Abstract
Objective
Bone flap resorption (BFR) is a complication of cranioplasty (CP) that increases the risk of brain damage and can cause cosmetic defects. In this study, the risk factors for BFR were examined to improve the prognosis of patients after CP for traumatic brain injury (TBI).
Methods
This study was conducted in 80 patients with TBI who underwent decompressive craniectomy and CP with an autologous bone graft between August 2006 and August 2017. BFR was defined as a >0.1 ratio of the difference between the initial bone flap area and the last bone flap area to the craniectomy size and a <0.5 ratio of the last bone flap thickness to the bone thickness of the contralateral region on computed tomography scans and plain skull radiographs. The patients were divided into the BFR and non-BFR groups, and medical data were compared between the two groups.
Results
Among the 80 patients, 22 (27.5%) were diagnosed as having BFR after CP. The earliest cases of BFR occurred at 57 days after CP, and the latest BFR cases occurred at 3,677 days after CP. Using multivariate logistic regression analyses, the initial dead space size (odds ratio [OR], 1.002; 95% confidence interval [CI], 1.001–1.004; p=0.006) and multiplicity of the bone flap (OR, 3.058; 95% CI, 1.021–9.164; p=0.046) were found to be risk factors for BFR.
Cranioplasty (CP) is performed for restoration of the cosmetic, protective, and hydrodynamic conditions of the skull after decompressive craniectomy (DC) for traumatic brain injury (TBI).293031) CP is known to be associated with a high incidence of postoperative complications (e.g., epidural fluid collection, surgical site infection, intracerebral hemorrhage, epidural hematoma, wound dehiscence, hydrocephalus, seizure, and bone flap resorption [BFR]).357101526) Among these, BFR increases the risk of severe brain damage and may cause undesirable cosmetic defects. Furthermore, it results in patient distress and adds to the treatment cost because of further hospitalization and re-CP.
CP can be performed with an autologous or alloplastic bone graft. Recently, various alloplastic materials have been developed owing to advances in medical technology. In addition to polymethyl methacrylate, titanium mesh, and polyetheretherketone obtained by 3-dimensional printing are also now being used frequently.2027) Nevertheless, an autologous bone flap is still used in most cases owing to its low cost, perfect match to the bony defect, and convenient surgical handling.2730)
The purpose of this study was to identify the risk factors for BFR to reduce the risk of brain damage caused by this complication, reduce cosmetic defects, and improve prognosis.
The study was reviewed and approved by the Institutional Review Board (approval no. 2018-07-009).
This study was conducted in 156 patients who underwent 1) DC because of TBI (resulting from epidural hemorrhage, subdural hemorrhage, subarachnoid hemorrhage, intracerebral hemorrhage, hemorrhagic contusion, intraventricular hemorrhage, brain swelling, fracture compound comminuted depressed, etc.); and 2) CP between August 2006 and August 2017 at the Department of Neurosurgery, Hallym University Kangnam Sacred Heart Hospital, Hallym University College of Medicine. The exclusion criteria were CP performed with an artificial bone flap, death, radiological follow-up loss, bone flap removal because of complications after CP, and additional CP performed at other hospitals. On the basis of the exclusion criteria, 76 patients were excluded from the study and 80 patients were finally included (Figure 1).
Medical data including age, sex, presence of hypertension, presence of diabetes mellitus, initial diagnosis before DC, multiplicity of bone flap (because of facture or additional bone flap incision during operation), craniectomy size, initial bone flap area, initial dead space size (craniectomy size - initial bone flap area), CP operative time, presence or absence of a ventriculo-peritoneal shunt/lumboperitoneal shunt, the interval between DC and CP, and other complications were analyzed through a retrospective review.
BFR was defined as a >0.1 ratio of the difference between the initial bone flap area and the last bone flap area to the craniectomy size18) (Figure 2) and a <0.5 ratio of the last bone flap thickness to the bone thickness of the contralateral region on computed tomography (CT) scans or plain skull radiographs (Figure 3).14) As all radiographs were digitized using a picture archiving and communication system (PiViewStar 5.0; Infinitt, Seoul, Korea) at our hospital, all areas and diameters were measured using the calipers and free region of interest provided by the software. Measurements of the initial bone flap area and craniectomy size were obtained through plain skull radiography immediately after CP, and those of the last bone flap area and bone flap thickness were obtained using the radiographs and CT scans obtained at the last follow-up. Follow-up was scheduled at our outpatient department, according to an institutional protocol, with plain skull radiography and CT scans at 6 weeks, 3 months, 6 months, 12 months, and yearly thereafter.
