Abstract
Bacterial biofilms have been demonstrated to be closely related to clinical infections and contribute to drug resistance. Berberine, which is the main component of Coptis chinensis, has been reported to have efficient antibacterial activity. This study aimed to investigate the potential effect of a combination of berberine with ciprofloxacin (CIP) to inhibit Salmonella biofilm formation and its effect on expressions of related genes (rpoE, luxS, and ompR). The fractional inhibitory concentration (FIC) index of the combination of berberine with CIP is 0.75 showing a synergistic antibacterial effect. The biofilm's adhesion rate and growth curve showed that the multi-resistant Salmonella strain had the potential to form a biofilm relative to that of strain CVCC528, and the antibiofilm effects were in a dose-dependent manner. Biofilm microstructures were rarely observed at 1/2 × MIC/FIC concentrations (MIC, minimal inhibition concentration), and the combination had a stronger antibiofilm effect than each of the antimicrobial agents used alone at 1/4 × FIC concentration. LuxS, rpoE, and ompR mRNA expressions were significantly repressed (p < 0.01) at 1/2 × MIC/FIC concentrations, and the berberine and CIP combination repressed mRNA expressions more strongly at the 1/4 × FIC concentration. The results indicate that the combination of berberine and CIP has a synergistic effect and is effective in inhibiting Salmonella biofilm formation via repression of luxS, rpoE, and ompR mRNA expressions.
Salmonellosis is the major cause of food poisoning in humans throughout the world and has important significance in public health [8]. Salmonella causes severe diseases including gastroenteritis, septicemia, and partially purulent infection, which produce serious challenges to graziery and public health. Fluoroquinolones, including ciprofloxacin (CIP), are front-line drugs in the treatment of Salmonellosis [25]. However, overuse or inappropriate use of antimicrobial agents in animals, especially food-producing animals, can lead to the transfer of bacterial resistance to humans through the food chain [17]. An increase in the occurrence of multidrug-resistant Salmonella expressing resistance to fluoroquinolones has become a global concern recently [2]. The treatment of Salmonellosis becomes increasingly difficult due to drug resistance, which has been demonstrated to be related to bacterial biofilm formation [21]. A biofilm is generally defined as a structured community of bacterial cells, enclosed in a self-produced polymeric matrix and adherent to an inert or living surface [11]. In fact, the adherent sessile cells within a biofilm are highly resistant to antimicrobials and host defenses [1] and are closely related to host colonization and virulence. Additionally, it was estimated that 80% of all clinical infections involve biofilm [24]. Therefore, considering biofilm as a target in drug development aimed at inhibiting bacterial biofilm production has been a hot topic in the study of antibacterial infections.
Previous reports have mentioned that Salmonella biofilm formation was associated with the expressions of cellulose, biofilm-associated protein A (BapA), and curli [32]. Effective control of biofilms can be achieved by understanding the molecular mechanisms, especially the expression of regulatory genes involved in biofilm formation. The regulation of transcription, which is the most basic and important way to precisely control the life of prokaryotes, depends on various sigma (σ) factors, such as the rpoE regulator. Studies have shown that rpoE deletion can account for the inhibition of biofilm formation as a result of repressing curli. S-Ribosylhomocysteinase (LuxS) has an important role in the activated methyl cycle pathway and quorum sensing, which leads to biofilm formation and causes other virulence factors in many bacterial species [22]. It has been reported that the luxS gene is involved in the regulation of cell growth, biofilm formation, quorum sensing, motility, virulence, and resistance [15]. OmpR is a regulatory gene for biofilm formation and is an important virulence factor in Salmonella. The well-characterized targets of ompR regulation include capsule formation, flagella, and virulence factor regulation [5]. Thus, identification of products that could inhibit luxS, rpoE, and ompR gene expressions may be an important new research focus that may indicate a natural, widespread, antimicrobial strategy with significant potential impact on biofilm formation.
At present, much attention has been focused on traditional Chinese herbal medications in combination with antimicrobials in order to achieve drug synergy, enhance efficacy, and reduce toxicity [23]. Coptis chinensis has been widely used with the effects of purging intense heat and removing toxicosis, as well as anti-inflammatory, anti-diabetic, antibacterial, antioxidant, and anti-Alzheimer effects [31] due to its multi-alkaloid constituents [6]. Studies have shown that the main component of C. chinensis is berberine [9], the principal alkaloid in C. chinensis and the most important contributor to therapeutic effects. Nevertheless, there is evidence that berberine has a low level of toxicity [28]. Regardless, some studies have focused on the antibiofilm effect and inhibitory mechanism of berberine combined with CIP on Salmonella at the molecular level. Such a combination could be part of a new antibiofilm strategy and represent an important breakthrough in integrative medicine research by providing an insight into the molecular mechanism of drug resistance.
