Abstract
Purpose
Research has shown that sevoflurane-induced toxicity causes neurodegeneration in the developing brain. miR-34a has been found to negatively regulate ketamine-induced hippocampal apoptosis and memory impairment. However, the role of miR-34a in sevoflurane-induced hippocampal neurodegeneration remains largely unclear.
Materials and Methods
C57/BL6 mice (7-day-old) inhaled 2.3% sevoflurane for 2 h/day over 3 consecutive days. miR-34a expression was reduced through intracerebroventricular injection with miR-34a interference lentivirus vector (LV-anti-miR-34a) into mouse hippocampus after anesthesia on the first day of exposure. Hippocampal apoptosis was detected by TUNEL assay and flow cytometry analysis. Spatial memory ability was evaluated by the Morris water maze test. The interaction between miR-34a and Wnt1 was confirmed by luciferase reporter assay, RNA immunoprecipitation, Western blot, and immunofluorescence staining. The effects of miR-34a on protein levels of B-cell lymphoma 2 (Bcl-2), bcl-2-like protein 4 (Bax), and Wnt/β-catenin pathway-related proteins were evaluated using Western blot analysis.
Results
Sevoflurane upregulated hippocampal miR-34a, and miR-34a inhibitor attenuated sevoflurane-induced hippocampal apoptosis and memory impairment. miR-34a negatively regulated Wnt1 expression by targeting miR-34a in hippocampal neurons. Moreover, forced expression of Wnt1 markedly undermined miR-34a-mediated enhancement of sevoflurane-induced apoptosis of hippocampal neurons, while Wnt1 silencing greatly restored anti-miR-34a-mediated repression of sevoflurane-induced apoptosis of hippocampal neurons. Increased expression of miR-34a inhibited the Wnt/β-catenin pathway in hippocampal neurons exposed to sevoflurane, while anti-miR-34a exerted the opposite effects.
Increasing studies have revealed neuronal toxicity induced by commonly used anesthetics both in animals and humans and have suggested that these anesthetics would unavoidably induce neurodegeneration and apoptosis in the hippocampus, thereby leading to learning and memory impairment.1 Sevoflurane is one of the most commonly used volatile anesthetics and is administered by inhalation for both induction and maintenance of anesthesia in clinical surgery for pediatric patients.2 Research has shown that sevoflurane-induced toxicity elicits apoptosis of rat hippocampal neurons and cognitive dysfunction in the developing brain.34 Unfortunately, the underlying mechanism by which sevoflurane induces hippocampal neurodegeneration is still undefined.
Accumulating evidence has well documented the association between neurological impairments induced by sevoflurane and changes in the expression of multiple genes involved in brain development5. It has been well-acknowledged that sevoflurane anesthesia causes alterations in expression levels of microRNAs (miRNAs) in the rat hippocampus.6 miRNAs are a group of short-sequenced, small noncoding RNAs that negatively regulate gene expression through binding to the 3′untranslated region (UTR) of target mRNA, promoting translation inhibition or mRNA degradation. There is striking evidence that miRNAs play a fundamental role in various cellular processes, such as cell death, survival, and differentiation.7 Moreover, emerging evidence has shown that miRNAs are abundantly expressed in various cortical regions, including the hippocampus,8 and play prominent roles in regulating brain development, including neurogenesis and maturation,9 cortical neuropathy, and neurodegenerative diseases.10 Among cortically expressed miRNAs, miR-34a, which belongs to the miR-34 family that is highly conserved among different species, is constitutively expressed in the brain and plays critical roles in many aspects of cortical development and tumorigenesis.11 miR-34a has been reported to negatively regulate ketamine-induced hippocampal apoptosis and memory impairment.12 However, the role of miR-34a in sevoflurane-induced hippocampal neurodegeneration remains largely unclear.
In the present study, we aimed to explore the role of miR-34a in sevoflurane-induced neurodegeneration in the hippocampus and to further investigate the association between miR-34a and the Wnt/β-catenin pathway.
The animal procedures were approved by the Institutional Animal Care and Use Committee at School of Medicine of Shandong University. C57/BL6 mice (male; age, 7 days; average weight, 16–17 g) were purchased from Shanghai Laboratory Animal Center, Chinese Academy of Sciences (Shanghai, China). All animals were maintained under standard laboratory conditions (12-h light-dark cycle, 21±1℃, and 60% humidity), with free access to water and food.