During DC, the bone flap was collected, immediately followed by removal of connective tissue; irrigation was done using sterile H2O2 and 9% normal saline (9 mg/mL). The bone flap was covered with sterile gauze and pad and placed inside a 2-layer sterile instrument pouch. Subsequently, the cleaned bone flap was aseptically stored in a medical ultra-low freezer (Revco Ultra-Low Upright Ultima II ULT2586-9D; Thermo Fisher, Asheville, NC, USA) at −86℃.
The bone flap was removed from the freezer and placed in sterile povidone-iodine solution. The previous incision was made near the scalp, and the temporal muscle was carefully dissected while avoiding injury to the dura mater. If dissection was difficult because of strong adhesion between the temporal muscle and dura mater, the bone flap was placed over the temporal muscle. The bone flap and skull margin at the craniectomy site were accurately closed using a drill and diamond burs. Subsequently, the bone flap was fixed at the cranium using Craniofix® (B Braun Aesculap, Tuttlingen, Germany) and cranio-maxillofacial surgery instrument kit (TPO-006; Osteonic Co., Ltd., Seoul, Korea). Epidural and subcutaneous drains were inserted, and antibiotics were intravenously administered for 7 days after CP.
Data were analyzed using SPSS version 21.0 software for Windows (IBM Corp., Armonk, NY, USA). All statistical analyses were 2-tailed, and a p<0.05 was considered statistically significant. Discrete variables are expressed as total number and percentage, and continuous variables as mean±standard deviation for normal distribution or median and interquartile range for non-normal distribution, unless stated otherwise. Categorical data were compared using the χ2 test or Fisher's exact test, as appropriate. Continuous variables conforming to a normal distribution were compared using Student's t-test; otherwise, the Mann-Whitney U-test was used. The risk factors for BFR that were statistically significant in the univariate model in our study and those identified in other BFR studies were included in the multivariate analysis; however, these were limited to 5 variables owing to the relatively low number of patients in our study.
The mean age of the patients was 47.6±15.5 years (range, 8–73 years); 43 patients were men and 37 were women. Among them, 21 patients (26.3%) had hypertension and 6 patients (7.5%) had diabetes mellitus. DC was performed for the following reasons: subdural hemorrhage in 44 patients (55%), epidural hemorrhage in 5 patients (6.3%), intracerebral hemorrhage in 25 patients (31.3%), hemorrhagic contusion in 5 patients (6.3%), and cerebral swelling in 1 patient (1.3%). Overall, the median time to replacement between DC and CP was 118 days (interquartile range, 81–191 days). The mean size of the craniectomy site was 7,834±2,323 mm2. The mean size of the initial bone flap area was 7,085±2,177 mm2 and that of the last bone flap area was 6,453±2,236 mm2. The average size of the initial dead space was 748±338 mm2. There were 48 patients (60%) with 1 fragment and 32 patients (40%) with 2 or more fragments. The median operative time during CP was 147 min (interquartile range, 108–173 min). There were 17 patients (21.3%) with shunt-dependent hydrocephalus. Complications occurred in 8 patients, including infections in 4 patients (5%), displacement in 2 patients (2.5%), and epidural hemorrhage in 2 patients (2.5%).
Among the 80 patients, 22 (27.5%) were diagnosed as having BFR after CP. The earliest cases occurred at 57 days and the latest cases, at 3,677 days after CP.
Among the categorical variables, multiplicity of the bone flap (p=0.032) was determined to be a risk factor for BFR. Hypertension was a borderline significant (p=0.066) factor (Table 1). In the case of complications, the number of samples for each independent variable was too small to be statistically significant. The rate of BFR was not affected by the initial diagnosis (p=0.956) and the presence of diabetes mellitus (p=0.338).
All continuous variables were statistically analyzed using the Mann-Whitney U-test, and the size of the initial dead space (p=0.019) was found to be a significant risk factor. The craniectomy size (p=0.447), CP operative time (p=0.229), and age (p=0.415), which previous studies suggested as risk factors for BFR, were not found to be significant in this study (Table 2).
We performed univariate and multivariate logistic regression analyses. On multivariate logistic regression analysis, 2 parameters were identified as predictive factors for BFR: multiplicity of the bone flap (odds ratio [OR], 3.058; 95% confidence interval [CI], 1.021–9.164; p=0.046) and initial dead space size (OR, 1.002; 95% CI, 1.001–1.004; p=0.006) (Table 3).