This study was aimed to explore the antibiofilm effect of berberine combined with CIP on Salmonella and to investigate that effect in order to provide a new theoretical basis and determine its clinical significance in the inhibition of biofilm formation and elucidating the resistance phenotype of Salmonella.
Salmonella strains were isolated from chickens and preserved under standard laboratory protocol after identification. Salmonella gallinarum CVCC528 (control strain) was purchased from the National Institute for the Control of Pharmaceutical and Biological Products (China).
By using the Kirby-Bauer method, recommended by the World Health Organization and the guidelines of the Clinical and Laboratory Standards Institute (CLSI) [7], we tested the antimicrobial susceptibility of Salmonella strains. We analyzed the susceptibility of common therapeutic antimicrobial agents including ofloxacin (5 µg), ampicillin (10 µg), chloramphenicol (30 µg), CIP (5 µg), gentamicin (10 µg), norfloxacin (10 µg), sulfamethoxazole-trimethoprim (25 µg), cefazolin (30 µg), tetracycline (30 µg), and kanamycin (30 µg) (BioKangtai, China). Broth at the concentration of 1 × 108 CFU·mL−1 (CFU, colony-forming unit) was evenly coated on Mueller-Hinton agar plates and antimicrobial susceptibility disks adhered completely on the surface of plate center. The plates were then held at 37℃ for 16 to 18 h. Subsequently, inhibition zone was measured with a Vernier caliper to determine susceptibility according to CLSI [7].
Air-dried rhizome of C. chinensis (65 g) was purchased from Harbin Sankeshu Medicinal Herbs Market (China). Berberine extraction was performed as previously described [19]. In brief, C. chinensis rhizome was pulverized and alcohol-extracted with 520 mL 75% EtOH (1:8, VC. chinensis/VEtOH) at 50℃. The extract was concentrated and heated at 100℃ to dissolve in acetic acid solution. Extract pH (1–2) was adjusted with hydrochloric acid. Purified berberine was obtained by filtrating, washing, and evaporating under vacuum twice with cold water and once with acetone. Finally, 5.31 g berberine extracts were collected at an extraction rate of 8.17 ± 0.2%. The berberine was analyzed via ultraviolet spectrophotometry. The dried berberine was diluted in distilled water at a concentration of 100,000 µg/mL and stored at 4℃.
The minimal inhibition concentration (MIC) was determined by using the broth microdilution method according to the CLSI. The fractional inhibitory concentration (FIC) of berberine and CIP was estimated by applying a checkerboard method mixed at different concentrations. The formula for calculation of the FIC index was
where A is berberine and B is CIP. A FIC index < 1.0 indicates a synergistic interaction; 1.0 indicates an additive interaction; 1.0 < FIC index < 2.0 indicates a sub-additive interaction; 2.0 indicates an indifferent interaction; FIC index > 2.0 indicates an antagonistic interaction [1218]. All tests were performed in triplicate.
Biofilm formation and the antibiofilm effect of three antimicrobial agents (berberine, CIP, and the combination of the two) on Salmonella were tested in 96-well plates. Each well was filled with 100 µL antimicrobial agent and inoculated with 100 µL cultures (105 CFU/mL). The final concentrations of the three antimicrobial agents were 1 × MIC/FIC, 1/2 × MIC/FIC, and 1/4 × MIC/FIC, respectively. Negative control wells were only filled with TSB culture medium, and wells inoculated with 200 µL diluted bacterial suspension without antimicrobial agents were taken as positive controls. Plates were wrapped with parafilm to prevent evaporation during incubation for 72 h at 37℃. Biofilms were quantified by CV assay [3] to measure the optical density at 595 nm (OD595 nm) using a microplate reader. The formula for calculation of the B value (adhesion rate) is
where A1 refers to the OD595 nm at the end of incubation, A2 refers to the OD595 nm after staining, and A1c and A2c refer to the blank control wells. Each experiment was performed in triplicate and the mean ± SD was calculated.
Biofilms were prepared in 96-well plates as described above, and cultured for 2, 6, 12, 18, 24, 30, 36, 48, 72, 96, and 120 h. Afterward, each well was filled with 20 µL XTT and incubated in the dark for 2 h at 37℃ to measure the OD450 nm using a microplate reader. Each experiment was performed in triplicate and the mean ± SD was calculated.
Biofilm microstructure was analyzed using scanning electron microscopy (SEM) [4]. Salmonella treated with different concentrations of the antimicrobial agents were cultured as described above and biofilms were allowed to develop on sterilized coverslips which were placed vertically in 24-well plates for 72 h at 37℃. Samples were fixed, dehydrated and critical point dried, gold sputtered with an ion sputtering instrument (current 15 mA, 2 min) and observed using SEM.