Mice were randomly divided into control (n=15), sevoflurane (n=15), and sevoflurane+miR-34a interference lentivirus vector (LV-anti-miR-34a) groups (n=15). The rats in the control group were placed into the plastic container and exposed to simply humidified 50% O2 balanced by N2 for 2 h/day over 3 consecutive days. Rats in the sevoflurane group were placed in the same plastic container and inhaled 2.3% sevoflurane (Sigma-Aldrich, St Louis, MO, USA) in the same mixed air for 2 h/day over 3 consecutive days at a gas flow of 2 L/min. The anesthetic concentration in expired gas in the container was monitored using a gas monitor (Detex Ohmeda, Inc., Louisville, CO, USA). An external heating device (NPS-A3 heated device; Midea Group, Beijiao, China) was used to maintain the temperature between 37℃ and 38℃ in the container. For the sevoflurane+LV-anti-miR-34a group, LV-anti-miR-34a was purchased from RiboBio (Shanghai, China) and injected intracerebroventricularly into the mouse hippocampus on the left lateral cerebral ventricles using a Hamilton syringe at a total volume of 2 µL of lentiviruses after anesthesia on the first day of exposure. Transfection efficiencies were analyzed by qRT-PCR.
At the age of 2 months, the spatial memory ability of the mice was evaluated by the Morris water maze (MWM) test. The MWM consisted of a circular pool (200 cm diameter and 60 cm height) filled with warm water (25±1.0℃) to a depth of 1 cm above the top of an invisible platform (1.5 cm×1.5 cm). The MWM test began by placing the rat at a random starting position, facing the pool wall, allowing them to swim to a hidden platform within 120 s, followed by 30 s of rest on the platform. If the mouse failed to find the platform within 120 s, the rats were manually placed on the platform for 20 s at the end of the trial. A visual cue of flashing green light was positioned above the platform to guide the mice to swim to the target. A computerized tracking/analyzing video system was positioned to record the escape latency of animals (the time from dipping into the water to finding the hidden platform). In the acquisition phase, five training sessions per day were conducted on 5 continuous days and probe trails were performed on the 5th day after anesthesia. The time to reach the platform was used as a measure of spatial memory and learning ability.
Hippocampal apoptosis was detected by an in situ cell death detection kit (Roche, Indianapolis, IN, USA). Mice were killed under anesthesia with pentobarbital sodium (80 mg/kg), and fresh hippocampal tissues were collected, fixed with 4% polyformaldehyde, conventionally dehydrated, embedded in paraffin, and cut into 10-µM sections. After deparaffinization, the sections were incubated with proteinase K solution at 37℃ for 10 min. Next, the sections were treated with 0.3% H2O2 in methanol for 10 min and incubated with the terminal deoxynucleotidyltransferase mediated dUTP-biotin nick end labeling (TUNEL) reaction mixture for 60 min at 37℃ in the dark. The sections were then washed with PBS three times and incubated with 4′,6-diamidino-2-phenylindole (DAPI). The number of TUNEL-positive nuclei was counted in five random fields under ×400 magnification using a fluorescence microscope (Fluoview FV1000, Olympus, Tokyo, Japan). Neuronal apoptosis was determined by comparing the number of TUNEL-positive nuclei with the number of DAPI-positive nuclei.
Hippocampi were dissected from neonatal C57BL/6 mice pups, and the hippocampal neurons were dissociated by trypsinization and trituration. The dissociated cells were filtered and centrifuged to obtain single layer cells. The cells were seeded onto poly-D-lysine-coated Petri dishes with Neurobasal Medium (Invitrogen, Carlsbad, CA, USA) supplemented with 2% B27 plus glucose (4.5 g/L), 0.25% Glumax, and 1% penicillin/streptomycin (Sigma-Aldrich). Three days after planting, 5 µg/mL of cytosine arabinoside C (araC; Sigma-Aldrich) was added into the neuronal cultures for 24 h to prevent the proliferation of glial cells. Hippocampal neurons were cultured for 14 days in a humidified incubator at 37℃ under 5% CO2, and half volume of medium was replaced every three days with fresh medium. Human embryonic kidney 293 (HEK293) cells were obtained from the American Type Culture Collection (ATCC, Manassas, VA, USA) and routinely cultured in Dulbecco's Modified Eagle's Medium (DMEM; Gibco, Rockville, MD, USA) in a humidified incubator at 37℃ under 5% CO2.
