Abstract
Background
Real-time PCR is more sensitive than microscopic examination for detecting Pneumocystis jirovecii. We compared the performance of two assays for detecting P. jirovecii DNA: the RealStar Pneumocystis jirovecii PCR Kit 1.0 CE (Altona Diagnostics, Hamburg, Germany) and the AmpliSens Pneumocystis jirovecii (carinii)-FRT PCR kit (InterLabService Ltd., Moscow, Russia).
Methods
We used 159 samples from the lower respiratory tract (112 bronchoalveolar lavage [BAL] fluid, 37 sputum, and 10 endotracheal aspirate [ETA] samples) of non-HIV immunocompromised patients. Nested PCR and sequencing were used to resolve discordant results. The performance of the two assays was evaluated according to clinical categories (clinical Pneumocystis pneumonia [PCP], possible PCP, or unlikely PCP) based on clinical and radiological observations.
Results
The positive and negative percent agreement values were 100% (95% confidence interval [CI], 85.4–100%) and 96.6% (95% CI, 90.9–98.9%), respectively, and kappa was 0.92 (95% CI, 0.84–0.99). P. jirovecii DNA load was significantly higher in the clinical PCP group than in the other groups (P<0.05). When stratified by sample type, the positive rate for BAL fluids from the clinical PCP group was 100% using either assay, whereas the positive rate for sputum/ETA samples was only 20%.
Pneumocystis jirovecii is an opportunistic fungal pathogen that causes Pneumocystis pneumonia (PCP) in immunocompromised patients [1]. PCP diagnosis is based on clinical presentation, radiological manifestation, and microbiological evidence [2]. The microbiological diagnosis of PCP relies on histological and microscopic identification of the ascus and/or trophic forms of P. jirovecii from respiratory samples using different stains, such as Giemsa, calcofluor-white, toluidine blue O, and Gomori methenamine silver, direct or indirect immunofluorescence (IF) staining, and β-D-glucan assay [23]. However, microscopy following conventional or IF staining is too insensitive to be reliably used for PCP diagnosis [34]. Various PCR assays, particularly real-time PCR, show improved sensitivity compared with microscopic identification methods [567]. An evidence-based review of molecular assays strongly supports the use of real-time PCR for the diagnosis of PCP, particularly in non-HIV immunocompromised patients [3].
Several commercial real-time PCR assays for molecular diagnosis of PCP have been developed, and a few studies have evaluated their performances [6891011]. The RealStar Pneumocystis jirovecii PCR Kit 1.0 CE (RealStar assay; Altona Diagnostics, Hamburg, Germany) was recently developed and has received Conformit Europe In-Vitro Diagnostic approval [12]. In addition, the AmpliSens Pneumocystis jirovecii (carinii)-FRT PCR kit (AmpliSens assay; InterLabService Ltd., Moscow, Russia) is a well-validated, widely used qualitative real-time PCR assay targeting the mitochondrial large subunit ribosomal RNA gene [8]. We compared the performance of these two assays for detecting P. jirovecii DNA in lower respiratory tract (LRT) samples and according to clinical PCP probability.
This study was conducted at Samsung Medical Center, Seoul, Korea, and was approved by its Institutional Review Board (approval number: 2017-02-151), which waived informed consent from the patients. DNA was extracted from 159 respiratory samples (112 bronchoalveolar lavage [BAL] fluid samples, 37 sputum samples, and 10 endotracheal aspirate [ETA] samples) collected from consecutive patients with respiratory clinical symptoms from November 2015 to March 2016. Patient medical records, including clinical, radiological, and treatment data, were collected retrospectively. Of the 159 patients, 51 (32.1%) had a solid tumor, 45 (28.3%) had a hematologic malignancy, and 21 (13.2%) had undergone stem cell transplantation. A total of 143 and 98 patients had clinical and radiological observations compatible with PCP, respectively. Results of microscopic examination using the Calcofluor-white stain were also collected. Based on the clinical information of each patient, three specialists (a microbiologist and two infectious diseases specialists) independently classified the patients into three categories: clinical, possible, and unlikely PCP. The categories were determined on the basis of the following criteria: (i) compatible clinical symptoms (dyspnea, fever, and nonproductive cough), (ii) underlying immunodeficiency, (iii) compatible radiological findings (chest X-ray or computed tomography findings of interstitial infiltrates), and (iv) response to P. jirovecii-specific treatment. Clinical PCP was defined by the presence of all four criteria in the absence of an alternative diagnosis. This category included both microscopically proven PCP and probable PCP with negative microscopic examination. Possible PCP included patients who met three out of the four criteria with no other cause for pneumonia. Unlikely PCP was defined as the presence of fewer than three criteria. The classification was performed blind to the PCR results.