Total RNA was extracted from bacterial cells using TRIzol reagent (Invitrogen, USA) according to the manufacturer's protocol. The RNA was quantified using a Nanodrop 2000C spectrophotometer (Thermo Scientific, USA). The RNA concentration was fixed to 1 µg/µL and then cDNA was synthesized using a PrimeScript RT-PCR Kit (Takara Bio, Japan). The qRT-PCR was performed using the Green SYBR I method. The qRT-PCR reactions were carried out as follows: 94℃ for 30 sec and 45 cycles of 94℃ for 5 sec, 60℃ for 15 sec, and 72℃ for 15 sec. The expression level of each gene was normalized to the level of 16S rRNA. The relative quantitative results were analyzed using the 2−ΔΔCt method [16]. The primers for rpoE, luxS, and ompR are listed in Table 1.
All the data were analyzed in SPSS statistical software (ver. 17.0; SPSS, USA) using one-way analysis of variance (ANOVA) with Tukey's honest significant difference post hoc test. A p value of < 0.05 or < 0.01 was considered statistically significant. The data were expressed as mean ± SD values.
Inhibition zone of the Salmonella strains are presented in Table 2. Salmonella strain (No. 5) was observed to be resistant to all of the antibiotics except ofloxacin, showing that it was a particularly multi-resistant Salmonella strain with a complex resistance phenotype. Therefore, we used the No. 5 multi-resistant Salmonella strain for subsequent experiments.
The MICs and the FIC indices of the multi-resistant Salmonella strain and the CVCC528 strain are presented in Table 3. According to the determining standards for FIC indices, berberine combined with CIP had a synergistic effect against both the multi-resistant Salmonella strain and CVCC528, as the FIC index was 0.75 (MICs of berberine reduced from 3,125–1,562 µg/mL; MICs of CIP reduced from 2.56–0.64 µg/mL). The results indicate that berberine combined with CIP could be an effective approach to treat Salmonella infections.
The biofilm adhesion rate results of the different antimicrobial agents are presented in Fig. 1. The biofilm adhesion rate of the multi-resistant Salmonella strain was 0.246, which was much higher than that of CVCC528 (B595 nm = 0.0159). As shown in panels A and B in Fig. 1, the biofilm adhesion rate of Salmonella administrated a 1/8 × MIC concentration of the CIP and berberine combination was markedly decreased from that of the control group (p < 0.05), and those of the 1 × MIC, 1/2 × MIC, and 1/4 × MIC groups were significantly decreased compared with the control group (p < 0.01). In addition, the adhesion rate gradually decreased with an increased antimicrobial agent concentration. Similarly, when Salmonella was administrated the CIP and berberine combination, the adhesion rates of the 1 × FIC, 1/2 × FIC, and 1/4 × FIC groups were significantly decreased, in a dose-dependent manner, from that in the control group (p < 0.01) (panel C in Fig. 1). In the 1/2 × FIC group, the adhesion rate (B595 nm = 0.082) was lower than those in the 1/2 × MIC CIP group (B595 nm = 0.091) and the 1/2 × MIC berberine group (B595 nm = 0.112) (panel D in Fig. 1). Also, in the 1/2 × FIC group, the adhesion rate (B595 nm = 0.120) was lower than those in the 1/2 × MIC CIP group (B595 nm = 0.125) and the 1/2 × MIC berberine group (B595 nm = 0.133).
The biofilm growth curve results are presented in Fig. 2. The multi-resistant Salmonella strain apparently produced biofilm in a duration between 18 h and 30 h (panel A in Fig. 2). However, there was no obvious growth trend in the biofilm formation of the CVCC528 strain. Compared with non-treated multi-resistant Salmonella strain (panel A in Fig. 2), CIP, berberine, and the combination treatments significantly inhibited biofilm formation at 1/2 × MIC/FIC and 1/4 × MIC/FIC concentration (panels B–D in Fig. 2). It is evident that sub-MICs of berberine (OD450 nm = 0.765 at 24 h with 1/2 × MIC, OD450 nm = 0.861 at 24 h with 1/4 × MIC), CIP (OD450 nm = 0.760 at 24 h with 1/2 × MIC, OD450 nm = 0.869 at 24 h with 1/4 × MIC), and the combination (OD450 nm = 0.755 at 24 h with 1/2 × FIC, OD450 nm = 0.854 at 24 h with 1/4 × FIC) could inhibit bacterial biofilm growth during the logarithmic growth phase effectively compared to that of the non-treated strain (OD450 nm = 1.982 at 24 h). As shown in panel A in Fig. 2, the biofilm formation of multi-resistant Salmonella strain entered the stationary phase at 72 h. It is evident that the sub-MICs of the antimicrobial agents inhibit biofilm formation during the stationary phase (OD450 nm decreased from 2.457 to 0.898 at 96 h with 1/2 × FIC of combination).
SEM images of the Salmonella strains were taken to visualize the biofilm microstructure that could reflect the effect of the antimicrobial agents (berberine, CIP, and the combination) on biofilm formation (Fig. 3). A representative micrograph of the multi-resistant Salmonella strain biofilms (non-treated group) is presented in panel A in Fig. 3, and a micrograph of the CVCC528 biofilm is presented in panel B in Fig. 3. There was no evidence of a biofilm attached on the glass slide with the CVCC528 strain. In particular, SEM revealed the appearance of a thick polyptychial extracellular polymeric substance and mutual adhesion of the Salmonella with a high cell density. However, after treatment with 1/2 × MIC/FIC concentration of antimicrobial agents, the biofilm microstructure was severely damaged with changes in cell morphology (panels C, E, and F in Fig. 3). Treated biofilm cells were distributed in monolayers with a remarkable decrease in thickness and sizes compared to non-treated cells. When the culture medium was added to the 1/4 × MIC/FIC concentration of antimicrobial agents, the biofilms were observed to have less thickness and a smaller size than the non-treated cells, but the films were much more massive than that in the 1/2 × MIC/FIC treated cells. The SEM analysis revealed that berberine, CIP, and the combination can inhibit Salmonella biofilm formation at a sub-MIC concentration.