In this study, we reexamined the issue of BFR and analyzed the potential risk factors that can highly contribute to CP failure. Among the various risk factors identified in previous BFR studies, young age was the most frequently reported. The authors specifically assessed the effect of patient age on the risk of complications in 6 of the studies. 3617181925) Bowers et al.3) speculated that the extremely rapid period of head growth, with its accompanying increased metabolic demand on the calvaria in children (age ≤2.5 years), may result in insufficient time for extremely large bone flaps to create a fusion. In our study, there was no correlation between age and BFR; however, Gosain et al.8) demonstrated in an animal experiment that younger subjects have improved osteogenic capabilities with concurrent lower resorption rates.
Whether the presence of shunt-dependent hydrocephalus is a risk factor for BFR is controversial. Dünisch et al.6) and Bowers et al.3) suspected that the burr hole leads to variation in the cranial pressure, resulting in incomplete adherence between the dura mater and the bone flap, thus disturbing skull growth. However, in several studies including ours, the presence of shunt-dependent hydrocephalus was not a significant risk factor.
The time interval between craniectomy and CP is usually 6 weeks to 1 year.2121923) Several studies on adults have evaluated the relationship of the time interval between craniectomy and CP with the risk of BFR. Schuss et al.24) showed that early CP (<2 months) increases the risk of BFR. In a study on delayed CP, Iwama et al.11) demonstrated, using histological studies of cryopreservation, that osteocyte activity continued in the bone flaps up to 3 years after cryopreservation. Conversely, some studies reported that patients with a long interval (>3 months) between DC and CP manifested symptoms of large cranial defects and sunken scalps.5) In this regard, like our study, the studies by Dünisch et al.6) and Park et al.18) failed to demonstrate a statistical correlation between the timing of CP and BFR.
In terms of anatomical approaches, previous BFR studies have focused on craniectomy size,1723) and our study investigated dead space as an additional risk factor, taking into account the relationship between the craniectomy site and the self-bone flap. According to the mechanism related to the size of the dead space, the survival of a bone implantation graft depends on the reaction of the surrounding tissue and on functional contact between cancellous bone and adjacent resident bone.21) The most critical period of bone healing is the first 2 weeks. During the first week after grafting, capillaries from the surrounding bone, dura, and scalp infiltrate the transplant bed. During the second week, fibrous granulation tissue proliferates and osteoplastic activity occurs.13) In the case of a huge dead space between the craniectomy site and the implanted bone flap, vascularization and osteoprogenitor cell migration are difficult.1142122) Eventually, the limitation of surface bone growth causes BFR. Multiplicity of the bone flap as a result of fracture or a surgical procedure showed a clear correlation with the risk of BFR. Osteoinduction and osteoconduction procedures that are unnecessary in a single bone flap additionally occurred for bone healing. Fractures interfere with blood circulation and angiogenesis, which, in turn, interfere with bone formation.46925) Various types of bone growth factors are necessary for bone formation, including insulin-like growth factor (I, II), fibroblast growth factor, transforming growth factor-β, and platelet-derived growth factor.1) We believe that the growth factors have a limited availability, whereas multiplicity of bone flaps results in an additional requirement for growth factors; therefore, bone formation is not properly achieved, resulting in BFR.
In particular, during the first week of bone healing, steroid medications, cytotoxic agents, and nonsteroidal anti-inflammatory medications can have harmful effects. Irradiation of the fusion site within the first 2 to 3 weeks can inhibit cell proliferation and induce acute vasculitis that considerably compromises bone healing.13)
In previous studies, the prevalence of BFR was reported to be 1.4% to 50%.69162328) There is a huge difference in the prevalence reported in different studies, and the first reason is that BFR is defined differently by each study. Dünisch et al.6) and Schoekler and Trummer23) defined craniectomy size as an ellipse with the formula π×a×b (longest axis being 2a and the 90° short axis being 2b). To improve the structural accuracy of BFR, our study was based on 2 parameters. The second reason for the difference is the variable patient settings among studies. In most studies, patients of all age groups were included, with a predominance of adults; in other studies, patients younger than 20 years were included. In addition, the prevalence of BFR was low in some studies, as they included not only patients with autologous bones but also those with artificial bones.
References
1. Albrektsson T, Johansson C. Osteoinduction, osteoconduction and osseointegration. Eur Spine J. 2001; 10(Suppl 2):S96–S101. PMID: 11716023.