To mimic the conditions of anesthesia by sevoflurane in vitro, hippocampal neurons were placed in an airtight humidified incubator chamber (Billups-Rothenberg, Del Mar, CA, USA) and exposed to 1% sevoflurane with 94% air and 5% CO2. Sevoflurane was delivered by a calibrated vaporizer (Datex-Ohmeda, Helsinki, Finland) to the chamber at 10 L/min for 6 h. Control cells were exposed to normal conditions with 95% air and 5% CO2.
Additionally, miR-34a mimic (miR-34a), miRNA negative control (miR-NC), miR-34a inhibitor (miR-34a), inhibitor negative control (anti-miR-NC), pcDNA-Wnt1 (Wnt1), pcDNA control vector (pcDNA), small interfering RNA (siRNA) against Wnt1 (si-Wnt1), and siRNA negative control (si-NC) were obtained from Shanghai GeneChem, Inc. (Shanghai, China). Hippocampal neurons were transfected with these oligonucleotides or plasmids using Lipofectamine 2000 (Invitrogen) prior to exposure to sevoflurane.
Wild-type (WT) fragments of Wnt1 3′UTR harboring the miR-34a binding sites, as well as the mutated (MUT) seed sequence, were purchased from Guangzhou RiboBio Co., Ltd. (Guangzhou, China) and inserted into the Xho I and Not I sites of psiCHECK- 2 vector (Promega Corporation, Madison, WI, USA) to generate Wnt1-WT and Wnt1-MUT. For the luciferase reporter assay, HEK293 cells were seeded into 24-well plates and cotransfected with 100 ng of Wnt1 3′UTR reporter plasmid constructs and 50 nM miR-34a or miR-NC using Lipofectamine 2000 (Invitrogen). Cells were harvested at 48 h posttransfection, and relative luciferase activity was tested with the Dual-Luciferase Reporter Assay System (Promega Corporation).
Co-immunoprecipitation (Co-IP) of microRNA ribonucleoprotein complex (miRNP) with anti-Argonaute 1 (anti-Ago1; Abcam, Cambridge, MA, USA) or IgG (Sigma) was performed as previously reported.13 Hippocampal neurons at 80% confluence were harvested and lysed by RNA immunoprecipitation (RIP) lysis buffer. Afterwards, cell extracts were incubated with RIP buffer containing magnetic beads conjugated to the human anti-Ago1 (Abcam) or negative control IgG (Sigma) at 4℃. Precipitate was digested with proteinase K with shaking, and the immunoprecipitated RNA was isolated and subjected to qRT-PCR analysis.
Total RNA was extracted from hippocampal tissue or hippocampal neurons using TRIzol (Invitrogen). A total of 1 µg of RNA was reversely transcribed into cDNA first strands by the PrimeScript™ RT reagent kit (TaKaRa, Tokyo, Japan). qPCR was performed to detect the expressions of Wnt1 mRNA and miR-34a using the SYBR Green PCR kit (TaKaRa) and TaqMan Universal PCR Master Mix (Applied Biosystems, Foster City, CA, USA) on the Roche Light Cycler 480 Real-Time PCR System (Roche), respectively. Relative expression levels were calculated using the 2−ΔΔCt method.14 GAPDH and U6 small nuclear RNA were used as the internal controls for RNA and miRNA, respectively.
Hippocampal neurons were seeded on sterilized coverslips and transfected with miR-34a, anti-miR-34a, or respective controls. After 48 h of transfection, cells were washed with PBS and fixed with 4% paraformaldehyde, followed by permeabilization with 0.1% Triton X-100. Then, the cells were blocked with 10% bovine serum albumin for 1 h and incubated with primary antibody against Wnt1 (1:1000 dilution; R&D Systems, Minneapolis, MN, USA) overnight at 4℃. The next day, the cells were washed with PBS and incubated with Alexa Fluor 488-conjugated antibody to rabbit IgG (1:5000; Santa Cruz Biotechnology, Santa Cruz, CA, USA) for 1 h. The coverslips were stained with DAPI, and the cells were visualized using a fluorescence microscope (Fluoview FV1000, Olympus).
The treated hippocampal neurons were collected and lysed with RIPA lysis buffer (Beyotime, Shanghai, China) with protease and phosphatase inhibitors (Roche). After denaturing at 95℃ for 5 min, the protein samples (30 µg/lane) were subjected to 10% SDS-PAGE gels and transferred to nitrocellulose filter membranes (Millipore, Madison, WI, USA). After blocking for 2 h with 5% non-fat dry milk in Tris-buffered saline containing 0.1% Tween-20, the membranes were incubated with primary antibodies against B-cell lymphoma 2 (Bcl-2) (1:1000 dilution; Abcam), bcl-2-like protein 4 (Bax) (1:2000 dilution; Abcam), Wnt1 (1:1000 dilution; R&D Systems), transcription factor-4 (TCF-4) (1:2000 dilution; Thermo Scientific, Rockford, IL, USA), cyclin D1 (1:2000 dilution; Abcam), β-catenin (1:1000 dilution; Abcam), or β-actin (1:2000 dilution; Abcam) at 4℃ overnight, followed by incubation with horseradish peroxidase-conjugated secondary antibody (1:5000; Santa Cruz Biotechnology) at room temperature for 1 h. Protein signals were detected using an ECL detection kit (Amersham Pharmacia Biotech, Uppsala, Sweden) and quantified with Image J software (National Institutes of Health, Bethesda, MD, USA).