The changes in luxS, rpoE, and ompR mRNA expression levels are displayed in Fig. 4. Compared to the non-treated group, the presence of the antimicrobial agents caused reductions in the mRNA expression levels of the three genes in a dose-dependent manner. Moreover, the 1/2 × MIC/FIC concentrations of the antimicrobial agents caused significant decreases (p < 0.01) in the luxS, rpoE, and ompR genes mRNA expression levels. The berberine and CIP combination had a stronger effect than those of CIP or berberine alone at the 1/4 × MIC/FIC concentration against the multi-resistant Salmonella strain. The 1/4 × MIC CIP treatment decreased ompR mRNA expression markedly (p < 0.05) and rpoE mRNA expression significantly (p < 0.01), but it did not change the luxS mRNA expression (panel A in Fig. 4). However, the 1/4 × FIC of the combination treatment significantly decreased the mRNA expressions of all three genes (p < 0.01). Intriguingly, it has been observed that CIP was more efficient than berberine or the combination against the CVCC528 strain (panel B in Fig. 4). These findings suggest that CIP, berberine, and the CIP and berberine combination can inhibit luxS, rpoE, and ompR mRNA expressions, and the combination was more efficient than CIP or berberine alone at the 1/4 × MIC/FIC concentration.
Berberine is the main alkaloid of C. chinensis and is the most important contributor to its therapeutic effects; therefore, we extracted and used berberine in the current study. Our results revealed a potential antibacterial effect of berberine on Salmonella strains. In this study, based on the Loewe theory, an FIC index of 0.75 revealed a synergistic effect of berberine and CIP against the tested Salmonella strains. Our data demonstrate that berberine enhances the antimicrobial activity of CIP against Salmonella, and their combination might reduce the CIP dose required for therapy, providing the additional benefit of avoiding drug resistance and side-effects. However, interactions between berberine and CIP and their effects on Salmonella remain unknown.
Previous studies have demonstrated an antibiofilm effect of C. chinensis on Escherichia coli and Streptococcus suis [14]. Adhesion of bacteria on a surface is the first step of biofilm formation; thus, adhesion and growth of the biofilm were studied to evaluate biofilm formation ability. According to the standard for antimicrobial susceptibility testing of Salmonella provided by the CLSI [7], strain CVCC528 was sensitive to all of the antibiotics in this test. It is clear from our results that the CVCC528 strain was a weak biofilm producer, whereas the multi-resistant Salmonella strain (No. 5) was observed to be a strong biofilm producer. Biofilm formation is related to the cell itself and includes flagella, pili, and prosthecae, and it has a certain relationship with resistant phenotypes [30]. A significant antibiofilm effect against the multi-resistant Salmonella strain was revealed, including effective adhesion and growth. We found that berberine, CIP, and their combination could inhibit biofilm formation and even damage biofilms by affecting film thickness and the size of monolayers in a dose-dependent manner. Furthermore, the antibiofilm effects varied among the antimicrobial agents, with the combination having a stronger inhibitory effect than berberine and CIP alone. Moreover, the effects were positively correlated with the antimicrobial concentration, especially at 1/4 × MIC/FIC concentration. It was noted that the inhibitory effect against biofilms was particularly evident when berberine was combined with CIP. Based on these findings, the combination of berberine and CIP could be used as new treatments for biofilm inhibition and consequently may provide new strategies to avoid biofilm formation by reducing biofilm growth rates, cell density, and the extracellular matrix.
Some genes related to stress responses and the quorum-sensing signaling system can promote the drug resistance of a biofilm [26]. This study investigated the regulation of mRNA expressions of some biofilm-related genes. RpoE is known to play an essential role in activating or regulating gene expression in response to heat shock, hypertonic, starvation, and oxygen stress. OmpR responds to osmotic stress and cytoplasmic acidification, which requires the secretion of virulence factors [5], and ompR deletion can lead to repression of biofilm formation, flagella, and virulence factors. Flagella and pili have been shown to be important for adhesion and colonization to surfaces, and a Salmonella biofilm is associated with the expression of cellulose and curli [32]. OmpR deletion has an antibiofilm effect by repressing