2. Baumeister S, Peek A, Friedman A, Levin LS, Marcus JR. Management of postneurosurgical bone flap loss caused by infection. Plast Reconstr Surg. 2008; 122:195e–208e.


3. Bowers CA, Riva-Cambrin J, Hertzler DA 2nd, Walker ML. Risk factors and rates of bone flap resorption in pediatric patients after decompressive craniectomy for traumatic brain injury. J Neurosurg Pediatr. 2013; 11:526–532. PMID: 23473303.


4. Brommeland T, Rydning PN, Pripp AH, Helseth E. Cranioplasty complications and risk factors associated with bone flap resorption. Scand J Trauma Resusc Emerg Med. 2015; 23:75. PMID: 26437934.


5. Chang V, Hartzfeld P, Langlois M, Mahmood A, Seyfried D. Outcomes of cranial repair after craniectomy. J Neurosurg. 2010; 112:1120–1124. PMID: 19612971.


6. Dünisch P, Walter J, Sakr Y, Kalff R, Waschke A, Ewald C. Risk factors of aseptic bone resorption: a study after autologous bone flap reinsertion due to decompressive craniotomy. J Neurosurg. 2013; 118:1141–1147. PMID: 23451904.


7. Gooch MR, Gin GE, Kenning TJ, German JW. Complications of cranioplasty following decompressive craniectomy: analysis of 62 cases. Neurosurg Focus. 2009; 26:E9.


8. Gosain AK, Gosain SA, Sweeney WM, Song LS, Amarante MT. Regulation of osteogenesis and survival within bone grafts to the calvaria: the effect of the dura versus the pericranium. Plast Reconstr Surg. 2011; 128:85–94. PMID: 21399563.


9. Grant GA, Jolley M, Ellenbogen RG, Roberts TS, Gruss JR, Loeser JD. Failure of autologous bone-assisted cranioplasty following decompressive craniectomy in children and adolescents. J Neurosurg. 2004; 100:163–168. PMID: 14758944.


10. Honeybul S, Ho KM. Long-term complications of decompressive craniectomy for head injury. J Neurotrauma. 2011; 28:929–935. PMID: 21091342.


11. Iwama T, Yamada J, Imai S, Shinoda J, Funakoshi T, Sakai N. The use of frozen autogenous bone flaps in delayed cranioplasty revisited. Neurosurgery. 2003; 52:591–596. PMID: 12590683.


12. Jho DH, Neckrysh S, Hardman J, Charbel FT, Amin-Hanjani S. Ethylene oxide gas sterilization: a simple technique for storing explanted skull bone. Technical note. J Neurosurg. 2007; 107:440–445. PMID: 17695404.
14. Kim JS, Cheong JH, Ryu JI, Kim JM, Kim CH. Bone flap resorption following cranioplasty after decompressive craniectomy: Preliminary report. Korean J Neurotrauma. 2015; 11:1–5. PMID: 27169057.