Apoptosis of treated hippocampal neurons were detected using the Annexin V-fluorescein isothiocyanate (FITC)/propidium iodide (PI) Apoptosis Detection Kit (BD Biosciences, Franklin Lakes, NJ, USA) and analyzed using a FACScan flow cytometer (BD Biosciences).
All results are shown as the mean±standard deviation (SD) of three independent biological experiments. Statistical analysis was performed using GraphPad Prism (version 6.0; GraphPad Software, Inc., La Jolla, CA, USA). Comparison among multiple groups was performed using one-way analysis of variance or Student's t-test. *p<0.05, **p<0.01, or ***p<0.001 was considered to indicate statistically significant differences.
To evaluate the effects of miR-34a on sevoflurane-induced neurodegeneration in vivo, LV-anti-miR-34a was injected intracerebroventricularly into the mouse hippocampus using a Hamilton syringe at a total volume of 2 µL of lentiviruses after sevoflurane exposure. As shown in Fig. 1A, hippocampal miR-34a expression was significantly enhanced in the hippocampus of mice exposed to sevoflurane, compared with the control group (p=0.0004), which was evidently relieved after injection of LV-anti-miR-34a (p=0.0011). After mice received hippocampal injection of lentiviruses and 3 consecutive days of sevoflurane exposure, the spatial memory ability of mice was evaluated by the MWM test. The results implicated that sevoflurane exposure triggered remarkable memory impairment with longer times to locate the hidden platform (p=0.0019), while miR-34a inhibitor distinctly attenuated sevoflurane-induced memory impairment (p=0.0060) (Fig. 1B). The effect of miR-34a on sevoflurane-induced hippocampal apoptosis was assessed by TUNEL assay, and the results demonstrated marked hippocampal apoptosis in the hippocampus upon sevoflurane exposure (p=0.0007). Suppression of miR-34a prominently protected against hippocampal apoptosis induced by sevoflurane (p=0.0016) (Fig. 1C). These results revealed that miR-34a inhibitor greatly protected against sevoflurane-induced hippocampal apoptosis and memory impairment.
Wnt1 has been shown in the literature to be a target of miR-34a.1516 To identify whether Wnt1 is a direct target of miR-34a in our study, we cloned the WT or MUT fragments of Wnt1 3′UTR into psiCHECK-2 vector, as shown in Fig. 2A, and transfected miR-34a or miR-NC into HEK293 cells. Luciferase reporter assay showed that cotransfection with miR-34a and Wnt1-WT dramatically decreased luciferase activity in HEK293 cells (p=0.0003), whereas cotransfection with Wnt1-MUT and miR-34a elicited no significant difference on luciferase activity (Fig. 2B). RIP assay revealed that mRNA of Wnt1 could be specifically recruited to the miRNP complexes isolated using anti-Ago1 antibody following miR-34a expression (p=0.0048) (Fig. 2C), confirming the authentic binding between miR-34a and Wnt1. To explore the regulatory effects of miR-34a on Wnt1 expression, Western blot analysis was performed to determine the protein levels of Wnt1 expression in hippocampal neurons transfected with miR-34a, anti-miR-34a, or corresponding controls. As displayed in Fig. 2D, Wnt1 levels notably declined in miR-34a-transfected hippocampal neurons (p=0.0017), but were substantially elevated in anti-miR-34a-introduced hippocampal neurons (p=0.0062). Immunofluorescence staining also demonstrated that ectopic expression of miR-34a obviously decreased Wnt1 expression, while anti-miR-34a apparently increased Wnt1 expression in hippocampal neurons (Fig. 2E). These data suggested that miR-34a negatively regulates Wnt1 expression via direct binding.
Our study further investigated whether Wnt1 is also involved in the regulation of miR-34a on sevoflurane-induced apoptosis of hippocampal neurons. Flow cytometry analysis demonstrated that sevoflurane administration triggers a substantial increase in the apoptotic rate of hippocampal neurons (p=0.0002) (Fig. 3A). However, miR-34a significantly intensified sevoflurane-induced apoptosis in hippocampal neurons, compared with the miR-NC group (p=0.0042), while this effect was remarkably undermined after overexpressing Wnt1 expression (p=0.0036) (Fig. 3B). In contrast, anti-miR-34a considerably restrained sevoflurane-induced apoptosis in hippocampal neurons with respect to anti-miR-NC (p=0.0013), which was partially restored following transfection with si-Wnt1 (p=0.0024) (Fig. 3C). Moreover, the related proteins levels of apoptosis were measured in sevoflurane-treated hippocampal neurons. Results showed that sevoflurane treatment induced Bax expression and inhibited Bcl-2 level (Fig. 3D). Furthermore, miR-34a exacerbated the effect of sevoflurane on protein abundances, whereas introduction of Wnt1 attenuated miR-34a-mediate apoptosis in sevoflurane- treated hippocampal neurons, compared with their counterparts, respectively (Fig. 3E). However, anti-miR-34a and si-Wnt1 showed the opposite effect on Bax and Bcl-2 expression (Fig. 3F). Collectively, these results indicated that anti-miR-34a impedes sevoflurane-induced apoptosis in hippocampal neurons by upregulating Wnt1.
