Abstract
In the present study, we investigated whether tussilagone, a natural product derived from Tussilago farfara, significantly affects the production and gene expression of airway MUC5AC mucin. Confluent NCI-H292 cells were pretreated with tussilagone for 30 min and then stimulated with EGF (epidermal growth factor) or PMA (phorbol 12-myristate 13-acetate) for 24 h or the indicated periods. The MUC5AC mucin gene expression was measured by RT-PCR. Production of MUC5AC mucin protein was measured by ELISA. To elucidate the action mechanism of tussilagone, effect of tussilagone on PMA-induced NF-κB signaling pathway was investigated by western blot analysis. Tussilagone significantly inhibited the production of MUC5AC mucin protein and down-regulated the expression of MUC5AC mucin gene, induced by EGF or PMA. Tussilagone inhibited PMA-induced activation (phosphorylation) of inhibitory kappa B kinase (IKK), and thus phosphorylation and degradation of inhibitory kappa Ba (IκBα). Tussilagone inhibited PMA-induced phosphorylation and nuclear translocation of nuclear factor kappa B (NF-κB) p65. This, in turn, led to the down-regulation of MUC5AC protein production in NCI-H292 cells. These results suggest that tussilagone can regulate the production and gene expression of mucin by acting on airway epithelial cells through regulation of NF-κB signaling pathway.
Mucins are the major glycoproteins present in pulmonary mucus and produced by goblet cells in the surface epithelium as well as mucous cells in the submucosal gland. Pulmonary mucus containing mucins is very important in defensive action of human body against invading pathogenic microorganisms, diverse particles and irritating chemicals. The protective function of pulmonary mucus is attributed to the viscoelasticity, a specific physicochemical property, of mucins. However, any abnormality in the quality or quantity of mucins is a common pathological feature in chronic obstructive pulmonary disease (COPD), asthma, cystic fibrosis (CF), and lung cancer [1]. Therefore, we suggest it is valuable to find the possible activity of controlling (inhibiting) the excessive mucin production by the compounds derived from various medicinal plants. We have tried to investigate the possible activities of some natural products on mucin secretion from cultured airway epithelial cells. As a result of our trial, we previously reported that several natural products affected mucin secretion and/or production from airway epithelial cells [23456].
According to many reports, tussilagone (Fig. 1), a natural product derived from Tussilago farfara which has been utilized as folk remedy for controlling pulmonary inflammatory diseases in traditional oriental medicine, was reported to show anti-inflammatory effects on such an inflammatory status of human organ systems [78910]. However, to the best of our knowledge, there are no report about the potential effect of tussilagone on the gene expression and production of mucin from airway epithelial cells. Therefore, we examined the effect of tussilagone on EGF- or PMA-induced MUC5AC mucin gene expression and production from NCI-H292 cells, a human pulmonary mucoepidermoid cell line, which are frequently used for the purpose of elucidating intracellular signaling pathways involved in airway mucin production and gene expression [111213]. Also, in order to elucidate the action mechanism of tussilagone, we checked whether tussilagone affects PMA-induced NF-κB signaling pathway in NCI-H292 cells, based on the report that PMA stimulated NF-κB signaling pathway in airway epithelial cells and colon cancer cells [1415].
All the chemicals and reagents used in this experiment were purchased from Sigma (St. Louis, MO, U.S.A.) unless otherwise specified. Tussilagone (purity: 98.0%) was purchased from Avention (AV-K-006, Incheon, Korea). Anti-NF-κB p65 (sc-8008), anti-IκBα (sc-371), anti-actin (sc-8432), anti-p84 (sc-98783), anti-TRAF2 (sc-7187), anti-TRADD (sc-7868) antibodies were purchased from Santa Cruz Biotechnology (Santa Cruz, CA, U.S.A.). Anti-RIP1 antibody (#610459) was purchased from BD biosciences (San Jose, CA, USA). Phosphospecific anti-p65 (serine 536, #3036S), Phospho-specific anti-IκBα (serine 32/36, #9246), antiphospho-IKKα/β (Ser176/180, #2687) antibodies were purchased from Cell signaling Technology Inc. (Danvers, MA, U.S.A.). A Goat Anti-rabbit IgG (#401315) or Goat Anti-mouse IgG (#401215) was used as the secondary antibody (Calbiochem, Carlsbad, CA, U.S.A.).