15. Kim SP, Kang DS, Cheong JH, Kim JH, Song KY, Kong MH. Clinical analysis of epidural fluid collection as a complication after cranioplasty. J Korean Neurosurg Soc. 2014; 56:410–418. PMID: 25535519.


16. Klinger DR, Madden C, Beshay J, White J, Gambrell K, Rickert K. Autologous and acrylic cranioplasty: a review of 10 years and 258 cases. World Neurosurg. 2014; 82:e525–e530. PMID: 24036124.


17. Martin KD, Franz B, Kirsch M, Polanski W, von der Hagen M, Schackert G, et al. Autologous bone flap cranioplasty following decompressive craniectomy is combined with a high complication rate in pediatric traumatic brain injury patients. Acta Neurochir (Wien). 2014; 156:813–824. PMID: 24532225.


18. Park SP, Kim JH, Kang HI, Kim DR, Moon BG, Kim JS. Bone flap resorption following cranioplasty with autologous bone: Quantitative measurement of bone flap resorption and predictive factors. J Korean Neurosurg Soc. 2017; 60:749–754. PMID: 29142636.
19. Piedra MP, Thompson EM, Selden NR, Ragel BT, Guillaume DJ. Optimal timing of autologous cranioplasty after decompressive craniectomy in children. J Neurosurg Pediatr. 2012; 10:268–272. PMID: 22861195.


20. Piitulainen JM, Kauko T, Aitasalo KM, Vuorinen V, Vallittu PK, Posti JP. Outcomes of cranioplasty with synthetic materials and autologous bone grafts. World Neurosurg. 2015; 83:708–714. PMID: 25681593.


21. Redfern RM, Pülhorn H. Cranioplasty. Adv Clin Neurosci Rehabil. 2007; 7:32–34.
22. Sanan A, Haines SJ. Repairing holes in the head: a history of cranioplasty. Neurosurgery. 1997; 40:588–603. PMID: 9055300.


23. Schoekler B, Trummer M. Prediction parameters of bone flap resorption following cranioplasty with autologous bone. Clin Neurol Neurosurg. 2014; 120:64–67. PMID: 24731578.


24. Schuss P, Vatter H, Marquardt G, Imöhl L, Ulrich CT, Seifert V, et al. Cranioplasty after decompressive craniectomy: the effect of timing on postoperative complications. J Neurotrauma. 2012; 29:1090–1095. PMID: 22201297.


25. Schwarz F, Dunisch P, Walter J, Sakr Y, Kalff R, Ewald C. Cranioplasty after decompressive craniectomy: is there a rationale for an initial artificial bone-substitute implant? A single-center experience after 631 procedures. J Neurosurg. 2016; 124:710–715. PMID: 26406796.


26. Shaffrey ME, Persing JA, Shaffrey CI. Craniofacial reconstruction. In : Apuzzo MLJ, editor. Brain surgery: Complication avoidance and management. New York, NY: Churchill Livingstone;1993. p. 1373–1398.
27. Shah AM, Jung H, Skirboll S. Materials used in cranioplasty: a history and analysis. Neurosurg Focus. 2014; 36:E19. PMID: 24684331.


28. Wachter D, Reineke K, Behm T, Rohde V. Cranioplasty after decompressive hemicraniectomy: underestimated surgery-associated complications? Clin Neurol Neurosurg. 2013; 115:1293–1297. PMID: 23273384.


29. Winkler PA, Stummer W, Linke R, Krishnan KG, Tatsch K. Influence of cranioplasty on postural blood flow regulation, cerebrovascular reserve capacity, and cerebral glucose metabolism. J Neurosurg. 2000; 93:53–61.


30. Won YD, Yoo DS, Kim KT, Kang SG, Lee SB, Kim DS, et al. Cranioplasty effect on the cerebral hemodynamics and cardiac function. Acta Neurochir Suppl. 2008; 102:15–20. PMID: 19388280.


