Abstract
Gastric cancer (GC) is the third leading cause of cancer-related deaths worldwide. GC stem-like cells (GCSCs), with unlimited self-renewal, differentiation, and tumor-regenerating capacities, contribute significantly to the refractory features of GC and have gained increasing attention for their role in GC drug resistance, relapse, and metastasis. Therapies targeting GCSCs seem to be one of the most promising methods to improve the outcomes of GC patients. Extensive investigations have attempted to outline the regulatory mechanisms in GCSCs and to develop GCSCs-targeting therapies with which to diminish GC drug resistance, metastasis and relapse. To the best of our knowledge, there is a lack of reviews summarizing these studies. In this review, we systematically recapitulated findings regarding the regulatory mechanisms of GCSCs, as well as therapies that target GCSCs, hoping to support the development of prognostic biomarkers and GCSCs-targeting anticancer therapies in GC.
Gastric cancer (GC) is one of the five most common malignancies and the third leading cause of cancer mortality worldwide.1 Multidisciplinary treatment has been applied to improve prognosis of patients with advanced stage GC.2 Despite the great progress achieved in treatment, the prognosis of GC patients remains poor, primarily because of frequent relapse, metastasis, and drug resistance; the 5-year overall survival rate of patients with advanced GC is at approximately 25%.3 Thus, further investigations of critical mechanisms in GC relapse, metastasis, and drug resistance are imperative. Recently, cancer stem cell hypotheses are attracting increasing attention, and research suggests that cancer stem-like cells (CSCs) contribute to tumor aggressiveness, metastasis, chemotherapy resistance, and relapse.4 CSCs possess the abilities of self-renewing and differentiating into multiple lineages.5 Since the first identification of CSCs in acute myeloid leukemia, numerous studies have identified the existence of CSCs in breast, brain, and several other types of solid tumors, including GC.678910 In GC, cancer drug resistance, metastasis, and recurrence may be majorly attributed to GC stem-like cells (GCSCs).11
Circulating tumor cells (CTCs) could be considered as progenitors of cancer metastasis and relapse, especially CTCs with stem cell-like properties. In GC, the presence of CD44+ CTCs has been shown to be significantly associated with tumor metastasis and relapse, indicating that CD44+ GC CTCs could act as a prognosis predictor in GC.12 Besides this, stemness-associated genes also appear to be prognostic markers for GC. For example, CD44 shares a positive correlation with malignant transformation, TNM grading, remote metastasis, and relapse of GC, and could act as an independent prognosis factor for GC.13 miR-501-5p, which is crucial in maintaining stemness properties of GCSCs, shares a negative correlation with overall survival of GC patients, demonstrating the potential application of miR-501-5p as a prognosis predictor.14 Some preclinical studies have proposed the feasibility of GCSC-targeting therapies through inhibiting stemness-associated genes, which may pave way for its clinical application. These investigations of GCSCs provide insights into new prognosis markers and appealing therapeutic strategies for GC. In this review, we summarized current findings of diverse regulatory mechanisms for GCSCs, as well as GCSC-targeting therapies based on stemness-associated genes, which may help us systematically understand GCSCs, as well as the promising future of GCSC-targeting therapies.
Identification and isolation of GCSCs is critical to exploring the underlying mechanisms and functions thereof. The development of experimental techniques has ushered in several methods of use in the isolation of GCSCs from tumor cells.
Several studies have suggested that, when cultured in serum-free suspension culture medium supplemented with growth factors, cancer stem cells can form spheroid cells and maintain self-renew characteristics, whereas non-CSCs cannot survive. Sphere-forming assay is regarded as a convenient way to obtain CSCs, as spheroid cells exhibit stemness properties, such as extensive proliferation, persistent self-renew, and increasing expression of CSC-related genes (e.g., CD44, OCT4, Sox2, ABCG2, and Nanog).151617 However, as a result of the lack of stimulus from the microenvironment, the properties of stem cells may change in the sphere-forming process. Side population (SP) cell isolation using flow cytometry is feasible for enriching GCSCs, based on the CSC property of spitting out the nucleic acid dye Hoechst 33342, which can emit fluorescence at specific wavelengths when stimulated by ultraviolet light.18 SP cells in GC cells appear to be more resistant to 5-fluorouracil (5-FU) and doxorubicin and to show increased tumor initiating capacity after transplanted in immune-deficient NOG mice.19 However, it is worth noting that Hoechst 33342 is toxic to cells, which may negatively affect the accuracy of experiments. Xue, et al.20 reported that vincristine-preconditioned GC cells exhibit significantly increased tumor sphere formation ability (tumor formation ability in vivo and differentiation ability in vitro), as well as increased expression of stemness associated genes, such as CD44, Sox2, Oct4, and Musashi-1. This indicates that vincristine treatment may be used to enrich GCSCs.
Several GCSC-specific cell surface markers have been applied to isolate GCSCs through fluorescence-activated cell sorting (FACS) or magnetic-activated cell sorting. Despite controversy regarding the specificity of markers in isolating GCSCs, increasing evidence has confirmed the feasibility of surface markers for enriching GCSCs. CD44 was the first GCSC surface marker identified in GC cells, playing a critical role in tumor cell response to their microenvironment. According to research, 2×104−3×104 CD44+ GC cells generate tumors in both the skin and stomach of SCID mice after 8–12 weeks, while 3×104−10×104 CD44− GC cells cannot generate tumors, indicating the greater tumorigenicity of CD44+ GC cells. Moreover, CD44+ GC cells also exhibit increased tumor sphere formation ability, chemoresistance, and radio-resistance.1021 Zhang, et al.22 reported that concurrent CD44/CD24 expression may be used for enriching CSCs in GC, as CD44+/CD24+ cancer cells possess the abilities of self-renewing, producing differentiated progeny, and higher tumorigenesis, compared with CD44−/CD24− counterparts. Additionally, CD44+/CD54+ GC cells derived from human GC tissue have been shown to be capable of self-renew and to show increased tumor formation ability in immunodeficient mice. CD44+/CD54+ cells isolated from peripheral blood-derived, CD44+, spheroid GC cells also show increased self-renewal ability, indicating that CD44+/CD54+ could be used for identifying GCSCs both in tumor tissues and in peripheral blood.23 The RNA-binding protein Musashi-1 plays a critical role in maintaining the undifferentiated state of stem cells,24 and concurrent Musashi-1/CD44 expression can also be used for enriching GCSCs. Xu, et al.25 reported CD44/Musashi-1 as GCSCs-enriching surface markers and demonstrated that CD44+/Musashi-1+ GC cells exhibit increased levels of proliferation, self-renew ability, and drug resistance. EpCAM, combined with CD44, can also be used for enriching GCSCs.26 Several other molecules, such as CD90, CD71, CD133, and lgr5, have also been reported to possess the potential to be candidate surface markers for GCSCs.11272829