NCI-H292 cells, a human pulmonary mucoepidermoid carcinoma cell line, were purchased from the American Type Culture Collection (ATCC, Manassas, VA, U.S.A.) and cultured in RPMI 1640 supplemented with 10% fetal bovine serum (FBS) in the presence of penicillin (100 units/ml), streptomycin (100 µg/ml) and HEPES (25 mM) at 37℃ in a humidified, 5% CO2/95% air, water-jacketed incubator. For serum deprivation, confluent cells were washed twice with phosphate-buffered saline (PBS) and recultured in RPMI 1640 with 0.2% fetal bovine serum for 24 h.
After 24 h of serum deprivation, cells were pretreated with varying concentrations of tussilagone for 30 min and treated with EGF (epidermal growth factor) (25 ng/ml) or PMA (phorbol 12-myristate 13-acetate) (10 ng/ml) for 24 h in serum-free RPMI 1640. Tussilagone was dissolved in dimethylsulfoxide and treated in culture medium (final concentrations of dimethylsulfoxide were 0.5%). The final pH values of these solutions were between 7.0 and 7.4. Culture medium and 0.5% dimethylsulfoxide did not affect mucin gene expression and production from NCI-H292 cells. After 24h, cells were lysed with buffer solution containing 20 mM Tris, 0.5% NP-40, 250 mM NaCl, 3 mM EDTA, 3 mM EGTA and protease inhibitor cocktail (Roche Diagnostics, IN, U.S.A.) and collected to measure the production of MUC5AC protein (in 24-well culture plate). The total RNA was extracted for measuring the expression of MUC5AC gene (in 6-well culture plate) by using RT-PCR. For western blot analysis, cells were treated with tussilagone for 24 h and then treated with PMA for 30 min.
MUC5AC airway mucin production was measured by ELISA. Cell lysates were prepared with PBS at 1:10 dilution, and 100 µl of each sample was incubated at 42℃ in a 96-well plate, until dry. Plates were washed three times with PBS and blocked with 2% bovine serum albumin (BSA) (fraction V) for 1 h at room temperature. Plates were again washed three times with PBS and then incubated with 100 µl of 45M1, a mouse monoclonal MUC5AC antibody (1:200) (NeoMarkers, CA, U.S.A.), which was diluted with PBS containing 0.05 % Tween 20 and dispensed into each well. After 1 h, the wells were washed three times with PBS, and 100 µl of horseradish peroxidase-goat anti-mouse IgG conjugate (1:3,000) was dispensed into each well. After 1 h, plates were washed three times with PBS. Color reaction was developed with 3,3′,5,5′-tetramethylbenzidine (TMB) peroxide solution and stopped with 1 N H2SO4. Absorbance was read at 450 nm.
Total RNA was isolated by using Easy-BLUE Extraction Kit (INTRON Biotechnology, Inc. Kyung-Ki-do, Korea) and reverse transcribed by using AccuPower RT Premix (BIONEER Corporation, Daejeon, Korea) according to the manufacturer's instructions. Two µg of total RNA was primed with 1 µg of oligo (dT) in a final volume of 50 µl (RT reaction). Two µl of RT reaction product was PCR amplified in a 25 µl by using Thermorprime Plus DNA Polymerase (ABgene, Rochester, NY, U.S.A.). Primers for MUC5AC were (forward) 5′-TGA TCA TCC AGC AGG GCT-3′ and (reverse) 5′-CCG AGC TCA GAG GAC ATA TGG G-3′. As quantitative controls, primers for Rig/S15 rRNA, which encodes a small ribosomal subunit protein, a housekeeping gene that was constitutively expressed, were used. Primers for Rig/S15 were (forward) 5′-TTC CGC AAG TTC ACC TAC C-3′ and (reverse) 5′-CGG GCC GGC CAT GCT TTA CG-3′. The PCR mixture was denatured at 94℃ for 2 min followed by 40 cycles at 94℃ for 30 s, 60℃ for 30 s and 72℃ for 45 s. After PCR, five µl of PCR products were subjected to 1% agarose gel electrophoresis and visualized with ethidium bromide under a transilluminator.
NCI-H292 cells (confluent in 150 mm culture dish) were pretreated for 24 h at 37℃ with 1, 2, 5, 10 and 20 µM of tussilagone and then stimulated with PMA (50 ng/ml) for 30 min. After the treatment of the cells with tussilagone, the cells were harvested using 3× trypsin-EDTA solution and then centrifuged in a microcentrifuge (1,200 rpm, 3 min, 4℃). The supernatant was discarded and the cell pellet was washed by suspending in PBS. The cytoplasmic and nuclear protein fractions were extracted using NE-PER® nuclear and cytoplasmic extraction reagent (Thermo-Pierce Scientific, Waltham, MA, U.S.A.) according to the manufacturer's instructions. Both extracts were stored at −20℃. Protein content in extract was determined by Bradford method.