DNA samples were extracted using the MagNA Pure 96 system (Roche Diagnostics, Mannheim, Germany) with the “Pathogen Universal Protocol” according to the procedure recommended by the manufacturer. Extracted DNA samples were stored at −70℃.
The RealStar and AmpliSens assays were performed in parallel according to the manufacturers' instructions. Briefly, the PCR reaction for the RealStar assay was performed in a total volume of 30 µL (20 µL PCR reaction mixture and 10-µL template DNA). Thermocycling conditions included a two min step at 95℃ followed by 45 cycles of 95℃ for 15 seconds, 58℃ for 45 seconds, and 72℃ for 15 seconds. The assay included an artificial heterologous extrinsic control. The PCR for the AmpliSens assay was performed in a total volume of 25 µL (15 µL PCR reaction mixture and 10-µL template DNA). Thermocycling conditions included a 15 minutes step at 95℃ followed by 45 cycles of 95℃ for 20 seconds and 60℃ for 60 seconds. The human β-globin gene was amplified simultaneously as a heterologous intrinsic control to monitor PCR inhibition. For both real-time PCR assays, amplifications were performed on a CFX96 Real-Time PCR Detection instrument (Bio-Rad, Hercules, CA, USA). Positive fungal template (P. jirovecii DNA) and no-template controls were included in each run. Probes specific for the P. jirovecii and the internal control were labeled with the FAM and JOE fluorophores in the RealStar assay, whereas those in the AmpliSens assay were labeled with JOE/HEX and FAM fluorophores. According to the manufacturer's instructions, a positive test result was defined as a cycle threshold (Ct) ≤35 for the AmpliSens assay, whereas any well-defined exponential amplification in the RealStar assay was considered a positive test result. If the internal control was not amplified, the test was considered invalid. The turnaround time (the interval between PCR reagent set-up and amplification) was similar for both assays (140 minutes for the RealStar assay and 130 minutes for the AmpliSens assay, for 54 samples).
Samples with discordant results between the two assays were confirmed by nested PCR and sequencing according to the protocol described by Tia et al. [13], using two sets of primers (outer primers PJLSUF0 and PJLSUR0 and inner primers PJLSUF1 and PJLSUR1) targeting mitochondrial large subunit ribosomal RNA gene. Amplification conditions for the first round consisted of denaturation at 94℃ for two minutes; 35 cycles of 94℃ for 30 seconds, 55℃ for 30 seconds, and 72℃ for 30 seconds; and a final extension at 72℃ for five minutes. For nested PCR, 25 cycles were performed. Following detection of the 113-bp PCR product by gel electrophoresis and purification of the PCR product, sequencing was performed on an automated ABI Prism 3730 instrument using a BigDye terminator cycle sequencing kit (Thermo Fisher Scientific, Waltham, MA, USA). The primers used for the sequencing reaction were the same as inner primers (PJLSUF1 and PJLSUR1) used for the second round of PCR.