Several aberrantly expressed mRNAs and proteins in GCSCs appear to play vital roles in GCSCs maintenance. CD44, which is regarded as a stem cell-surface marker, also serves as a driving factor in the development of GCSCs. Zhang, et al.30 defined a p-ERK mediated positive feedback loop between CD44 and Oct4 in GCSCs that was engaged in the maintenance of stemness properties of GC cells, such as drug resistance, higher tumorigenicity, and metastasis ability. Meanwhile, CD44 variant (CD44v) generated through alternative mRNA splicing also contributes to maintenance of GC cells. xCT, a light-chain subunit of cystine-glutamate exchange transporter, plays an essential role in intracellular redox balance through determining cystine uptake and glutathione synthesis.31 Ishimoto, et al.32 reported that CD44v can interact with and stabilize xCT to increase intracellular glutathione content, in turn contributing to increased resistance of GC cells to oxidative stress. In clinical research, patients with advanced GC who were treated with sulfasalazine, an inhibitor of xCT, showed reduced levels of CD44v-positive stem-like cells, indicating the possibility that administration of sulfasalazine, in combination with chemotherapies, may improve the prognosis of GC patients.33 Another stemness-associated mRNA-Sox2 is increased in GCSCs and contributes significantly to spheroid colony formation and doxorubicin resistance.34 The stemness-associated gene BMI1 is overexpressed in spheroid cells from SGC7901 and MKN45 cell lines. BMI1 shares a positive correlation with several stem cell markers, such as Oct4, Sox2, Nanog, CD44, and CD133. BMI1 exerts its positive regulatory effects on stemness properties through Akt/NF-κB signal pathway-mediated miR-21 activation.35 It was reported that knock-down of ubiquitin-specific protease 22 (USP22) can be an effective approach to inhibit GC stem cell properties through downregulating BMI1 expression, as USP22 can stabilize BMI1 protein to increase GC stem cell properties.36 Various studies have defined the regulatory role of classic stemness-associated signal pathways in the maintenance of GCSCs (Fig. 1). The significant role of the Wnt/β-catenin pathway in maintaining stemness properties is gaining increasing attention.37 It was reported that SLC34A2-induced activation of Wnt/β-catenin signaling was responsible for the self-renewal of CD44+ GCSCs and chemo-resistance. Further investigation showed that SLC34A2 promotes miR-25 expression via binding to the promoter region of miR-25, which could directly inhibit GSK3β expression and further activate Wnt/β-catenin signaling.38 The Notch1 signaling pathway has been found to be activated in CD44+ GC cells. The inhibition of Notch1 by γ-secretase can further suppress self-renewal, tumor-initiating, and migration abilities of CD44+ GC cells, as well as chemotherapy resistance, indicating that Notch1 signaling is essential for the maintenance of GCSCs.39 The Sonic Hedgehog pathway, which is essential for maintenance of stemness properties,40 is activated in CD44+/Musashi-1+ GC cells. It can induce activation of the GLI1/ABCG2 pathway, further contributing to increased self-renew ability and resistance to doxorubicin.25 GLI1 expression also contributes to GCSCs' intrinsic tolerance of CDDP through transcriptional activation of ABCG2.41 Genetically or pharmacologically inhibition of Hedgehog has been found to decrease stem cell-like properties, such as spheroid colony formation, anchorage-independent growth, and chemotherapy resistance.42
Non-coding RNAs (ncRNAs), which do not possess protein-coding potential, are briefly classified into two categories: small RNA with no more than 200 nucleotides and long non-coding RNA (lncRNA) that are longer than 200 nucleotides.4344 Accumulating evidence has demonstrated the regulatory function of microRNA (miRNA) in GC stem cells (Table 1). Using miRNA microarray analysis, Zhang, et al.45 defined the differential miRNA expression patterns between FACS-sorted SP and major population cells in MKN-45 cells. miRNA expression patterns between the spheroid body-forming cells and the parental cells of the MKN-45 cell line also exhibited significant differences. Some target genes of these miRNAs are associated with pivotal signal pathways of stemness regulation, such as TGF-β/Smad, Wnt/β-catenin, and Notch signal pathways.4647 This evidence indicates that dysregulated miRNAs expression also participates in the regulation of GCSC stemness properties.
miR-196-5p, which is upregulated in CD44+ GCSCs, has been shown to strengthen the self-renewal ability of CD44+ cells through directly inhibiting Smad4 expression by targeting 3′-UTR of Smad4.48 Yu, et al.47 also reported the critical role of miR-106b in the maintenance of stemness properties of GC cells by activating the TGF-β/Smad signaling pathway. Several miRNAs reportedly exert regulatory effects on GCSCs through the Wnt/β-catenin pathway. For instance, miR-483-5p can promote growth and self-renewal of GCSCs through activation of the Wnt/β-catenin pathway.49 miR-501-5p activates Wnt/β-catenin signaling by directly downregulating DKK1, NKD1, and GSK3β expression, contributing to enhanced stemness properties of GC cells.14 The expression of miR-19b, miR-92a, and miR-20a gradually decrease during the differentiation of CD44+/EpCAM+ GC sphere-forming cells. miR-19b/20a/92a have been found to significantly strengthen drug resistance in GCSCs, as well as self-renewal capability and tumorigenicity. The possible underlying mechanism thereof may be that miR-19b/20a/92a can directly inhibit two Wnt pathway inhibitors, E2F1 and HIPK1, further activating the Wnt/β-catenin pathway. Interestingly, analyzing the expression level of miR-92a in stomach tissue of 97 GC patients has demonstrated that miR-92a could act as an independent prognosis factors in GC.5051 Meanwhile, the Notch signaling pathway can also be modulated by miRNA, further regulating stemness properties of GCSCs. N2IC is the activated form of Notch2 receptor, which can enhance gastric carcinogenesis through upregulating cyclooxygenase-2.52 Huang, et al.53 outlined the negative reciprocal regulation loop between N2IC, Ets1, and miR-23b in GCSCs, which plays an important role in regulating stemness properties. Through inactivating N2IC and Ets1, miR-23b can inhibit tumor sphere formation ability and decrease the expression of pluripotency genes, including CD44, Nanog, and SOX2. The converse effect may be achieved by overexpression of N2IC and Ets1, which can stimulate the expression of E2F1, further leading to the inhibition of miR-23b.
Numerous studies have demonstrated the role of CSCs in drug resistance, which is regarded as a stem-like property.5455 ABCG2, which is responsible for the maintenance of stem cells through SP regulation for its role in exclusion of Hoechst 33342 dye, contributes to drug resistance in GC.56 miR-132 can strengthen the drug resistance of lgr5+ GCSCs to cisplatin, as miR-132 can maintain activation of CERB by directly inhibiting SIRT1 expression, finally leading to the increased expression of ABCG2.11