Since the Wnt/β-catenin pathway is associated with apoptosis, we next analyzed the effects of miR-34a on the Wnt/β-catenin pathway in hippocampal neurons exposed to sevoflurane. As shown in Fig. 4A, sevoflurane treatment dramatically reduced the protein levels of Wnt1 in hippocampal neurons (p=0.0058). The downstream target genes of the Wnt/β-catenin pathway, including TCF-4, cyclin D1, β-catenin, and Wnt1, were also detected by Western blot. These results showed that miR-34a overexpression conspicuously curbed the protein levels of TCF-4 (p=0.0002), cyclin D1 (p=0.0049), β-catenin (p=0.0002), and Wnt1 (p=0.0018), while anti-miR-34a exerted the opposite effects in hippocampal neurons exposed to sevoflurane (p=0.0041, 0.0003, 0.0004, and 0.0014, respectively) (Fig. 4B). These results indicated that anti-miR-34a activates the Wnt/β-catenin pathway by upregulating Wnt1 in hippocampal neurons exposed to sevoflurane.
General anesthetics are used extensively in modern day medicine, making their safety a major health issue. There is growing concern that exposure of the developing brain to commonly used anesthetic agents including sevoflurane can cause widespread neuronal cell death and long-term neurocognitive deficiency.17 For instance, mild exposure to ketamine, or a combination of these drugs, caused a dose-dependent apoptotic neurodegeneration in the infant mouse brain.18 Exposure of 7-day-old postnatal mouse pups to a subanesthetic concentration of sevoflurane triggered neuronal apoptosis in the developing C57BL/6 mouse brain.4 Also, repeated exposure to sevoflurane significantly impaired the learning and memory of older male rats.19 Consistently, our study provided evidence that exposure to 2.3% sevoflurane for 2 h/day over 3 consecutive days in neonatal C57/BL6 mice potentiated memory impairment in the hippocampus, as demonstrated by MWM test. Moreover, sevoflurane exposure caused apoptosis in the hippocampus and hippocampal neurons, as illustrated by TUNEL assay and flow cytometry analysis. These results confirmed the neurodegenerative effects of sevoflurane on neonatal brains.
The exact molecular mechanism underlying the neurotoxicity effect associated with sevoflurane exposure has not yet been thoroughly characterized. It has been proposed that ketamine upregulates hippocampal miR-34a and that inhibition of miR-34a protects against ketamine-induced hippocampal apoptosis and memory impairment in the hippocampus.12 A previous study also reported that miR-34c is involved in sevoflurane-induced neural apoptosis in the hippocampus of developing rat brains.20 Based on these findings, it is reasonable to hypothesize that miR-34a could participate in the regulation of sevoflurane-induced neurodegeneration. In our study, we found that sevoflurane induced miR-34a expression in the hippocampus. It has been well acknowledged that exposure to 2.3% sevoflurane alters miRNA expression patterns in the hippocampus.20 We performed hippocampal injection of LV-anti-miR-34a after anesthesia on the first day of exposure and found that sevoflurane-induced hippocampal apoptosis and that memory impairment was dramatically attenuated after inhibiting miR-34a expression, suggesting that miR-34a plays a crucial role in protecting against sevoflurane-induced neurodegeneration, validating our hypothesis.
The Wnt/β-catenin pathway, which is highly conserved in multicellular eukaryotes, is closely associated with cell apoptosis, neutral stem cell patterning, and cell proliferation and differentiation in different cell types.21 Moreover, the Wnt/β-catenin pathway plays an important role in the regulation of rat hippocampal neurogenesis and neurodegenerative disease.22 It has been proposed that miRNAs can regulate the Wnt/β-catenin pathway by directly targeting Wnt components.23 Previous studies demonstrated that miR-34a suppresses the proliferation and progression of breast cancer by targeting Wnt1 through inactivation of the Wnt/β-catenin pathway.15 Accordingly, we hypothesized whether miR-34a regulates sevoflurane-induced hippocampal neurodegeneration via targeting Wnt1 through the Wnt/β-catenin pathway. As expected, our study identified Wnt1 as a target of miR-34a and demonstrated that miR-34a suppresses Wnt1 expression