After the treatment of the cells with tussilagone as mentioned above, media were aspirated and the cells were washed with cold PBS. The cells were collected by scraping and centrifuged at 3,000 rpm for 5 min. The supernatant was discarded. The cells were mixed with RIPA buffer (25 mM Tris-HCl pH 7.6, 150 mM NaCl, 1% NP-40, 1% sodium deoxycholate, 0.1% SDS) for 30 min with continuous agitation. The lysate was centrifuged in a microcentrifuge at 14,000 rpm for 15 min at 4℃. The supernatant was used or immediately stored at −80℃. Protein content in extract was determined by Bradford method.
Cytosolic, nuclear and whole cell extracts containing proteins (each 50 µg as protein) were subjected to 10% sodium dodecyl sulfate polyacrylamide gel electrophoresis (SDS-PAGE) and then transferred onto the polyvinylidene difluoride (PVDF) membrane. The blots were blocked using 5% skim milk and probed with appropriate primary antibody in blocking buffer overnight at 4℃. The membrane was washed with PBS and then probed with the secondary antibody conjugated with horseradish peroxidase. Immunoreactive bands were detected by an enhanced chemiluminescence kit (Pierce ECL western blotting substrate, Thermo Scientific, Waltham, MA, U.S.A.).
MUC5AC gene expression induced by EGF from NCI-H292 cells was inhibited by pretreatment with tussilagone (Fig. 2A).
Tussilagone significantly inhibited EGF-induced MUC5AC production from NCI-H292 cells. The amounts of mucin in the cells of cultures were 100±5% (control), 206±23% (25 ng/ml of EGF alone), 159±5% (EGF plus tussilagone 1 µM), 127±6% (EGF plus tussilagone 10 µM), and 102±4% (EGF plus tussilagone 100 µM), respectively (Fig. 2B). Cell viability was checked by sulforhodamine B (SRB) assay and there was no cytotoxic effect of tussilagone, at 1, 10 and 100 µM (data not shown).
MUC5AC gene expression induced by PMA from NCI-H292 cells was inhibited by pretreatment with tussilagone (Fig. 3A).
Tussilagone significantly inhibited PMA-induced MUC5AC production from NCI-H292 cells. The amounts of mucin in the cells of cultures were 100±10% (control), 271±4% (10 ng/ml of PMA alone), 199±6% (PMA plus tussilagone 1 µM), 146±2% (PMA plus tussilagone 10 µM), and 139±7% (PMA plus tussilagone 100 µM), respectively (Fig. 3B).
As shown in Fig. 4, nuclear translocation of NF-κB p65 by PMA was inhibited by pretreatment with tussilagone, dose-dependently. In the nuclear fraction of the cells treated with PMA only, there was an increase in nuclear translocation of p65 gradually and reached optimal level at 30 min. However, in the cells treated with tussilagone plus PMA, the level of p65 was gradually decreased as compared to the cells treated with PMA only. Transcriptional activity of p65 largely depends on its phosphorylation. PMA-induced phosphorylation of p65 was reached optimal level at 30 min. However, tussilagone suppressed the phosphorylation of p65.
As shown in Fig. 5, PMA increased the phosphorylation of IκBα. Preincubation of NCI-H292 cells with tussilagone prior to PMA treatment inhibited the phosphorylation of IκBα. PMA also induced degradation of IκBα. However, IκBα degradation was inhibited by pretreatment of tussilagone. Activation of IKK, which phosphorylates IκBα, depends on its phosphorylation. Therefore, we investigated whether tussilagone inhibits the PMA-induced phosphorylation of IKKα/β. PMA activated the IKKα/β, although tussilagone suppressed its activation by regulating the serine 176/180 phosphorylation of IKKα/β.
Among the twenty one or more MUC genes coding human mucins reported up to now, MUC5AC was mainly expressed in goblet cells in the airway surface epithelium [116]. Takeyama et al. reported that epidermal growth factor (EGF) regulated MUC5AC gene expression in the lung. According to their reports, MUC5AC mRNA expression was increased after ligand binding to the EGF receptor and activation of the mitogen-activated protein kinase (MAPK) cascade [1217]. Phorbol 12-myristate 13-acetate (PMA) was reported to stimulate the endogenous activator of protein kinase C (PKC), diacylglycerol (DAG) [18] and to be an inflammatory stimulant that can control a gene transcription [19], cell growth and differentiation [20]. PMA also can induce MUC5AC gene expression in NCI-H292 cells [21] as well as in various lung epithelial cells [22].