Diagnostic performance was compared between the RealStar and AmpliSens assays by measuring positive/negative percent agreement and kappa coefficients with 95% confidence interval (CI). Normality of the data was tested using the Shapiro-Wilk test. Nonparametric Kruskal-Wallis tests with Tukey post hoc tests were applied to compare variability and means across the three clinical categories. Two-sided P<0.05 was considered statistically significant. A Pearson correlation analysis was performed between assay results. All analyses were performed using the VassarStats website (http://vassarstats.net/) and SPSS version 24 (IBM Corp., Armonk, NY, USA).
A total of 33 and 29 samples were positive according to the RealStar and AmpliSens assays, respectively. The AmpliSens assay generated 14 invalid results, while the RealStar assay did not generate invalid results. Excluding the 14 invalid results, 145 results were included in the comparative analysis. As shown in Table 1, the two assays showed high agreement rates. Discordant results were observed in four samples, all RealStar-positive and AmpliSens-negative. These were confirmed to be negative using nested PCR followed by sequencing.
Although the Ct values of the AmpliSens assay without a standard curve could not be interpreted for quantification, a strong correlation was observed between the Ct values of the AmpliSens assay and quantitative results (log copies/mL) of the RealStar assay (Fig. 1).
Table 2 presents the number of positive and negative results according to patient classification and sample type. Two BAL fluid samples from patients categorized as clinical PCP were positive by direct microscopic examination using the calcofluor-white stain. In the clinical PCP group, the positive rate in BAL fluid samples was 100% for both assays, whereas only 20% showed positive results in sputum/ETA samples. In the possible PCP group, the positive rate range in BAL and sputum/ETA samples was 43.8–46.9% and 20–30%, respectively. In the unlikely PCP group, the positive rate was <10% for all patients, regardless of the molecular method and sample type. The quantitation results of the RealStar assay and the Ct values of the AmpliSens assay by classification are shown in Fig. 2. Results for both assays differed among the three clinical categories (RealStar assay, P=0.001; AmpliSens assay, P=0.007). P. jirovecii DNA load was significantly higher in the clinical PCP group than in the other groups (Fig. 2A). Similarly, the Ct values were significantly lower in the clinical PCP group than in the other groups (Fig. 2B). For BAL samples, differences were also observed between assay results of the three clinical categories (RealStar assay, P=0.002; AmpliSens assay, P=0.004; Fig. 2C and 2D).
Over the past decade, advances in molecular diagnostic testing for detecting P. jirovecii have led to improvements in PCP diagnosis [314]. Numerous studies have shown that PCR assays are more sensitive than non-PCR assays [1516]. However, increased sensitivity can result in false-positive results because of insufficient technical performance or positive results from colonization in addition to infection [317]. These drawbacks for PCP diagnosis can be overcome using quantitative real-time PCR. The closed system of real-time PCR prevents contamination with amplicons, which cause false-positive results. Furthermore, quantification can be used to distinguish true infection from colonization, although an indeterminate zone would remain [314]. In this study, the RealStar assay and the AmpliSens assay demonstrated similar performance. The results of the two assays showed a strong correlation, although the Ct values of the AmpliSens assay could not be used for quantification.
The four samples exhibiting discordant results were positive only in the RealStar assay because of low P. jirovecii burden (<500 copies/mL). This may be explained by differences in data interpretation; a positive test result is defined as a threshold ≤35 for the AmpliSens assay, whereas any amplification in the RealStar assay is considered a positive test result.
The finding that P. jirovecii DNA load was significantly higher in the clinical PCP group than in the other groups shows that real-time PCR can be helpful in discriminating active PCP from colonization. Our data confirm previous findings of a lower fungal burden in colonized patients than in PCP patients [5817181920]. In this context, DNA copy number or Ct values have been suggested as cut-off values of fungal burden for differentiating PCP from colonization [4691721]. However, we did not report cut-off values as the number of patients with clinical PCP was low, and the DNA load distribution of the two assays ranged widely. Our study population comprised non-HIV immunocompromised patients, which could explain the low discrimination level between PCP and other groups compared with studies in HIV-positive patients [4621].