As a member of ncRNA, lncRNA manipulates gene expression in cis and trans manners by interacting with DNA, RNA, and protein to regulate chromatin remolding, alternative splicing processes, and transcriptional machinery assembly.57 Wang, et al.58 reported the regulatory effect of lncRNA regulator of reprogramming (ROR) in GCSCs. Overexpressed in CD133-positive GCSCs, lncRNA ROR promotes proliferation and invasion of GSCSs, accompanied by increased expression of stemness genes, such as OCT4, SOX2, and NANOG.
The special microenvironment in which CSCs reside is called the CSC niche (Fig. 2), and stromal cells in the CSC niche play a significant role in the maintenance of the CSC state.59 The interaction between cancer cells and microenvironment may be achieved through complex paracrine and autocrine mechanisms, and disrupting these interactions can result in aberrant cell functions. It was reported that cancer-associated fibroblasts (CAFs) can stimulate stemness properties in GCSCs from the scirrhous GC cell line OCUM-12. Culture media from CAFs has been found to significantly increase the percentage of SP fractions and to upregulate the expression of ABCG2, CD44, Nanog, and Oct4. Research has shown that subcutaneous inoculation of 1.5×104 OCUM-12/SP alone does not result in tumor formation, while co-inoculation of 1.5×104 OCUM-12/SP with CAFs results in tumor formation in mice, indicating the increased tumorigenicity of OCUM-12/SP when co-inoculated with CAFs.60 Furthermore, adipose-derived stromal cells, a type of endocrine tissue, are also reported to promote chemo-sensitivity to 5-FU in CD44+ GCSCs; moreover, the colon formation of CD44+ non-adherent cancer cells were significantly reduced when co-cultured with ADSCs in 5-FU containing soft agar gel.61 Microenvironment stimuli, such as TGF-β, can promote the dedifferentiation of non-CSCs to cells with increased stemness properties.62 Wei, et al.63 demonstrated a similar effect for isoproterenol (stress-associated hormone) in GC cells. Isoproterenol contributes to the stemness properties of GCSCs by inducing activation of STAT3, and STAT3 further suppresses miR-373 expression by binding to its promoter, which consequently increases CD44 expression. Hypoxia is also reported to regulate stem cell differentiation and self-renewal by inducing a set of transcriptional responses and to generate a more aggressive tumor phenotype. This effect is primarily attributed to transcriptional factor hypoxia-inducible factors (HIF).6465 Miao, et al.66 defined an important role of the hypoxic microenvironment in peritoneal milky spots (PMS) in controlling the GC stem cell phenotype during the peritoneal dissemination process. The presence of an elevated GCSCs ratio in the GCC population, shown as enhanced expression of GCSCs-related markers lgr5 and CD44, was confirmed in the hypoxic PMS. Retardance of the regulation of hypoxic microenvironment to GCSCs or decreased expression of HIF-1α in GCSCs cells alleviated GC peritoneal dissemination. All of these indicate that hypoxic PMSs serve to favor GCSC engraftment and self-renewal, further inducing GC peritoneal dissemination through the regulation of HIF-1α.66
Helicobacter pylori infection is regarded as a major pathogenic factor for GC.67 It is reported that CagA-positive H. pylori strains also contribute to the induction of CSC-like properties of GC by activating Wnt/β-catenin signaling. CagA-positive H. pylori-infected GC cells exhibit increased spheroid formation ability, as well as increased expression of Nanog, Oct4, lgr5, and CD44. Immunofluorescence stain and luciferase reporter assay confirmed that CagA-positive H. pylori can induce nuclear accumulation of β-catenin and enhance its transcriptional activation, which is essential for the maintaining of stemness properties of GCSCs.68
The frequent recurrence of GC may be a result of anti-cancer therapy that primarily targets non-GCSCs.69 For their role in drug resistance and tumor metastasis, CSCs contribute significantly to the dismal outcomes of cancer treatment. Cancer therapies that target critical molecules in CSC maintenance appear to be theoretically feasible in diminishing GCSCs to improve the outcome of GC patients.70 Several novel therapies targeting stemness-associated genes have been proposed for specifically eradicating GCSCs (Fig. 3).
Nanotechnologies may contribute to cancer prevention, diagnosis, and treatment due to their unprecedented capability of loading diagnostic and therapeutic compounds at specific sites.717273 Due to the critical role of CD44 in GCSCs, antibody-guided therapy targeting CD44 seems to be an effective anticancer strategy. Compared with CD44, its variant isoform CD44v6 has much more restricted distribution in vivo, and several CD44v6-spcecific antibodies have been tested in clinical phase I trials, demonstrating favorable biodistribution and excellent tumor targeting effect.747576 Liang, et al.77 reported that gold nanostars (GNSs)-based PEGylated and CD44v6 monoclonal antibodies-conjugated nanoprobes (GNS-PEG-CD44v6) show excellent biocompatibility, specific affinity to GCSCs, and photothermal conversion efficiency. Synthetic GNS-PEG-CD44v6 may induce CD44-positive GCSCs clearance by interfering with the function of molecular CD44, exerting regulatory effects on tumor formation. Mice with GC xenograft subcutaneous tumors were injected with 150 µL of GNS-PEG-CD44v6 and then were exposed to near-infrared laser irradiation every two days for two weeks, demonstrating reduced tumor volumes, compared with the control group. These indicate the potential application of GNS-PEG-CD44v6 in GCSCs targeting therapy.
Contemporary epigenetic tools, RNA interference-inducing small molecules, are also under development to suppress stemness-associated genes expression. miR-34a, which is often downregulated in tumor tissue, is a negative regulator of CD44.7879 Nanovesicle-mediated PLI/miR-34a (NVs/miR-34a) delivery systems were shown to greatly increase delivery efficiency in vivo, as well as CD44 knockdown effects in GC cells with favorable biocompatibility. Compared with PLI/miR-34a, treatment with NVs/miR-34a significantly suppressed CD44 expression and induced apoptosis of tumor cells in vivo, a promising anti-tumor effect, via targeting GCSCs with a nanoscale stable, miR-34a delivery system.80 Co-delivery of nucleic acid and anticancer drugs also seems to be a promising method to diminish CSCs and non-CSCs. The stable gelatinases-stimuli nanoparticles containing miR-200c and docetaxel (DOC) showed superior tumor cell targeting ability and synergistic antitumor effects. Treatment with miR-200c/DOC nanoparticles was found to improve E-cadherin expression and to decrease CD44 expression, lowering tumor formation capacity.81 Adenoviral vector carrying Bmi-1 shRNA can selectively silence Bmi-1 expression, suppressing self-renewing ability and chemotherapy resistance in GC cells. Despite the direct inhibitory effect on tumor activity, adenoviral vector carrying Bmi-1 shRNA can also indirectly suppress tumorigenesis though inhibiting angiogenesis via modulating the PTEN/Akt/VEGF pathway.82