On the other hand, as mentioned in Introduction, tussilagone, a natural product derived from Tussilago farfara which has been utilized as folk remedy for controlling pulmonary inflammatory diseases in traditional oriental medicine, was reported to show anti-inflammatory effects on such an inflammatory status of human organ systems [78910]. Based upon these reports, we investigated whether tussilagone significantly affects the production and gene expression of airway mucin induced by EGF or PMA. As shown in results, tussilagone suppressed the expression of MUC5AC mucin gene induced by EGF or PMA (Figs. 2A and 3A). At the same time, tussilagone inhibited the production of MUC5AC mucin protein induced by the same inducers (Figs. 2B and 3B). These results suggest that tussilagone can regulate the gene expression and production of mucin induced by EGF or PMA, by acting on airway epithelial cells.
Next, in order to elucidate a potential mechanism of action of tussilagone, it was investigated whether tussilagone affects the NF-κB signaling in NCI-H292 cells. PMA was reported to stimulate NF-κB signaling pathway in airway epithelial cells and colon cancer cells [1415]. Also, PMA induced the gene expression levels of proinflammatory cytokines including TNF-α [16]. NF-κB is a heterodimer composed of p65, p50 and IκBα subunits present in the cytoplasm as an inactive state. In response to various stimuli, the IκBα subunit is phosphorylated and degraded, thereby facilitating the translocation of p50–p65 heterodimer to the nucleus. The p50–p65 heterodimer acts as a transcription factor regulating the expression of numerous genes including MUC5AC [23]. The transcription factor NF-κB has been a potential therapeutic target and NF-κB and related signaling proteins may be regulated by anti-inflammatory natural products including tussilagone.
To determine the effect of tussilagone on NF-κB activation followed by PMA treatment, we examined whether tussilagone inhibits PMA-induced nuclear translocation of NF-κB p65. As shown in Fig. 4, nuclear translocation of NF-κB p65 by PMA was inhibited by pretreatment with tussilagone, dose-dependently. In the nuclear fraction of the PMA only-treated cells, there was an increase in nuclear translocation of p65 gradually and reached optimal level at 30 min. However, in the cells treated with tussilagone plus PMA, the level of p65 was gradually decreased as compared to the PMA only-treated cells. Next, it was reported that transcriptional activity of p65 depends on its phosphorylation [23]. For this reason, we examined the effect of apigenin on PMA-induced phosphorylation of p65. As shown in Fig. 4, PMA-induced phosphorylation of p65 was reached optimal level at 30 min. However, tussilagone inhibited the phosphorylation of p65.
NF-κB activation involves the phosphorylation of IκBα by IKKs, resulting in IκBα degradation. As a result, NF-κB is released and translocates freely into the nucleus. Therefore, effect of tussilagone on the phosphorylation and degradation of IκBα was examined. Tussilagone appears to affect the phosphorylation and degradation of IκBα which is required for NF-κB dimerization and maximal activation of transcription. As shown in Fig. 5, PMA increased the phosphorylation of IκBa. However, preincubation of NCI-H292 cells with tussilagone prior to PMA exposure suppressed the phosphorylation of IκBα. IκBα degradation is required for the activation of NF-κB. Consequently, we checked whether tussilagone inhibits PMA-induced NF-κB activation by inhibition of IκBα degradation As shown in Fig. 5, PMA showed the induction of IκBα degradation. However, preincubation of NCI-H292 cells with tussilagone prior to PMA exposure suppressed the degradation of IκBα.
For most agents that activate NF-κB through a common pathway based on phosphorylation, proteaseome mediated the degradation of IκB. The key regulatory step in this pathway involves the activation of IκB kinase (IKK) complex. Activation of IKK depends on phosphorylation. Therefore, we investigated whether tussilagone inhibits the PMA-induced activity of IKKα/β. PMA activated the IKKα/β, although tussilagone suppressed its activation by regulating the serine 176/180 phosphorylation of IKKα/β (Fig. 5).