BAL fluid samples provide a good yield for the detection of fungal DNA and are the standard for PCP diagnosis, as P. jirovecii resides mainly in the alveolar space [1]. A gradient of fungal burden is expected between the highest load in BAL fluid samples and the lowest load in upper respiratory tract (URT) samples [3]. Indeed, a few studies have observed higher fungal detection rates in BAL fluids than in URT samples [222324]. However, information regarding the yield obtained from different LRT sample types is limited, particularly in non-HIV immunocompromised patients [2526]. Alanio et al. [26] reported that fungal burdens did not significantly differ between BAL fluids and induced sputum; however, we demonstrated low detection rates from sputum/ETA samples compared with BAL samples. As we used expectorated sputa from non-HIV patients, this difference may be explained by a low DNA burden in sputum/ETA samples. A false-negative result in a sputum/ETA sample can cause PCP diagnosis to be missed, especially in non-HIV immunocompromised patients.
Unlike the RealStar assay, in which no invalid results were observed, the AmpliSens assay gave 14 invalid results. This may be due to differences in the internal controls used in each assay. The RealStar assay uses an artificial heterologous extrinsic control, whereas the AmpliSens assay uses the human β-globin gene as a heterologous intrinsic control to monitor PCR inhibition. As heterologous intrinsic controls monitor the presence of cells and human DNA in a sample, it is possible to assess sample quality as well as nucleic acid extraction and the amplification process [27]. Therefore, these invalid results suggest that the respiratory samples were of inadequate quality.
The present study had some limitations. First, as the samples were obtained from consecutive patients, the number of clinical PCP patients included was low. Second, we could not compare the performance of the two assays with IF or β-D-glucan assays.
In conclusion, the RealStar and AmpliSens assays showed equivalent diagnostic performance. They had high agreement with each other and enabled detection of a low P. jirovecii burden in BAL fluid samples. Thus, both assays may be useful as routine methods for detecting P. jirovecii DNA in a clinical laboratory setting. Nevertheless, low-level PCR positive results should be interpreted while considering clinical context and type or quality of the sample.
References
1. Thomas CF Jr, Limper AH. Pneumocystis pneumonia. N Engl J Med. 2004; 350:2487–2498. PMID: 15190141.
2. White PL, Backx M, Barnes RA. Diagnosis and management of Pneumocystis jirovecii infection. Expert Rev Anti Infect Ther. 2017; 15:435–447. PMID: 28287010.
3. Alanio A, Hauser PM, Lagrou K, Melchers WJ, Helweg-Larsen J, Matos O, et al. ECIL guidelines for the diagnosis of Pneumocystis jirovecii pneumonia in patients with haematological malignancies and stem cell transplant recipients. J Antimicrob Chemother. 2016; 71:2386–2396. PMID: 27550991.
4. Fauchier T, Hasseine L, Gari-Toussaint M, Casanova V, Marty PM, Pomares C. Detection of Pneumocystis jirovecii by quantitative PCR to differentiate colonization and pneumonia in immunocompromised HIV-positive and HIV-negative patients. J Clin Microbiol. 2016; 54:1487–1495. PMID: 27008872.
5. Robert-Gangneux F, Belaz S, Revest M, Tattevin P, Jouneau S, Decaux O, et al. Diagnosis of Pneumocystis jirovecii pneumonia in immunocompromised patients by real-time PCR: a 4-year prospective study. J Clin Microbiol. 2014; 52:3370–3376. PMID: 25009050.
6. Montesinos I, Brancart F, Schepers K, Jacobs F, Denis O, Delforge ML. Comparison of 2 real-time PCR assays for diagnosis of Pneumocystis jirovecii pneumonia in human immunodeficiency virus (HIV) and non-HIV immunocompromised patients. Diagn Microbiol Infect Dis. 2015; 82:143–147. PMID: 25801778.
7. Botterel F, Cabaret O, Foulet F, Cordonnier C, Costa JM, Bretagne S. Clinical significance of quantifying Pneumocystis jirovecii DNA by using real-time PCR in bronchoalveolar lavage fluid from immunocompromised patients. J Clin Microbiol. 2012; 50:227–231. PMID: 22162560.