Apart from specifically targeting antigens or signaling pathways that significantly contribute to the self-renewing ability of GCSCs, differentiation therapy is also a promising strategy to inhibit CSCs proliferation by helping them differentiate into their no longer tumorigenic terminal progeny. ATOH1, a basic helix-loop-helix transcription factor, plays an essential role in inducing the differentiation of intestinal epithelial cells.83 Han, et al.84 reported that the expression of ATOH1 is markedly increased during the differentiation of GCSCs. Lentivirus-mediated overexpression of ATOH1 in GCSCs decreases the expression levels of various stemness genes, accompanied with increased expression of various differentiation markers, which in turn decreases sphere formation ability of GCSCs in vitro, as well as tumor formation ability in vivo. This result suggested the potential application of ATOH1 as a differentiation therapy to eradicate GCSCs. All-trans retinoic acids (ATRA), firstly introduced in the late 1980s to treat acute promyelocytic leukemia, have also been shown to be effective in inducing the differentiation of GCSCs, reflected as increased expression of gastric epithelial differentiation markers, such as cytokeratins, Mucin 6, and TFF3, as well as decreased expression of stemness-associated genes after ATRA treatment in tumorspheres. Flow-cytometry analysis also revealed significant decreases in the percentage of CD44+ and ALDH+ cells. ATRA-pretreated tumorspheres do not generate tumor growth when injected into immunodeficient NSG mice. Tumor-bearing mice were found to show noticeably inhibited tumor growth when treated with 33 µmol/kg of ATRA; meanwhile, GC cells isolated from the remaining treated tumors formed fewer tumorspheres in vitro, suggesting a decrease in the number of CSCs as a result of ATRA treatment. All these indicate that ATRA may be used to induce differentiation of GCSCs to eliminate GCSCs.85
In addition to the above, inhibiting GCSCs by impairing crosstalk between GCSCs and their microenvironment should be highlighted. Several studies have proposed the possibility of antiangiogenic drugs being applied to arrest tumor growth by disrupting supportive vascular niche-based microenvironment, which is essential for the maintenance of CSCs, and to help overcome traditional chemotherapy resistance.8687 However, whether this therapy is applicable in eradicating GCSCs remains largely unknown, and further experimentation regarding microenvironment-based GCSCs targeting therapies, especially anti-angiogenesis therapy, is in urgent need.
Great achievements have been made in determining the molecular mechanisms involved in GCSC maintenance. Stemness-associated mRNAs, proteins, ncRNAs, and the tumor microenvironment play crucial roles in regulating stemness properties and exhibit the potential to be applied as prognosis markers or specific targets for GC patients. However, there is still a need for better understanding of regulatory mechanisms related with the fact that CSCs remain in a dynamic state that is influenced by their interaction with the tumor microenvironment. Further comprehension of the interactions between CSCs and its niche may provide valuable clues on CSCs-targeting therapies. Accumulating evidence has demonstrated the critical roles of GCSCs in drug resistance, tumor metastasis, and relapse. Stemness-associated genes, which contribute to the development of CSCs, are also closely correlated with unfavorable outcomes in GC patients. These studies indicate the importance of GCSCs in GC, and targeted elimination of GCSCs seems to be a promising method with which to improve the outcome of patients with malignant disease. Differentiation therapies, nanotechnology, and contemporary epigenetic tools-based therapies, specifically targeting protein-coding genes or ncRNAs, which are essential to the maintenance of GCSCs stemness properties, appear to hold promising value in inhibiting GCSCs. Future studies are needed to evaluate their in vivo effects and to determine whether they can improve GC patient prognosis. A recent study demonstrated the critical role of lgr5+ CSCs in the development and maintenance of colorectal cancer-derived liver metastases. Targeting lgr5+ CSCs may achieve great effects in inhibiting liver metastases, although merely diminishing CSCs is not enough to lead to primary tumor regression.88 This indicates that the combination of traditional treatment with CSCs-targeting therapy may be a reasonable strategy with which to reduce drug resistance, recurrence, and metastasis and to improve the prognosis of patients with cancer. However, there is still much work to be done in order to achieve clinical application of GCSC targeting therapies. Moreover, further work is needed to identify biomarkers that can better identify patient subpopulations who show upregulated CSC pathways, and these patients are more likely to respond to combined therapies of CSC-targeting drugs with chemotherapies. Additionally, normal stem cells share some stemness-associated genes in common with GCSCs, and how to selectively target GCSCs without affecting normal tissue stem cells is still a great conundrum and remains to be explored in further studies.
Figures and Tables
![]() | Fig. 1Three signal pathways contribute to stemness properties of gastric cancer stem-like cells: Wnt/β-catenin signal pathway, Notch signal pathway, and Hedgehog signal pathway. (A) Wnt/β-catenin signal pathway: Wnt binds to its receptor-Frizzled to activate Dsh protein. The activated Dsh protein enhances the phosphorylation of GSK3β (a component of the cytoplasmic complex that promotes phosphorylation of β-catenin and its degradation), which inhibits the ability of GSK3β, further causing the accumulation of free and unphosphorylated β-catenin in the cytoplasm that is then translocated to the nucleus. In the nucleus, β-catenin binds to TCF/LEF to promote downstream target genes expression. (B) Notch signal pathway: Ligand binding-induced Notch activation causes γ-secretase (including Presenilin and Nicastrin) to cleave Notch COOH-terminal fragment to release NICD into the cytoplasm. Then, NICD translocates to the nucleus to interact with SKIP and CSL, which lead to SMRT/HDACs dissociation, further converting CSL to a transcriptional activator to initiate downstream gene expression. (C) Hedgehog signal pathway: Ptc-induced inhibition of Smo is reversed by Hh binding with Ptc, leading to the release of the complex of GLI (GLI/SUFU/SKT36) from microtubules, with GLI protein entering the nucleus to transcriptionally activate downstream target genes. |
![]() | Fig. 2GCSC niche. CAF, cancer-associated fibroblast; GCSC, gastric cancer stem-like cell; ECM, extracellular matrix. |
Table 1
ncRNAs Participating in the Regulation of GCSCs
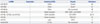
ncRNA | Expression | Enriching GCSC | Targets | Reference |
---|---|---|---|---|
miR-196-5P | ↑ | CD44+ | Smad4 | 48 |
miR-106b | ↑ | CD44+ | Smad7 | 47 |
miR-483-5p | ↑ | CD44+ | Not mentioned | 49 |
miR-501-5p | ↑ | Sphere formation | DKK1, NKD1, and GSK3β | 14 |
miR-19b, miR-92a, and miR-20a | ↑ | CD44+/EpCAM+ | E2F1,HIPK1 | 5051 |
miR-23b | ↓ | Sphere formation | N2IC,Ets1 | 53 |
miR-132 | ↑ | Lgr5+ | SIRT1 | 11 |
lncRNA ROR | ↑ | CD133+ | Not mentioned | 58 |
References
1. Torre LA, Bray F, Siegel RL, Ferlay J, Lortet-Tieulent J, Jemal A. Global cancer statistics, 2012. CA Cancer J Clin. 2015; 65:87–108.