Taken together, the inhibitory action of tussilagone on airway mucin production and gene expression might be mediated by, at least in part, affecting PMA-induced degradation of IκBα and nuclear translocation of NF-κB p65. We suggest it is valuable to find the natural products that have specific inhibitory effects on mucin production and gene expression-in view of both basic and clinical sciences-and the result from this study suggests a possibility of using tussilagone as a new efficacious mucoregulator for diverse inflammatory pulmonary diseases, although further studies are essentially required.
Figures and Tables
Fig. 2
Effect of tussilagone on EGF-induced MUC5AC mucin production and gene expression from NCI-H292 cells.
NCI-H292 cells were pretreated with varying concentrations of tussilagone for 30 min and then stimulated with EGF (25 ng/ml) for 24 h. Cell lysates were collected for measurement of MUC5AC mucin production by ELISA. Each bar represents a mean±S.E.M. of 3 culture wells in comparison with that of control set at 100% (B). MUC5AC gene expression was measured by RT-PCR (A). Three independent experiments were performed and the representative data were shown. *significantly different from control (p<0.05). †significantly different from EGF alone (p<0.05). cont: control, T: tussilagone, concentration unit is µM.
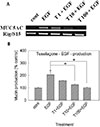
Fig. 3
Effect of tussilagone on PMA-induced MUC5AC mucin production and gene expression from NCI-H292 cells.
NCI-H292 cells were pretreated with varying concentrations of tussilagone for 30 min and then stimulated with PMA (10 ng/ml) for 24 h. Cell lysates were collected for measurement of MUC5AC mucin production by ELISA. Each bar represents a mean±S.E.M. of 3 culture wells in comparison with that of control set at 100% (B). MUC5AC gene expression was measured by RT-PCR (A). Three independent experiments were performed and the representative data were shown. *significantly different from control (p<0.05). †significantly different from PMA alone (p<0.05). cont: control, T: tussilagone, concentration unit is µM.
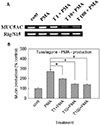
Fig. 4
Effect of tussilagone on PMA-induced phosphorylation and translocation of NF-kB p65 in NCI-H292 cells.
NCI-H292 cells were incubated with varying concentrations of tussilagone for 24 h and treated with 50 ng/ml PMA for 30 min. Nuclear protein extracts were prepared and subjected to western blot analysis using antibodies against p65 and phospho-p65. The result shown is a representative of three independent experiments. As a loading control, p84 levels were analyzed. cont: control, T: tussilagone, concentration unit is µM.
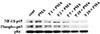
Fig. 5
Effect of tussilagone on PMA-induced phosphorylation of IκBα, degradation of IκBα and phosphorylation of IKKα/β in NCI-H292 cells.
NCI-H292 cells were incubated with varying concentrations of tussilagone for 24 h and treated with 50 ng/ml PMA for 30 min. Cytoplasmic extracts were fractionated and then subjected to western blot analysis using phospho-specific IκBα (Ser 32/36) antibody or antibody against anti-IκBα. Whole cell lysates (100 µg) were prepared and then subjected to western blot analysis using phospho-specific IKKα/β (Ser 176/180) antibody. The results shown are the representative of three independent experiments. Equal protein loading was evaluated by β-actin levels. cont: control, T: tussilagone, concentration unit is µM.
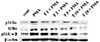
ACKNOWLEDGEMENTS
This research was supported by NRF-2014R1A6A1029617 and NRF-2017R1C1B1005126, Basic Science Research Program through the National Research Foundation of Korea (NRF) funded by the Ministry of Education.
Notes
References
2. Lee HJ, Park JS, Yoon YP, Shin YJ, Lee SK, Kim YS, Hong JH, Son KH, Lee CJ. Dioscin and methylprotodioscin isolated from the root of asparagus cochinchinensis suppressed the gene expression and production of airway MUC5AC mucin induced by phorbol ester and growth factor. Phytomedicine. 2015; 22:568–572.