8. Sasso M, Chastang-Dumas E, Bastide S, Alonso S, Lechiche C, Bourgeois N, et al. Performances of four real-time PCR assays for diagnosis of Pneumocystis jirovecii pneumonia. J Clin Microbiol. 2016; 54:625–630. PMID: 26719435.
9. Montesinos I, Delforge ML, Ajjaham F, Brancart F, Hites M, Jacobs F, et al. Evaluation of a new commercial real-time PCR assay for diagnosis of Pneumocystis jirovecii pneumonia and identification of dihydropteroate synthase (DHPS) mutations. Diagn Microbiol Infect Dis. 2017; 87:32–36. PMID: 27789058.
10. Guillaud-Saumur T, Nevez G, Bazire A, Virmaux M, Papon N, Le Gal S. Comparison of a commercial real-time PCR assay, RealCycler® PJIR kit, progenie molecular, to an in-house real-time PCR assay for the diagnosis of Pneumocystis jirovecii infections. Diagn Microbiol Infect Dis. 2017; 87:335–337. PMID: 28143789.
11. McTaggart LR, Wengenack NL, Richardson SE. Validation of the MycAssay Pneumocystis kit for detection of Pneumocystis jirovecii in bronchoalveolar lavage specimens by comparison to a laboratory standard of direct immunofluorescence microscopy, real-time PCR, or conventional PCR. J Clin Microbiol. 2012; 50:1856–1859. PMID: 22422855.
12. Altona Diagnostics. Introductions for use: RealStar Pneumocystis jirovecii PCR Kit 1.0, 09/2016 [package insert]. Altona Diagnostics;2016.
13. Tia T, Putaporntip C, Kosuwin R, Kongpolprom N, Kawkitinarong K, Jongwutiwes S. A highly sensitive novel PCR assay for detection of Pneumocystis jirovecii DNA in bronchoalveloar lavage specimens from immunocompromised patients. Clin Microbiol Infect. 2012; 18:598–603. PMID: 21951463.
14. Cooley L, Dendle C, Wolf J, Teh BW, Chen SC, Boutlis C, et al. Consensus guidelines for diagnosis, prophylaxis and management of Pneumocystis jirovecii pneumonia in patients with haematological and solid malignancies, 2014. Intern Med J. 2014; 44:1350–1363. PMID: 25482745.
15. Lu Y, Ling G, Qiang C, Ming Q, Wu C, Wang K, et al. PCR diagnosis of Pneumocystis pneumonia: a bivariate meta-analysis. J Clin Microbiol. 2011; 49:4361–4363. PMID: 22012008.
16. Fan LC, Lu HW, Cheng KB, Li HP, Xu JF. Evaluation of PCR in bronchoalveolar lavage fluid for diagnosis of Pneumocystis jirovecii pneumonia: a bivariate meta-analysis and systematic review. PLoS One. 2013; 8:e73099. PMID: 24023814.
17. Mühlethaler K, Bögli-Stuber K, Wasmer S, von Garnier C, Dumont P, Rauch A, et al. Quantitative PCR to diagnose Pneumocystis pneumonia in immunocompromised non-HIV patients. Eur Respir J. 2012; 39:971–978. PMID: 21920890.
18. Hauser PM, Bille J, Lass-Flörl C, Geltner C, Feldmesser M, Levi M, et al. Multicenter, prospective clinical evaluation of respiratory samples from subjects at risk for Pneumocystis jirovecii infection by use of a commercial real-time PCR assay. J Clin Microbiol. 2011; 49:1872–1878. PMID: 21367988.
19. Maillet M, Maubon D, Brion JP, François P, Molina L, Stahl JP, et al. Pneumocystis jirovecii (Pj) quantitative PCR to differentiate Pj pneumonia from Pj colonization in immunocompromised patients. Eur J Clin Microbiol Infect Dis. 2014; 33:331–336. PMID: 23990137.