2. Takahashi T, Saikawa Y, Kitagawa Y. Gastric cancer: current status of diagnosis and treatment. Cancers (Basel). 2013; 5:48–63.


4. Yu Z, Pestell TG, Lisanti MP, Pestell RG. Cancer stem cells. Int J Biochem Cell Biol. 2012; 44:2144–2151.


5. Dalerba P, Cho RW, Clarke MF. Cancer stem cells: models and concepts. Annu Rev Med. 2007; 58:267–284.


6. Bonnet D, Dick JE. Human acute myeloid leukemia is organized as a hierarchy that originates from a primitive hematopoietic cell. Nat Med. 1997; 3:730–737.


7. Al-Hajj M, Wicha MS, Benito-Hernandez A, Morrison SJ, Clarke MF. Prospective identification of tumorigenic breast cancer cells. Proc Natl Acad Sci U S A. 2003; 100:3983–3988.


8. Singh SK, Clarke ID, Terasaki M, Bonn VE, Hawkins C, Squire J, et al. Identification of a cancer stem cell in human brain tumors. Cancer Res. 2003; 63:5821–5828.
9. Ricci-Vitiani L, Lombardi DG, Pilozzi E, Biffoni M, Todaro M, Peschle C, et al. Identification and expansion of human colon-cancer-initiating cells. Nature. 2007; 445:111–115.


10. Takaishi S, Okumura T, Tu S, Wang SS, Shibata W, Vigneshwaran R, et al. Identification of gastric cancer stem cells using the cell surface marker CD44. Stem Cells. 2009; 27:1006–1020.