31. Zanaty M, Chalouhi N, Starke RM, Clark SW, Bovenzi CD, Saigh M, et al. Complications following cranioplasty: incidence and predictors in 348 cases. J Neurosurg. 2015; 123:182–188. PMID: 25768830.


FIGURE 1
Flowchart for the inclusion of patients in this retrospective study. CP: cranioplasty, f/u: follow-up.
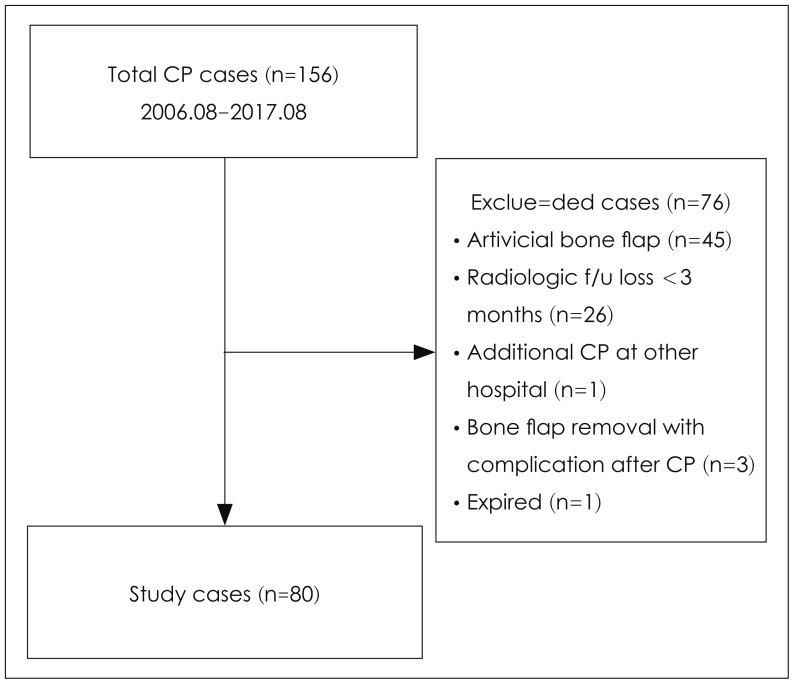
FIGURE 2
Plain skull radiographs in anteroposterior and lateral views. (A) Immediately after the operation; the yellow border indicates the initial bone flap area, the white border indicates the craniectomy area, and the red area indicates the initial dead space. (B) Two years after cranioplasty; the