20. Rudramurthy SM, Sharma M, Sharma M, Rawat P, Ghosh A, Venkatesan L, et al. Reliable differentiation of Pneumocystis pneumonia from Pneumocystis colonisation by quantification of Major Surface Glycoprotein gene using real-time polymerase chain reaction. Mycoses. 2018; 61:96–103. PMID: 28945326.
21. Louis M, Guitard J, Jodar M, Ancelle T, Magne D, Lascols O, et al. Impact of HIV infection status on interpretation of quantitative PCR for detection of Pneumocystis jirovecii. J Clin Microbiol. 2015; 53:3870–3875. PMID: 26468505.
22. Matos O, Costa MC, Lundgren B, Caldeira L, Aguiar P, Antunes F. Effect of oral washes on the diagnosis of Pneumocystis carinii pneumonia with a low parasite burden and on detection of organisms in subclinical infections. Eur J Clin Microbiol Infect Dis. 2001; 20:573–575. PMID: 11681438.
23. Samuel CM, Whitelaw A, Corcoran C, Morrow B, Hsiao NY, Zampoli M, et al. Improved detection of Pneumocystis jirovecii in upper and lower respiratory tract specimens from children with suspected pneumocystis pneumonia using real-time PCR: a prospective study. BMC Infect Dis. 2011; 11:329. PMID: 22123076.
24. Helweg-Larsen J, Jensen JS, Benfield T, Svendsen UG, Lundgren JD, Lundgren B. Diagnostic use of PCR for detection of Pneumocystis carinii in oral wash samples. J Clin Microbiol. 1998; 36:2068–2072. PMID: 9650964.
25. Chien JY, Liu CJ, Chuang PC, Lee TF, Huang YT, Liao CH, et al. Evaluation of the automated Becton Dickinson MAX real-time PCR platform for detection of Pneumocystis jirovecii. Future Microbiol. 2017; 12:29–37. PMID: 27936923.
26. Alanio A, Desoubeaux G, Sarfati C, Hamane S, Bergeron A, Azoulay E, et al. Real-time PCR assay-based strategy for differentiation between active Pneumocystis jirovecii pneumonia and colonization in immunocompromised patients. Clin Microbiol Infect. 2011; 17:1531–1537. PMID: 20946413.
27. Nummi M, Mannonen L, Puolakkainen M. Development of a multiplex real-time PCR assay for detection of Mycoplasma pneumoniae, Chlamydia pneumoniae and mutations associated with macrolide resistance in Mycoplasma pneumoniae from respiratory clinical specimens. Springerplus. 2015; 4:684. PMID: 26576327.
Fig. 1
Scatter plot showing a strong correlation between the quantitative results (log copies/mL) obtained using the RealStar assay and the Ct values obtained using the AmpliSens assay (r=−0.94; 95% confidence interval, −0.97 to −0.88).
Abbreviation: Ct, Cycle threshold.
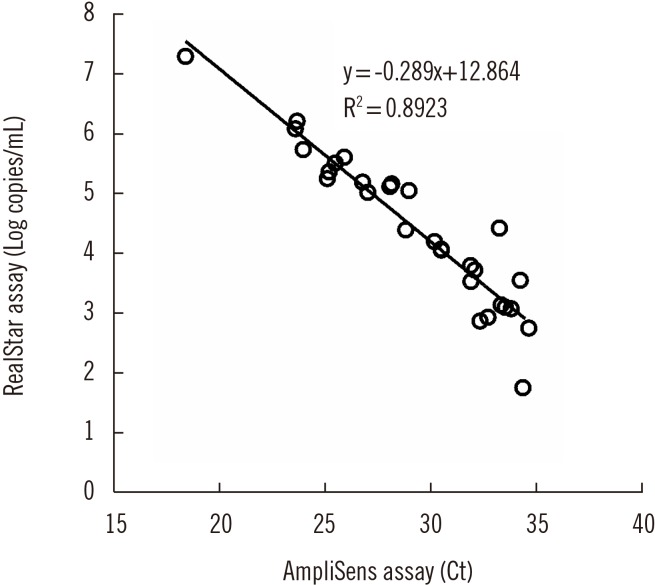
Fig. 2
Distribution of P. jirovecii DNA load according to clinical probability of PCP. (A) Distribution of quantitative results (log copies/mL) obtained using the RealStar assay in total samples; (B) Distribution of the Ct values obtained using the AmpliSens assay in total samples; (C) Distribution of quantitative results (log copies/mL) obtained by the RealStar assay in BAL samples; (D) Distribution of Ct values obtained by the AmpliSens assay in BAL samples. Median log copies/mL or Ct values and IQRs are indicated (central horizontal lines, median; outer horizontal lines, 25–95% IQR).