in hippocampal neurons by luciferase reporter assay, RIP, and immunofluorescence assay. Moreover, rescue experiments demonstrated that forced expression of Wnt1 markedly undermines miR-34a-mediated enhancement of sevoflurane-induced apoptosis of hippocampal neurons, while Wnt1 silencing greatly restores anti-miR-34a-mediated repression of sevoflurane-induced apoptosis of hippocampal neurons, suggesting that miR-34a regulates sevoflurane-induced apoptosis of hippocampal neurons by targeting Wnt1. Our study further showed that sevoflurane suppresses Wnt1 expression in hippocampal neurons. Moreover, increased expression of miR-34a inhibited the protein levels of TCF-4, Cyclin D1, β-catenin, and Wnt1 in hippocampal neurons exposed to sevoflurane, while anti-miR-34a exerted the reverse effects, suggesting that miR-34a inhibitor activates the Wnt/β-catenin pathway by upregulating Wnt1 in hippocampal neurons exposed to sevoflurane. Interestingly, it was previously demonstrated that exposure of pregnant mice to sevoflurane negatively affected the learning and memory abilities of their offspring by inhibition of the Wnt/β-catenin signaling pathway.24 We discerned from these results that miR-34a inhibitor may effectively protect against sevoflurane-induced hippocampal apoptosis through activation of the Wnt/β-catenin pathway by targeting Wnt1. This is consistent with a former study that reported the Wnt/β-catenin signaling pathway to be associated with apoptosis in many conditions.21 Nevertheless, our study failed to provide evidence demonstrating that the Wnt/β-catenin pathway directly regulates hippocampal apoptosis. Hence, research on the effect of the Wnt/β-catenin pathway on hippocampal apoptosis by blocking or activating the pathway is required in the future.
In conclusion, our study provides the first evidence that miR-34a inhibitor attenuates sevoflurane-induced hippocampal apoptosis in vitro and in vivo through activation of the Wnt/β-catenin pathway by targeting Wnt1, providing a novel therapeutic approach to reducing the neurotoxicity of sevoflurane in the developing brain.
Figures and Tables
Fig. 1
miR-34a inhibitor greatly protected against sevoflurane-induced hippocampal apoptosis and memory impairment. After mice received sevoflurane exposure for 2 h/day on 3 consecutive days and subsequent hippocampal injection of lentiviruses, (A) the expression of miR-34a was checked by qRT-PCR in the isolated hippocampi; (B) the spatial memory ability of mice was evaluated by the MWM test; and (C) TUNEL assay was applied to evaluate the apoptosis of hippocampus. **p<0.01 or ***p<0.001.
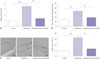
Fig. 2
Wnt1 was a target of miR-34a in hippocampal neurons. (A) Luciferase reporters carrying the fragments of Wnt1 3’UTR containing the WT or MUT miR-34a binding sites. (B) Luciferase activity was measured by luciferase reporter assay in hippocampal neurons after cotransfection with the constructed luciferase reporter plasmids and miR-34a or miR-NC. (C) RIP assay immunoprecipitated against Ago1. (D) The protein level of Wnt1 was detected by Western blot in hippocampal neurons transfected with miR-34a, anti-miR-34a, or matched controls. (E) The expression of Wnt1 was evaluated by immunofluorescence staining in hippocampal neurons transfected with miR-34a, anti-miR-34a, or matched controls. **p<0.01 or ***p<0.001.
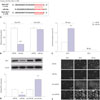
Fig. 3
Anti-miR-34a impedes sevoflurane-induced apoptosis in hippocampal neurons by upregulating Wnt1. (A) Flow cytometry analysis was conducted to analyze the apoptosis of hippocampal neurons exposed to sevoflurane. Hippocampal neurons were transfected with miR-34a, miR-NC, miR-34a+Wnt1, miR-34a+pcDNA, anti-miR-34a, anti-miR-NC, anti-miR-34a+si-Wnt1, or anti-miR-34a+si-NC, followed by treatment with sevoflurane. (B and C) Flow cytometry was then carried out to detect apoptosis of hippocampal neurons. (D–F) The expression of Bax and Bcl-2 protein was investigated in hippocampal neurons. **p<0.01 or ***p<0.001.
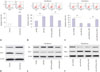
Fig. 4
Anti-miR-34a activates the Wnt/β-catenin pathway by upregulating Wnt1 in hippocampal neurons exposed to sevoflurane. (A and B) Western blot analysis of protein levels of TCF-4, cyclin D1, β-catenin, and Wnt1 in hippocampal neurons after transfection with miR-34a, anti-miR-34a, or respective controls, followed by sevoflurane exposure. **p<0.01 or ***p<0.001.
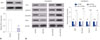
References
1. Bittner EA, Yue Y, Xie Z. Brief review: anesthetic neurotoxicity in the elderly, cognitive dysfunction and Alzheimer's disease. Can J Anaesth. 2011; 58:216–223.