3. Kim EJ, Yoon YP, Woo KW, Kim JH, Min SY, Lee HJ, Lee SK, Hong JH, Lee KR, Lee CJ. Verticine, ebeiedine and suchengbeisine isolated from the bulbs of Fritillaria thunbergii Miq. inhibited the gene expression and production of MUC5AC mucin from human airway epithelial cells. Phytomedicine. 2016; 23:95–104.


4. Lee HJ, Ryu J, Park SH, Seo EK, Han AR, Lee SK, Kim YS, Hong JH, Seok JH, Lee CJ. Suppressive effects of coixol, glyceryl trilinoleate and natural products derived from Coix Lachryma-Jobi var. Mayuen on gene expression, production and secretion of airway MUC5AC mucin. Arch Pharm Res. 2015; 38:620–627.


5. Ryu J, Lee HJ, Park SH, Kim J, Lee D, Lee SK, Kim YS, Hong JH, Seok JH, Lee CJ. Effects of the root of Platycodon grandiflorum on airway mucin hypersecretion in vivo and platycodin D(3) and deapi-platycodin on production and secretion of airway mucin in vitro. Phytomedicine. 2014; 21:529–533.


6. Park SH, Lee HJ, Ryu J, Son KH, Kwon SY, Lee SK, Kim YS, Hong JH, Seok JH, Lee CJ. Effects of ophiopogonin D and spicatoside A derived from Liriope tuber on secretion and production of mucin from airway epithelial cells. Phytomedicine. 2014; 21:172–176.