Abbreviations: Ct, Cycle threshold; BAL, bronchoalveolar lavage; IQR, interquartile range; PCP, Pneumocystis pneumonia.
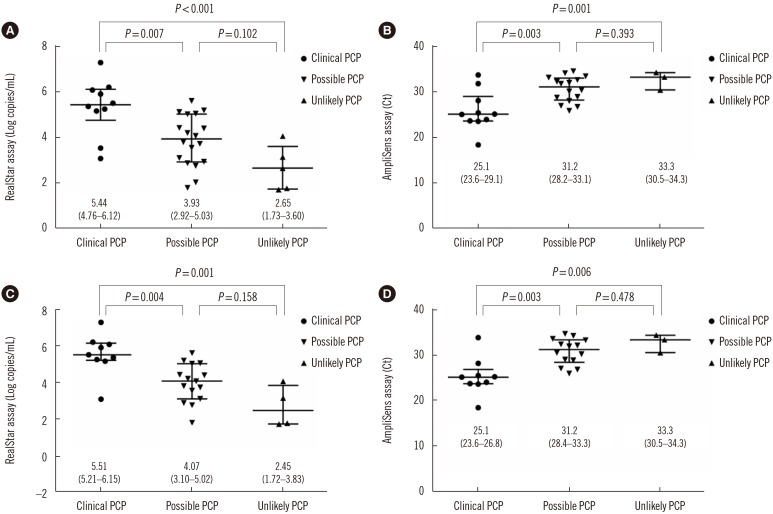
Table 1
Comparison of two real-time PCR assays for detecting Pneumocystis jirovecii DNA
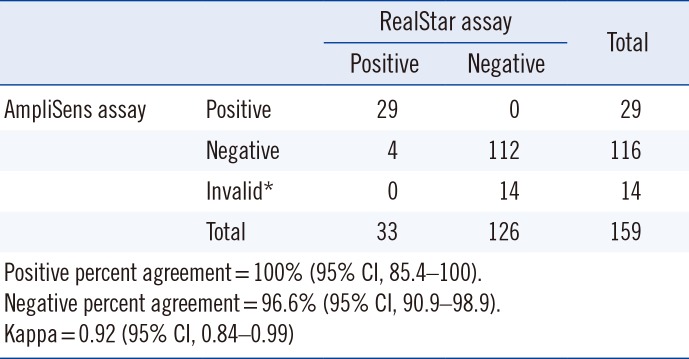
Table 2
Results of two real-time PCR assays according to patient classification and sample type
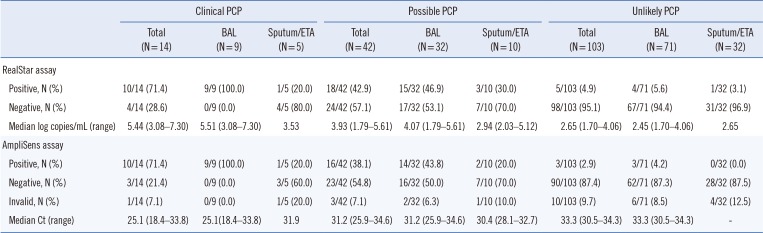