11. Zhang L, Guo X, Zhang D, Fan Y, Qin L, Dong S, et al. Upregulated miR-132 in Lgr5+ gastric cancer stem cell-like cells contributes to cisplatin-resistance via SIRT1/CREB/ABCG2 signaling pathway. Mol Carcinog. 2017; 56:2022–2034.


12. Li M, Zhang B, Zhang Z, Liu X, Qi X, Zhao J, et al. Stem cell-like circulating tumor cells indicate poor prognosis in gastric cancer. Biomed Res Int. 2014; 2014:981261.


13. Wang T, Ong CW, Shi J, Srivastava S, Yan B, Cheng CL, et al. Sequential expression of putative stem cell markers in gastric carcinogenesis. Br J Cancer. 2011; 105:658–665.


14. Fan D, Ren B, Yang X, Liu J, Zhang Z. Upregulation of miR-501-5p activates the wnt/β-catenin signaling pathway and enhances stem cell-like phenotype in gastric cancer. J Exp Clin Cancer Res. 2016; 35:177.


15. Liu J, Ma L, Xu J, Liu C, Zhang J, Liu J, et al. Spheroid body-forming cells in the human gastric cancer cell line MKN-45 possess cancer stem cell properties. Int J Oncol. 2013; 42:453–459.


16. Liu J, Ma L, Xu J, Liu C, Zhang J, Liu J, et al. Co-expression of CD44 and ABCG2 in spheroid body-forming cells of gastric cancer cell line MKN45. Hepatogastroenterology. 2013; 60:975–980.
17. Liu J, Wang L, Ma L, Xu J, Liu C, Zhang J, et al. Significantly increased expression of OCT4 and ABCG2 in spheroid body-forming cells of the human gastric cancer MKN-45 cell line. Oncol Lett. 2013; 6:891–896.


18. Hirschmann-Jax C, Foster AE, Wulf GG, Nuchtern JG, Jax TW, Gobel U, et al. A distinct “side population” of cells with high drug efflux capacity in human tumor cells. Proc Natl Acad Sci U S A. 2004; 101:14228–14233.


19. Fukuda K, Saikawa Y, Ohashi M, Kumagai K, Kitajima M, Okano H, et al. Tumor initiating potential of side population cells in human gastric cancer. Int J Oncol. 2009; 34:1201–1207.


20. Xue Z, Yan H, Li J, Liang S, Cai X, Chen X, et al. Identification of cancer stem cells in vincristine preconditioned SGC7901 gastric cancer cell line. J Cell Biochem. 2012; 113:302–312.


21. Misra S, Heldin P, Hascall VC, Karamanos NK, Skandalis SS, Markwald RR, et al. Hyaluronan-CD44 interactions as potential targets for cancer therapy. FEBS J. 2011; 278:1429–1443.


22. Zhang C, Li C, He F, Cai Y, Yang H. Identification of CD44+CD24+ gastric cancer stem cells. J Cancer Res Clin Oncol. 2011; 137:1679–1686.


23. Chen T, Yang K, Yu J, Meng W, Yuan D, Bi F, et al. Identification and expansion of cancer stem cells in tumor tissues and peripheral blood derived from gastric adenocarcinoma patients. Cell Res. 2012; 22:248–258.


24. Potten CS, Booth C, Tudor GL, Booth D, Brady G, Hurley P, et al. Identification of a putative intestinal stem cell and early lineage marker; musashi-1. Differentiation. 2003; 71:28–41.


25. Xu M, Gong A, Yang H, George SK, Jiao Z, Huang H, et al. Sonic hedgehog-glioma associated oncogene homolog 1 signaling enhances drug resistance in CD44(+)/Musashi-1(+) gastric cancer stem cells. Cancer Lett. 2015; 369:124–133.


26. Han ME, Jeon TY, Hwang SH, Lee YS, Kim HJ, Shim HE, et al. Cancer spheres from gastric cancer patients provide an ideal model system for cancer stem cell research. Cell Mol Life Sci. 2011; 68:3589–3605.


27. Katsuno Y, Ehata S, Yashiro M, Yanagihara K, Hirakawa K, Miyazono K. Coordinated expression of REG4 and aldehyde dehydrogenase 1 regulating tumourigenic capacity of diffuse-type gastric carcinoma-initiating cells is inhibited by TGF-β. J Pathol. 2012; 228:391–404.


28. Jiang J, Zhang Y, Chuai S, Wang Z, Zheng D, Xu F, et al. Trastuzumab (herceptin) targets gastric cancer stem cells characterized by CD90 phenotype. Oncogene. 2012; 31:671–682.


29. Ohkuma M, Haraguchi N, Ishii H, Mimori K, Tanaka F, Kim HM, et al. Absence of CD71 transferrin receptor characterizes human gastric adenosquamous carcinoma stem cells. Ann Surg Oncol. 2012; 19:1357–1364.


30. Zhang X, Hua R, Wang X, Huang M, Gan L, Wu Z, et al. Identification of stem-like cells and clinical significance of candidate stem cell markers in gastric cancer. Oncotarget. 2016; 7:9815–9831.


31. Lo M, Wang YZ, Gout PW. The x(c)-cystine/glutamate antiporter: a potential target for therapy of cancer and other diseases. J Cell Physiol. 2008; 215:593–602.


32. Ishimoto T, Nagano O, Yae T, Tamada M, Motohara T, Oshima H, et al. CD44 variant regulates redox status in cancer cells by stabilizing the xCT subunit of system xc(−) and thereby promotes tumor growth. Cancer Cell. 2011; 19:387–400.


33. Shitara K, Doi T, Nagano O, Imamura CK, Ozeki T, Ishii Y, et al. Dose-escalation study for the targeting of CD44v+ cancer stem cells by sulfasalazine in patients with advanced gastric cancer (EPOC1205). Gastric Cancer. 2017; 20:341–349.


