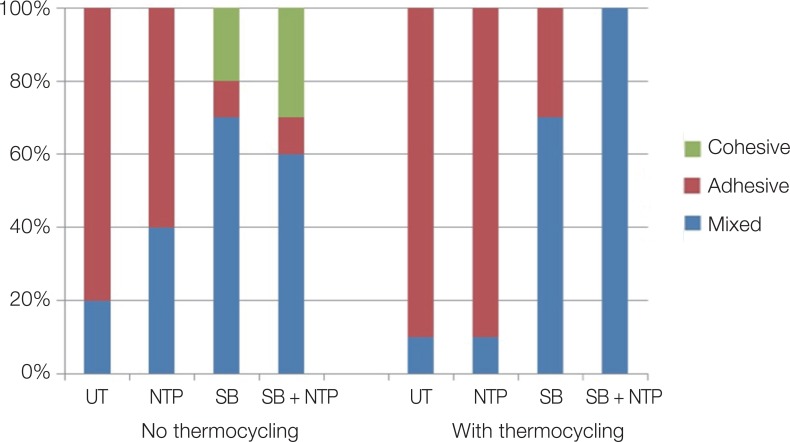
Table 1
Different surface treatments and numbers of specimens
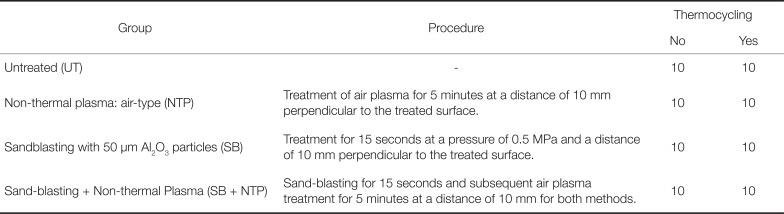
Table 2
Compositions of the resin cement and adhesive material
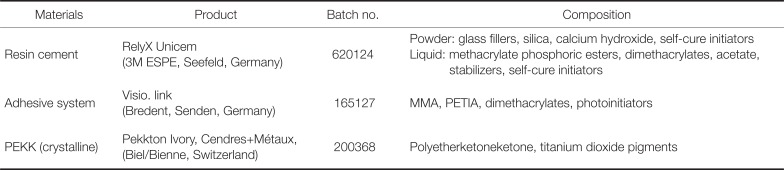