pili and cellulose [10], but rpoE deletion causes repression of curli and flagellin [13]. In this study luxS, rpoE, and ompR mRNA expressions were suppressed by berberine, CIP, and their combination in varying degrees, which indicated that the antibiofilm effect of berberine, CIP, and the combination may occur via inhibition of curli, adhesion, and cell growth by repression of luxS, rpoE, and ompR gene expressions. In addition, the inhibitory effect of the antimicrobials increased in a dose-dependent manner and was most significant at the 1/2 × MIC/FIC concentration. Many reports have demonstrated that biofilm formation could be inhibited in the presence of sub-inhibitory concentrations of antimicrobial agents in a dose-dependent manner [202729]. The biofilm formation and gene expression results in this study showed that the inhibitory effect of the berberine and CIP combination at a 1/2 FIC concentration was more effective than that at a 1/4 FIC concentration, showing that the inhibitory effect on biofilm occurred in a dose-dependent manner. Moreover, comparative inhibitory effects of the three antimicrobial remedies were examined. The inhibitory effect of the berberine and CIP combination was stronger than those of berberine or CIP alone for the multi-resistant Salmonella strain at the 1/4 × MIC/FIC concentration, but the combination did not show any obvious therapeutic advantage at the 1/4 × MIC/FIC concentration. Thus, we speculate that this phenomenon may be related to the multiple drug resistant phenotypes of the multi-resistant Salmonella strain and to the therapeutic targets of CIP. Based upon these results, we suggest that the antibiofilm effect of berberine, CIP, and the combination were associated with downregulation of luxS, rpoE, and ompR, which are related to the formation of pili, cellulose, curli, flagellin, and virulence factors.
In conclusion, our study has revealed antibiofilm effects of berberine and CIP alone and of their combination. Our findings show that the luxS, rpoE, and ompR genes are essential for Salmonella biofilm formation. The combination of berberine and CIP leads to enhanced efficacy and reduced toxicity of the antimicrobials. Moreover, the combination reduces the CIP dose required for therapy, providing the benefits of avoiding drug resistance and reducing side-effects. Further study is needed to confirm and clarify the relationships between CIP and berberine and their antibiofilm effect at the molecular level, and a clear understanding of gene regulation in Salmonella is needed to reveal the mechanism of biofilm formation, allowing the development of effective methods to inhibit biofilm formation.
Figures and Tables
Fig. 1
Effects of ciprofloxacin (CIP) (A), berberine (B), and their combination (C) on the biofilm formation by No. 5 multi-resistant Salmonella strain and the CVCC528 strain. (D) Comparison of antibiofilm effects of CIP, berberine, and the combination on the multi-resistant Salmonella strain. Data are presented as mean ± SD. Marked difference (*p < 0.05), significant difference (**p < 0.01) compared to the non-treated group. MIC, minimal inhibition concentration; FIC, fractional inhibitory concentration.
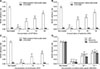
Fig. 2
The biofilm growth curves of the multi-resistant Salmonella strain and the CVCC528 strain without antimicrobial agents (A) and with ciprofloxacin (CIP) (B), berberine (C), and the combination (D). OD, optical density; MIC, minimal inhibition concentration; FIC, fractional inhibitory concentration.
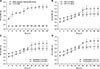
Fig. 3
Biofilm microstructure observations of Salmonella strains under scanning electron microscopy. (A) Non-treated multi-resistant Salmonella strain. (B) Non-treated CVCC528 strain. (C) Multi-resistant Salmonella strain with a 1/2 × MIC concentration of CIP. (D) Multi-resistant Salmonella strain with a 1/4 × MIC concentration of CIP. (E) Multi-resistant Salmonella strain with a 1/2 × MIC concentration of berberine. (F) Multi-resistant Salmonella strain with a 1/4 × MIC concentration of berberine. (G) Multi-resistant Salmonella strain with a 1/2 × FIC concentration of the berberine and CIP combination. (H) Multi-resistant Salmonella strain with a 1/4 × FIC concentration of the combination. MIC, minimal inhibition concentration; CIP, ciprofloxacin; FIC, fractional inhibitory concentration. Scale bars = 100 µm (A–H).
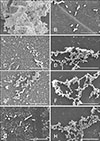
Fig. 4
The effects of the antimicrobial agents on luxS, rpoE, and ompR mRNA expression levels in the multi-resistant Salmonella strain (A) and in strain CVCC528 (B). Marked difference (*p < 0.05), significant difference (**p < 0.01) compared to the non-treated group. CIP, ciprofloxacin; FIC, fractional inhibitory concentration.
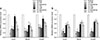
Acknowledgments
This work was supported by the Application Technology Research and Development Projects in Heilongjiang Province of China (grant No. PC13S03) and the foundation of Heilongjiang Province Educational Committee of China (grant No. 11541030).
References
1. Abdallah M, Benoliel C, Drider D, Dhulster P, Chihib NE. Biofilm formation and persistence on abiotic surfaces in the context of food and medical environments. Arch Microbiol. 2014; 196:453–472.