34. Tian T, Zhang Y, Wang S, Zhou J, Xu S. Sox2 enhances the tumorigenicity and chemoresistance of cancer stem-like cells derived from gastric cancer. J Biomed Res. 2012; 26:336–345.


35. Wang X, Wang C, Zhang X, Hua R, Gan L, Huang M, et al. Bmi-1 regulates stem cell-like properties of gastric cancer cells via modulating miRNAs. J Hematol Oncol. 2016; 9:90.


36. Ma Y, Fu HL, Wang Z, Huang H, Ni J, Song J, et al. USP22 maintains gastric cancer stem cell stemness and promotes gastric cancer progression by stabilizing BMI1 protein. Oncotarget. 2017; 8:33329–33342.


37. Takebe N, Harris PJ, Warren RQ, Ivy SP. Targeting cancer stem cells by inhibiting Wnt, Notch, and Hedgehog pathways. Nat Rev Clin Oncol. 2011; 8:97–106.


38. Zhang L, Guo X, Zhang L, Yang F, Qin L, Zhang D, et al. SLC34A2 regulates miR-25-Gsk3β signaling pathway to affect tumor progression in gastric cancer stem cell-like cells. Mol Carcinog. 2018; 57:440–450.


39. Li LC, Wang DL, Wu YZ, Nian WQ, Wu ZJ, Li Y, et al. Gastric tumor-initiating CD44+ cells and epithelial-mesenchymal transition are inhibited by γ-secretase inhibitor DAPT. Oncol Lett. 2015; 10:3293–3299.


40. Song Z, Yue W, Wei B, Wang N, Li T, Guan L, et al. Sonic hedgehog pathway is essential for maintenance of cancer stem-like cells in human gastric cancer. PLoS One. 2011; 6:e17687.


41. Yu B, Gu D, Zhang X, Li J, Liu B, Xie J. GLI1-mediated regulation of side population is responsible for drug resistance in gastric cancer. Oncotarget. 2017; 8:27412–27427.


42. Yoon C, Park DJ, Schmidt B, Thomas NJ, Lee HJ, Kim TS, et al. CD44 expression denotes a subpopulation of gastric cancer cells in which Hedgehog signaling promotes chemotherapy resistance. Clin Cancer Res. 2014; 20:3974–3988.


44. Beermann J, Piccoli MT, Viereck J, Thum T. Non-coding RNAs in development and disease: background, mechanisms, and therapeutic approaches. Physiol Rev. 2016; 96:1297–1325.


45. Zhang HH, Gu GL, Zhang XY, Li FZ, Ding L, Fan Q, et al. Primary analysis and screening of microRNAs in gastric cancer side population cells. World J Gastroenterol. 2015; 21:3519–3526.


46. Liu J, Ma L, Wang Z, Wang L, Liu C, Chen R, et al. MicroRNA expression profile of gastric cancer stem cells in the MKN-45 cancer cell line. Acta Biochim Biophys Sin (Shanghai). 2014; 46:92–99.


47. Yu D, Shin HS, Lee YS, Lee YC. miR-106b modulates cancer stem cell characteristics through TGF-β/Smad signaling in CD44-positive gastric cancer cells. Lab Invest. 2014; 94:1370–1381.


48. Pan Y, Shu X, Sun L, Yu L, Sun L, Yang Z, et al. miR196a5p modulates gastric cancer stem cell characteristics by targeting Smad4. Int J Oncol. 2017; 50:1965–1976.


49. Wu K, Ma L, Zhu J. miR4835p promotes growth, invasion and selfrenewal of gastric cancer stem cells by Wnt/βcatenin signaling. Mol Med Rep. 2016; 14:3421–3428.


50. Shao Q, Xu J, Guan X, Zhou B, Wei W, Deng R, et al. In vitro and in vivo effects of miRNA-19b/20a/92a on gastric cancer stem cells and the related mechanism. Int J Med Sci. 2018; 15:86–94.


51. Wu Q, Yang Z, Wang F, Hu S, Yang L, Shi Y, et al. MiR-19b/20a/92a regulates the self-renewal and proliferation of gastric cancer stem cells. J Cell Sci. 2013; 126(Pt 18):4220–4229.


52. Tseng YC, Tsai YH, Tseng MJ, Hsu KW, Yang MC, Huang KH, et al. Notch2-induced COX-2 expression enhancing gastric cancer progression. Mol Carcinog. 2012; 51:939–951.


53. Huang TT, Ping YH, Wang AM, Ke CC, Fang WL, Huang KH, et al. The reciprocal regulation loop of Notch2 pathway and miR-23b in controlling gastric carcinogenesis. Oncotarget. 2015; 6:18012–18026.


54. Magee JA, Piskounova E, Morrison SJ. Cancer stem cells: impact, heterogeneity, and uncertainty. Cancer Cell. 2012; 21:283–296.


55. Huntly BJ, Gilliland DG. Leukaemia stem cells and the evolution of cancer-stem-cell research. Nat Rev Cancer. 2005; 5:311–321.


56. Yu B, Gu D, Zhang X, Liu B, Xie J. The role of GLI2-ABCG2 signaling axis for 5Fu resistance in gastric cancer. J Genet Genomics. 2017; 44:375–383.


58. Wang S, Liu F, Deng J, Cai X, Han J, Liu Q. Long noncoding RNA ROR regulates proliferation, invasion, and stemness of gastric cancer stem cell. Cell Reprogram. 2016; 18:319–326.


60. Hasegawa T, Yashiro M, Nishii T, Matsuoka J, Fuyuhiro Y, Morisaki T, et al. Cancer-associated fibroblasts might sustain the stemness of scirrhous gastric cancer cells via transforming growth factor-β signaling. Int J Cancer. 2014; 134:1785–1795.


61. Liu G, Neumeister M, Reichensperger J, Yang RD. Therapeutic potential of human adipose stem cells in a cancer stem cell-like gastric cancer cell model. Int J Oncol. 2013; 43:1301–1309.