2. Flick RP, Nemergut ME, Christensen K, Hansen TG. Anesthetic-related neurotoxicity in the young and outcome measures: the devil is in the details. Anesthesiology. 2014; 120:1303–1305.
3. Hu N, Guo D, Wang H, Xie K, Wang C, Li Y, et al. Involvement of the blood-brain barrier opening in cognitive decline in aged rats following orthopedic surgery and high concentration of sevoflurane inhalation. Brain Res. 2014; 1551:13–24.


4. Zhang X, Xue Z, Sun A. Subclinical concentration of sevoflurane potentiates neuronal apoptosis in the developing C57BL/6 mouse brain. Neurosci Lett. 2008; 447:109–114.


5. Pan Z, Lu XF, Shao C, Zhang C, Yang J, Ma T, et al. The effects of sevoflurane anesthesia on rat hippocampus: a genomic expression analysis. Brain Res. 2011; 1381:124–133.


6. Goto G, Hori Y, Ishikawa M, Tanaka S, Sakamoto A. Changes in the gene expression levels of microRNAs in the rat hippocampus by sevoflurane and propofol anesthesia. Mol Med Rep. 2014; 9:1715–1722.


7. Chen K, Rajewsky N. The evolution of gene regulation by transcription factors and microRNAs. Nat Rev Genet. 2007; 8:93–103.