2. Bai L, Zhao J, Gan X, Wang J, Zhang X, Cui S, Xia S, Hu Y, Yan S, Wang J, Li F, Fanning S, Xu J. Emergence and diversity of Salmonella enterica serovar Indiana isolates with concurrent resistance to ciprofloxacin and cefotaxime from patients and food-producing animals in China. Antimicrob Agents Chemother. 2016; 60:3365–3371.


3. Castelijn GA, Parabirsing JA, Zwietering MH, Moezelaar R, Abee T. Surface behaviour of S. Typhimurium, S. Derby, S. Brandenburg and S. Infantis. Vet Microbiol. 2013; 161:305–314.


4. Castelijn GA, van der Veen S, Zwietering MH, Moezelaar R, Abee T. Diversity in biofilm formation and production of curli fimbriae and cellulose of Salmonella Typhimurium strains of different origin in high and low nutrient medium. Biofouling. 2012; 28:51–63.


5. Chakraborty S, Mizusaki H, Kenney LJ. A FRET-based DNA biosensor tracks OmpR-dependent acidification of Salmonella during macrophage infection. PLoS Biol. 2015; 13:e1002116.
6. Choi JS, Ali MY, Jung HA, Oh SH, Choi RJ, Kim EJ. Protein tyrosine phosphatase 1B inhibitory activity of alkaloids from Rhizoma Coptidis and their molecular docking studies. J Ethnopharmacol. 2015; 171:28–36.