yellow border indicates the last bone flap area, which shows severe bone flap resorption. The areas were measured using free region of interest.
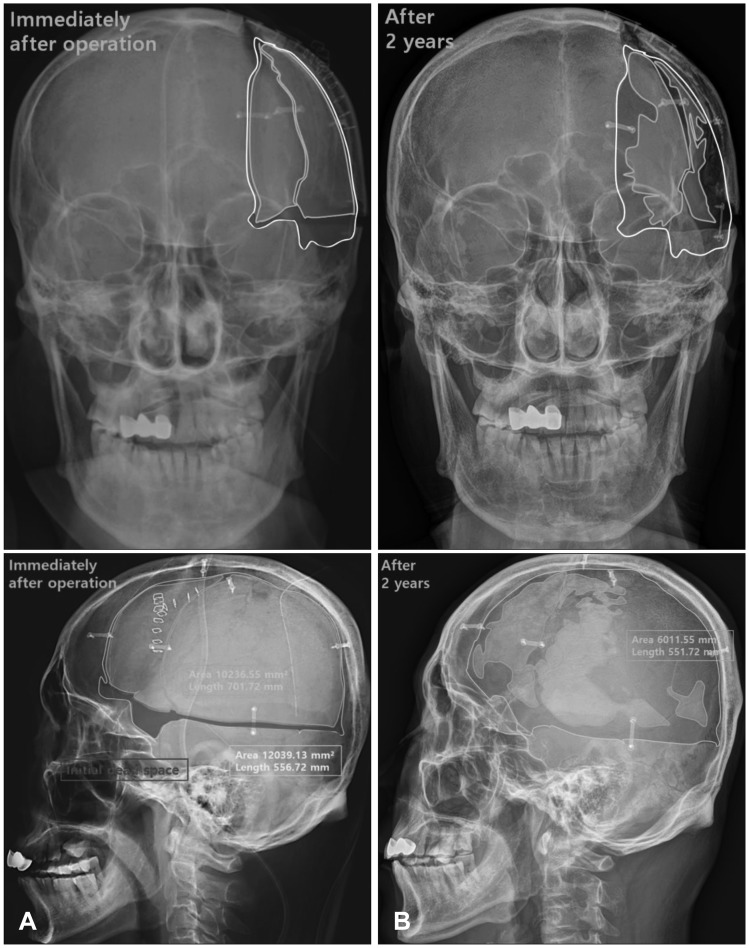
FIGURE 3
Computed tomography image of bone flap resorption at 2 years after cranioplasty, showing partial resorption of the bone flap, in which the remnant bone flap (thickness, 1.1 mm) was <50% as thick as the contralateral region (thickness, 6.1 mm) of the skull.
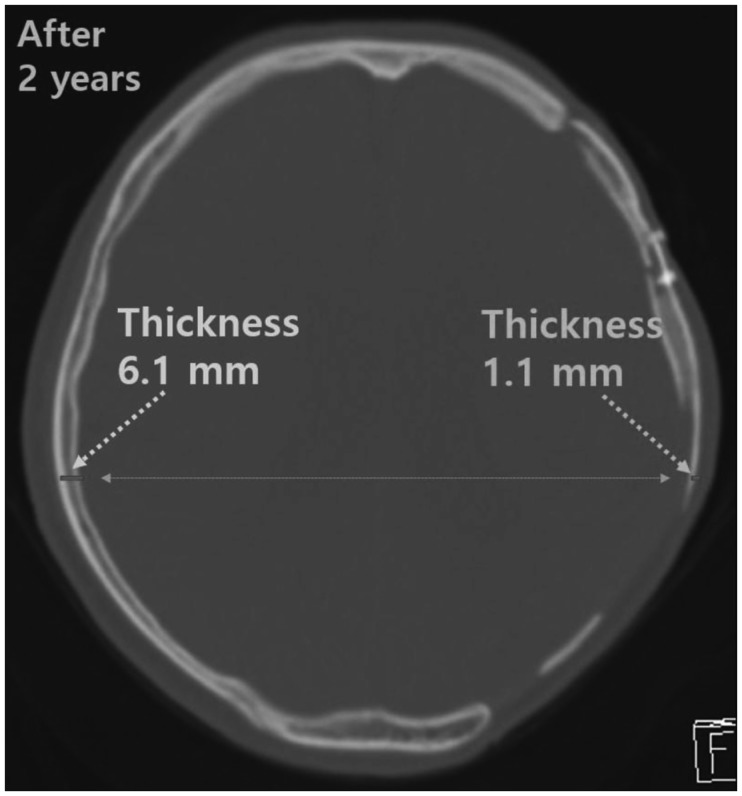
TABLE 1
Univariate analysis of categorical variables between the bone flap resorption and non-bone flap resorption groups
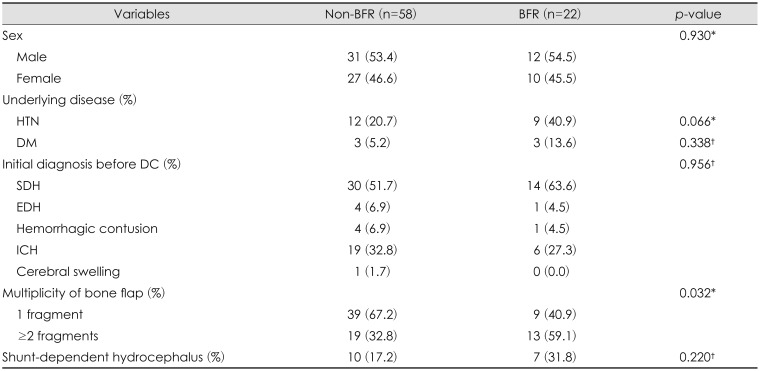