8. Juhila J, Sipilä T, Icay K, Nicorici D, Ellonen P, Kallio A, et al. MicroRNA expression profiling reveals miRNA families regulating specific biological pathways in mouse frontal cortex and hippocampus. PLoS One. 2011; 6:e21495.


9. Miranda RC. MicroRNAs and fetal brain development: implications for ethanol teratology during the second trimester period of neurogenesis. Front Genet. 2012; 3:77.


10. Goedeke L, Fernández-Hernando C. MicroRNAs: a connection between cholesterol metabolism and neurodegeneration. Neurobiol Dis. 2014; 72 Pt A:48–53.


11. Rokavec M, Li H, Jiang L, Hermeking H. The p53/miR-34 axis in development and disease. J Mol Cell Biol. 2014; 6:214–230.


12. Jiang XL, Du BX, Chen J, Liu L, Shao WB, Song J. MicroRNA-34a negatively regulates anesthesia-induced hippocampal apoptosis and memory impairment through FGFR1. Int J Clin Exp Pathol. 2014; 7:6760–6767.
13. Tan LP, Seinen E, Duns G, de Jong D, Sibon OC, Poppema S, et al. A high throughput experimental approach to identify miRNA targets in human cells. Nucleic Acids Res. 2009; 37:e137.


14. Livak KJ, Schmittgen TD. Analysis of relative gene expression data using real-time quantitative PCR and the 2ΔΔCT Method. Methods. 2001; 25:402–408.


15. Si W, Li Y, Shao H, Hu R, Wang W, Zhang K, et al. MiR-34a inhibits breast cancer proliferation and progression by targeting Wnt1 in Wnt/β-catenin signaling pathway. Am J Med Sci. 2016; 352:191–199.


16. Huang A, Yang Y, Chen S, Xia F, Sun D, Fang D, et al. MiR-34a promotes DCs development and inhibits their function on T cell activation by targeting WNT1. Oncotarget. 2017; 8:17191–17201.


17. Loepke AW, Hansen TG. Is this your (paediatric patient's) brain on (anaesthetic) drugs?: The search for a potential neurological phenotype of anaesthesia-related neurotoxicity in humans. Eur J Anaesthesiol. 2015; 32:298–300.
18. Young C, Jevtovic-Todorovic V, Qin YQ, Tenkova T, Wang H, Labruyere J, et al. Potential of ketamine and midazolam, individually or in combination, to induce apoptotic neurodegeneration in the infant mouse brain. Br J Pharmacol. 2005; 146:189–197.


19. Guo S, Liu L, Wang C, Jiang Q, Dong Y, Tian Y. Repeated exposure to sevoflurane impairs the learning and memory of older male rats. Life Sci. 2018; 192:75–83.


20. Zhou X, Xian D, Xia J, Tang Y, Li W, Chen X, et al. MicroRNA-34c is regulated by p53 and is involved in sevoflurane-induced apoptosis in the developing rat brain potentially via the mitochondrial pathway. Mol Med Rep. 2017; 15:2204–2212.


21. Pai SG, Carneiro BA, Mota JM, Costa R, Leite CA, Barroso-Sousa R, et al. Wnt/beta-catenin pathway: modulating anticancer immune response. J Hematol Oncol. 2017; 10:101.


22. Libro R, Bramanti P, Mazzon E. The role of the Wnt canonical signaling in neurodegenerative diseases. Life Sci. 2016; 158:78–88.