7. Clinical and Laboratory Standards Institute (CLSI). Performance Standards for Antimicrobial Susceptibility Testing. 28th ed. Wayne: CLSI;2018. CLSI Supplement M100.
8. Cogan TA, Humphrey TJ. The rise and fall of Salmonella Enteritidis in the UK. J Appl Microbiol. 2003; 94:114S–119S.
9. Dai C, Wang J, Kong W, Xiao X, Peng C, Zhao Y, Jin C. Investigation on the antibacterial activity of Coptis chinensis Franch and its components compatibility by microcalorimetry. Acta Chim Sinica. 2010; 68:936–940.
10. Garmendia J, Beuzón CR, Ruiz-Albert J, Holden DW. The roles of SsrA-SsrB and OmpR-EnvZ in the regulation of genes encoding the Salmonella typhimurium SPI-2 type III secretion system. Microbiology. 2003; 149:2385–2396.


11. Jeong HH, Jeong SG, Park A, Jang SC, Hong SG, Lee CS. Effect of temperature on biofilm formation by Antarctic marine bacteria in a microfluidic device. Anal Biochem. 2014; 446:90–95.


12. Kim JH, Yu D, Eom SH, Kim SH, Oh J, Jung WK, Kim YM. Synergistic antibacterial effects of chitosan-caffeic acid conjugate against antibiotic-resistant acne-related bacteria. Mar Drugs. 2017; 15:pii: E167.


13. Li J, Overall CC, Nakayasu ES, Kidwai AS, Jones MB, Johnson RC, Nguyen NT, McDermott JE, Ansong C, Heffron F, Cambronne ED, Adkins JN. Analysis of the Salmonella regulatory network suggests involvement of SsrB and H-NS in σE-regulated SPI-2 gene expression. Front Microbiol. 2015; 6:27.


14. Li YH, Zhou YH, Ren YZ, Xu CG, Liu X, Liu B, Chen JQ, Ding WY, Zhao YL, Yang YB, Wang S, Liu D. Inhibition of Streptococcus suis adhesion and biofilm formation in vitro by water extracts of Rhizoma Coptidis. Front Pharmacol. 2018; 9:371.