7. Lee J, Kang U, Seo EK, Kim YS. Heme oxygenase-1-mediated antiinflammatory effects of tussilagonone on macrophages and 12-O-tetradecanoylphorbol-13-acetate-induced skin inflammation in mice. Int Immunopharmacol. 2016; 34:155–164.
8. Hwangbo C, Lee HS, Park J, Choe J, Lee JH. The anti-inflammatory effect of tussilagone, from Tussilago farfara, is mediated by the induction of heme oxygenase-1 in murine macrophages. Int Immunopharmacol. 2009; 9:1578–1584.


9. Lim HJ, Lee HS, Ryu JH. Suppression of inducible nitric oxide synthase and cyclooxygenase-2 expression by tussilagone from Farfarae flos in BV-2 microglial cells. Arch Pharm Res. 2008; 31:645–652.


10. Kim YK, Yeo MG, Oh BK, Kim HY, Yang HJ, Cho SS, Gil M, Lee KJ. Tussilagone inhibits the inflammatory response and improves survival in CLP-induced septic mice. Int J Mol Sci. 2017; 18.


11. Li JD, Dohrman AF, Gallup M, Miyata S, Gum JR, Kim YS, Nadel JA, Prince A, Basbaum CB. Transcriptional activation of mucin by Pseudomonas aeruginosa lipopolysaccharide in the pathogenesis of cystic fibrosis lung disease. Proc Natl Acad Sci U S A. 1997; 94:967–972.


12. Takeyama K, Dabbagh K, Lee HM, Agustí C, Lausier JA, Ueki IF, Grattan KM, Nadel JA. Epidermal growth factor system regulates mucin production in airways. Proc Natl Acad Sci U S A. 1999; 96:3081–3086.


13. Shao MX, Ueki IF, Nadel JA. Tumor necrosis factor alphaconverting enzyme mediates MUC5AC mucin expression in cultured human airway epithelial cells. Proc Natl Acad Sci U S A. 2003; 100:11618–11623.
14. Ishinaga H, Takeuchi K, Kishioka C, Suzuki S, Basbaum C, Majima Y. Pranlukast inhibits NF-kappaB activation and MUC2 gene expression in cultured human epithelial cells. Pharmacology. 2005; 73:89–96.
15. Laos S, Baeckström D, Hansson GC. Inhibition of NF-kappaB activation and chemokine expression by the leukocyte glycoprotein, CD43, in colon cancer cells. Int J Oncol. 2006; 28:695–704.
17. Takeyama K, Dabbagh K, Jeong Shim J, Dao-Pick T, Ueki IF, Nadel JA. Oxidative stress causes mucin synthesis via transactivation of epidermal growth factor receptor: role of neutrophils. J Immunol. 2000; 164:1546–1552.


18. Hong DH, Petrovics G, Anderson WB, Forstner J, Forstner G. Induction of mucin gene expression in human colonic cell lines by PMA is dependent on PKC-epsilon. Am J Physiol. 1999; 277:G1041–G1047.
19. Hewson CA, Edbrooke MR, Johnston SL. PMA induces the MUC5AC respiratory mucin in human bronchial epithelial cells, via PKC, EGF/TGF-alpha, Ras/Raf, MEK, REK and Sp1-dependent mechanisms. J Mol Biol. 2004; 344:683–695.
20. Park SJ, Kang SY, Kim NS, Kim HM. Phosphatidylinositol 3-kinase regulates PMA-induced differentiation and superoxide production in HL-60 cells. Immunopharmacol Immunotoxicol. 2002; 24:211–226.


21. Kim KD, Lee HJ, Lim SP, Sikder A, Lee SY, Lee CJ. Silibinin regulates gene expression, production and secretion of mucin from cultured airway epithelial cells. Phytother Res. 2012; 26:1301–1307.


22. Wu DYC, Wu R, Reddy SP, Lee YC, Chang MM. Distinctive epidermal growth factor receptor/extracellular regulated kinase-independent and -dependent signaling pathways in the induction of airway mucin 5B and mucin 5AC expression by phorbol 12-myristate 13-acetate. Am J Pathol. 2007; 170:20–32.
23. Li Q, Verma IM. NF-kappaB regulation in the immune system. Nat Rev Immunol. 2002; 2:725–734.