62. Chaffer CL, Marjanovic ND, Lee T, Bell G, Kleer CG, Reinhardt F, et al. Poised chromatin at the ZEB1 promoter enables breast cancer cell plasticity and enhances tumorigenicity. Cell. 2013; 154:61–74.


63. Wei B, Sun X, Geng Z, Shi M, Chen Z, Chen L, et al. Isoproterenol regulates CD44 expression in gastric cancer cells through STAT3/MicroRNA373 cascade. Biomaterials. 2016; 105:89–101.


64. Das B, Tsuchida R, Malkin D, Koren G, Baruchel S, Yeger H. Hypoxia enhances tumor stemness by increasing the invasive and tumorigenic side population fraction. Stem Cells. 2008; 26:1818–1830.


65. Pistollato F, Rampazzo E, Persano L, Abbadi S, Frasson C, Denaro L, et al. Interaction of hypoxia-inducible factor-1α and Notch signaling regulates medulloblastoma precursor proliferation and fate. Stem Cells. 2010; 28:1918–1929.


66. Miao ZF, Wang ZN, Zhao TT, Xu YY, Gao J, Miao F, et al. Peritoneal milky spots serve as a hypoxic niche and favor gastric cancer stem/progenitor cell peritoneal dissemination through hypoxiainducible factor 1α. Stem Cells. 2014; 32:3062–3074.


67. Plummer M, Franceschi S, Vignat J, Forman D, de Martel C. Global burden of gastric cancer attributable to Helicobacter pylori. Int J Cancer. 2015; 136:487–490.
68. Yong X, Tang B, Xiao YF, Xie R, Qin Y, Luo G, et al. Helicobacter pylori upregulates Nanog and Oct4 via Wnt/β-catenin signaling pathway to promote cancer stem cell-like properties in human gastric cancer. Cancer Lett. 2016; 374:292–303.


69. Wang JC. Evaluating therapeutic efficacy against cancer stem cells: new challenges posed by a new paradigm. Cell Stem Cell. 2007; 1:497–501.


71. Wang X, Yang L, Chen ZG, Shin DM. Application of nanotechnology in cancer therapy and imaging. CA Cancer J Clin. 2008; 58:97–110.


72. Dong K, Liu Z, Li Z, Ren J, Qu X. Hydrophobic anticancer drug delivery by a 980 nm laser-driven photothermal vehicle for efficient synergistic therapy of cancer cells in vivo. Adv Mater. 2013; 25:4452–4458.


73. Yang X, Liu X, Liu Z, Pu F, Ren J, Qu X. Near-infrared light-triggered, targeted drug delivery to cancer cells by aptamer gated nanovehicles. Adv Mater. 2012; 24:2890–2895.


74. Jang BI, Li Y, Graham DY, Cen P. The role of CD44 in the pathogenesis, diagnosis, and therapy of gastric cancer. Gut Liver. 2011; 5:397–405.


75. Heider KH, Kuthan H, Stehle G, Munzert G. CD44v6: a target for antibody-based cancer therapy. Cancer Immunol Immunother. 2004; 53:567–579.


76. Chen Y, Huang K, Li X, Lin X, Zhu Z, Wu Y. Generation of a stable anti-human CD44v6 scFv and analysis of its cancer-targeting ability in vitro. Cancer Immunol Immunother. 2010; 59:933–942.


77. Liang S, Li C, Zhang C, Chen Y, Xu L, Bao C, et al. CD44v6 monoclonal antibody-conjugated gold nanostars for targeted photoacoustic imaging and plasmonic photothermal therapy of gastric cancer stem-like cells. Theranostics. 2015; 5:970–984.


78. Li XJ, Ren ZJ, Tang JH. MicroRNA-34a: a potential therapeutic target in human cancer. Cell Death Dis. 2014; 5:e1327.


79. Misso G, Di Martino MT, De Rosa G, Farooqi AA, Lombardi A, Campani V, et al. Mir-34: a new weapon against cancer? Mol Ther Nucleic Acids. 2014; 3:e194.


80. Jang E, Kim E, Son HY, Lim EK, Lee H, Choi Y, et al. Nanovesicle-mediated systemic delivery of microRNA-34a for CD44 overexpressing gastric cancer stem cell therapy. Biomaterials. 2016; 105:12–24.


81. Liu Q, Li RT, Qian HQ, Wei J, Xie L, Shen J, et al. Targeted delivery of miR-200c/DOC to inhibit cancer stem cells and cancer cells by the gelatinases-stimuli nanoparticles. Biomaterials. 2013; 34:7191–7203.


82. Zhang X, Guo W, Wang X, Liu X, Huang M, Gan L, et al. Antitumor activity and inhibitory effects on cancer stem cell-like properties of Adeno-associated virus (AAV)-mediated Bmi-1 interference driven by Bmi-1 promoter for gastric cancer. Oncotarget. 2016; 7:22733–22745.


83. Yang Q, Bermingham NA, Finegold MJ, Zoghbi HY. Requirement of Math1 for secretory cell lineage commitment in the mouse intestine. Science. 2001; 294:2155–2158.


84. Han ME, Baek SJ, Kim SY, Kang CD, Oh SO. ATOH1 can regulate the tumorigenicity of gastric cancer cells by inducing the differentiation of cancer stem cells. PLoS One. 2015; 10:e0126085.


85. Nguyen PH, Giraud J, Staedel C, Chambonnier L, Dubus P, Chevret E, et al. All-trans retinoic acid targets gastric cancer stem cells and inhibits patient-derived gastric carcinoma tumor growth. Oncogene. 2016; 35:5619–5628.


86. Calabrese C, Poppleton H, Kocak M, Hogg TL, Fuller C, Hamner B, et al. A perivascular niche for brain tumor stem cells. Cancer Cell. 2007; 11:69–82.