15. Ling H, Kang A, Tan MH, Qi X, Chang MW. The absence of the luxS gene increases swimming motility and flagella synthesis in Escherichia coli K12. Biochem Biophys Res Commun. 2010; 401:521–526.


16. Livak KJ, Schmittgen TD. Analysis of relative gene expression data using real-time quantitative PCR and the 2−ΔΔCT method. Methods. 2001; 25:402–408.


17. Marshall BM, Levy SB. Food animals and antimicrobials: impacts on human health. Clin Microbiol Rev. 2011; 24:718–733.


18. Norden CW, Wentzel H, Keleti E. Comparison of techniques for measurement of in vitro antibiotic synergism. J Infect Dis. 1979; 140:629–633.


19. Ong ES, Woo SO, Yong YL. Pressurized liquid extraction of berberine and aristolochic acids in medicinal plants. J Chromatogr A. 2000; 904:57–64.


20. Rachid S, Ohlsen K, Witte W, Hacker J, Ziebuhr W. Effect of subinhibitory antibiotic concentrations on polysaccharide intercellular adhesin expression in biofilm-forming Staphylococcus epidermidis. Antimicrob Agents Chemother. 2000; 44:3357–3363.


21. Rao RS, Karthika RU, Singh SP, Shashikala P, Kanungo R, Jayachandran S, Prashanth K. Correlation between biofilm production and multiple drug resistance in imipenem resistant clinical isolates of Acinetobacter baumannii. Indian J Med Microbiol. 2008; 26:333–337.


22. Sela S, Frank S, Belausov E, Pinto R. A mutation in the luxS gene influences Listeria monocytogenes biofilm formation. Appl Environ Microbiol. 2006; 72:5653–5658.


23. Sharma M, Manoharlal R, Negi AS, Prasad R. Synergistic anticandidal activity of pure polyphenol curcumin I in combination with azoles and polyenes generates reactive oxygen species leading to apoptosis. FEMS Yeast Res. 2010; 10:570–578.


24. Steenackers HP, Janssens JC, Levin J, Voet A, De Maeyer M, De Vos DE, Vanderleyden J, De Keersmaecker SJ. Inhibition of salmonella biofilm formation: a sustainable alternative in the production of safe and healthy food. Commun Agric Appl Biol Sci. 2008; 73:71–76.
25. Sun Y, Dai M, Hao H, Wang Y, Huang L, Almofti YA, Liu Z, Yuan Z. The role of RamA on the development of ciprofloxacin resistance in Salmonella enterica serovar Typhimurium. PLoS One. 2011; 6:e23471.
26. Tobin DM, Vary JC Jr, Ray JP, Walsh GS, Dunstan SJ, Bang ND, Hagge DA, Khadge S, King MC, Hawn TR, Moens CB, Ramakrishnan L. The lta4h locus modulates susceptibility to mycobacterial infection in zebrafish and humans. Cell. 2010; 140:717–730.


27. Wang S, Yang Y, Zhao Y, Zhao H, Bai J, Chen J, Zhou Y, Wang C, Li Y. Sub-MIC tylosin inhibits Streptococcus suis biofilm formation and results in differential protein expression. Front Microbiol. 2016; 7:384.


28. Yang Y, Ye XL, Li XG, Zhen J, Zhang B, Yuan L. Synthesis and antimicrobial activity of 8-alkylberberine derivatives with a long aliphatic chain. Planta Med. 2007; 73:602–604.


29. Yang YB, Wang S, Wang C, Huang QY, Bai JW, Chen JQ, Chen XY, Li YH. Emodin affects biofilm formation and expression of virulence factors in Streptococcus suis ATCC700794. Arch Microbiol. 2015; 197:1173–1180.


30. Yu HH, Kim KJ, Cha JD, Kim HK, Lee YE, Choi NY, You YO. Antimicrobial activity of berberine alone and in combination with ampicillin or oxacillin against methicillin-resistant Staphylococcus aureus. J Med Food. 2005; 8:454–461.

